ABSTRACT
Morphological study on spermatids and spermatozoa have long been performed regarding various changes of cell organelles during spermiogenesis as a potential phylogenetic inference. Based on the fact that the number of germ cells per cyst increases according to a geometric series, knowing the exact number of germ cells in a certain stage may lead to the total number of sperms produced per cyst. In spiders, however, the entire process takes place in a cyst represented by a spermatogonium, producing sperms in spherical shape. It is very difficult to count the exact number of germ cells produced per cyst through a 2D image analysis. Therefore, we applied a 3D image of testicular cyst of an orb-weaving spider to visualize the exact number of germ cells produced from a cyst. In this study, 2D images obtained from serially sectioned micrographs were scanned precisely and reconstructed using a 3D-rendering technique. Finally, this research reveals that the exact number of spermatozoa produced each cyst in Larinioides cornutus appeared to be 128 (27), which indicates that a single spermatogonium undergoes five mitotic divisions and two maturing divisions (meiosis) to produce final spermatozoa.
Introduction
Studies on the ultrastructure of spermatozoa in spiders revealed an astonishing diversity of this cell type (Alberti Citation1990, Citation2000; Michalik et al. Citation2004a, Citation2004b; Costa-Ayub & Faraco Citation2007). The study of sperm structure and development is providing new data relevant for phylogenetic inference within higher categories (Dallai et al. Citation2003a, Citation2003b; Scheltinga et al. Citation2003; Marotta & Ruhberb Citation2004; Meisner et al. Citation2005). However, data on spider sperm ultrastructure and development continue to be fragmentary in most groups (Michalik & Huber Citation2006), and most of the morphological studies are focused on spermiogenetic events (Alberti & Coyle Citation1991; Klann et al. Citation2005; Costa-Ayub & Faraco Citation2007).
In insects, germ cells get surrounded by a membrane formed by epithelial cells, and the divisions occur synchronously within each of the envelope called cysts or bundles. Since the entire process of spermatogenesis occurs within a cyst, the number of sperm cells produced in a cyst of given species may be uniformly maintained with almost no cell degeneration of sperms until the late stage of spermatid unless treated (Bowen Citation1925; Owusu-Daaku et al. Citation1993).
Therefore, the number of germ cells per ordinary cyst increases through a geometric series, 2n, and counting the exact number of spermatocytes becomes possible. When the exact number is known, the total number of sperm cells produced per cyst can be calculated accordingly (Virkki Citation1969). Understanding the exact number of sperm cells per cyst in spiders may be potentially useful in resolving various questions raised in many fields of spider biology, such as behavioral biology and ecology.
It is very difficult to acquire such data since this area of research is still limited. In insects, the exact number of sperm cells can be easily visualized because of their structural simplicity. Most insects have elongated spermatids as well as cysts, which makes it possible to count the exact number of cells in a single microscopic image of a transverse section. However, it is difficult to analyze the exact number of cells in a cyst of spiders, since each cyst adopts a spherical shape encapsulated in a sheath and their elongated spermatids are heavily coiled (Michalik et al. Citation2004b). Single image may be insufficient to gather necessary information from such cleistospermy, but on the other hand, 3D images of the entire cyst may be rendered by stacking 2D serial images.
Reconstruction of 3D image is not a new technique, but such techniques were mainly applied in the field of medical or neurosciences, which have been utilizing the advantages of 3D imaging with respective apparatuses such as confocal microscope and MRI (Sramek Citation2006). Up to now, this area of research in spiders is still limited and there are only few studies on the field revealing the exact number of sperms per cyst and the number of cell divisions during the spermatogenetic process (Kim et al. Citation2014).
Here, we examined serial sectioned images of testicular cyst in the furrow orb-weaver, Larinioides cornutus with aid of light and transmission electron microscopes by means of the 3D volume-rendering technique to reveal the fine structural reconstruction of the testicular cysts and to determine the exact number of germ cells per cyst during spermatogenesis.
Materials and methods
Male individuals of L. cornutus were collected at various sites around Dankook University (Cheonan, Korea). Collected samples were kept in a wooden cage (30 × 30 × 10 cm) where they were fed with Tenebrio molitor larvae daily, and maintained under ambient conditions with natural lighting. Sexually mature adults were selected as specimens to make microscopic sections of testicular cyst. The opisthosoma of the specimens was dissected in spider ringer solution (128 mM NaCl, 18 mM CaCl2, 1.3 mM KCl, and 2.3 mM NaHCO3, pH 7.4)
in a way to remove exoskeleton and isolate testis. (Moon & Tillinghast Citation2013; Park & Moon Citation2014)
For light microscopic observation, the opisthosomae were fixed in Bouin’s fixative solution for 24 hours, and dehydrated using ascending concentration of graded series of ethanol. After dehydration, ethanol was substituted to xylene for clearing. Then specimens were embedded in Paraplast medium (Polysciences Inc., Warrington, USA). Sectioning was performed with a Leica rotary microtome (Leica RM 2153, Berlin, Germany). The sections were stained with hematoxylin & eosin. Microscopic images were captured using Eclipse 80i research microscope (Nikon, Tokyo, Japan) coupled with Motic digital imaging system (Motic Instruments Inc., Richmond, Canada).
For transmission electron microscopy, the isolated testes were fixed in a cold solution of 2.5% paraformaldehyde – 2% glutaraldehyde (4°C, 0.1 M phosphate buffer, pH 7.4) for 2 hours. The tissues were then washed in 0.1 M phosphate buffer (4°C, pH 7.4) for 45 minutes, postfixed in 1% osmium tetroxide for 2 hours, and washed again in 0.1 M phosphate buffer for another 45 minutes. The specimens were dehydrated through a graded series of ethanol (70–100%; 15 minutes each), immersed in propylene oxide for 1 hour, and finally embedded in epoxy resin (EM Bed) (Polysciences Inc., Warrington, USA). The embedded blocks were then sectioned with ultramicrotome (Ultracut R, Leica, Berlin, Germany) with the thickness of 300 nm, to confirm the condition of samples, then again with the thickness of approximately 60 nm for thin sections. Thin sections were double stained with uranyl acetate and lead citrate on 300 mesh grids. Observation was done using transmission electron microscope (Tencai G2 S-Twin, FEI, Oregon, USA) at an accelerating voltage of 80 kV.
Captured images from photomicroscope were analyzed with the Motic Images Advanced program (Motic Instruments Inc., Richmond, Canada) and Image J program (National Institutes of Health, USA) to collect numerical data. For 3D volume rendering, images were edited with Adobe Photoshop CS3 for better adaptability to the rendering software. First, the images were converted into 8-bit grayscale images. Then if necessary, color inversion was applied on selected images to increase program sensitivity.
The section images were then loaded into the program Amira 5.3.2 (Visage Imaging Inc., Andover, MA, USA) using the import functions Channel 1 for 8-bit grayscale with dimensions calibrated in a way that one model unit corresponded to 1 µm, and automatically aligned using the least-squares function of the AlighSlices tool. Automatically aligned image stacks were verified by eye and corrected manually if necessary. After alignment, the image stacks were saved in amiramesh (.am) format.
Aligned images were labeled with the Labelfield tool, to separately designate the areas of the cyst and spermatocytes. To avoid interference between two channels, both cyst and spermatocytes were given separate labels, and to minimize artifacts in volume rendering, Interpolation was applied in every second and third images. In case of both cyst and spermatocytes, areas within the membrane were selected using the Brush tool. When labeling was done, the SmoothLabels tool and RemoveHalls tool for 3D image rendering in the Segmentation menu were applied to reduce uneven artifacts in the final visualization.
The 3D surface views of the reconstructed testicular cyst and spermatocytes were generated with the module SurfaceGen and were visualized with the SurfaceView tool. To locate the actual region of the 3D model in the testis, the whole section images were blended into a single 3D image as well.
Results
The paired testes occupy the most ventral portion in the center of the abdomen. These white tubular organs are located between and slightly behind the book lungs, merging into a common duct – vas deferens. The testis had a shape of a thick convoluted tube. Even though the abdomen is mostly filled with connective tissues and various silk glands, L. cornutus showed no intersections between testis and other. Each testis, which has fairly thick bounding epithelium, with basal lamina and a few muscle cells, continues into the vas deferens ().
Upon various micrographs of the testis, all typical stages of spermatogenesis were visible – starting with spherical germ cells, and the development takes place all over the testis in a way that entire processes occur in a cyst, surrounded by thin somatic membrane. Cysts at the periphery of testis mostly contained spermatogonia, while the ones in the latter stages of development were observed inward. Germ cells observed in a cyst were all at the same developmental stage, until the cyst bursts out to release mature sperm balls into the lumen, with a secretion sheath presenting cleistospermia. The produced coiled sperms are kept in the testis until ejaculation ( and ).
Figure 1. Different stages of spermatogenesis observed in the testis of L. cornutus. Spermatogenesis of L. cornutus consists of four distinctive stages: spermatogonium, spermatocyte, spermatid, and spermatozoon. SG: silk gland; Ts: Testis. White arrowhead indicates spermatogonia; Black arrowhead indicates spermatocytes; Black arrow indicates spermatids undergoing spermiogenesis. White arrow indicates spermatozoon. Magnifications are as follow: (a) ×40; (b) ×100; (c) ×200; (d) ×400.
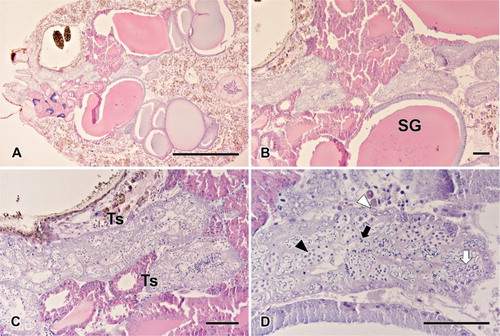
Figure 2. Transverse section of the testis in L. cornutus. Spermatogenesis in spider testis occurs along inward direction. Spermatogonium stays along the testicular membrane, maintaining the cell line. As development progresses, spermatocytes moves inward to produce differentiated cells. SG: spermatogonium; SCp: primary spermatocyte; SCs: secondary spermatocyte; ST: spermatid. Magnifications are as follow: (a, b, e) ×2800; (c, d) ×3900; (f): ×5800.
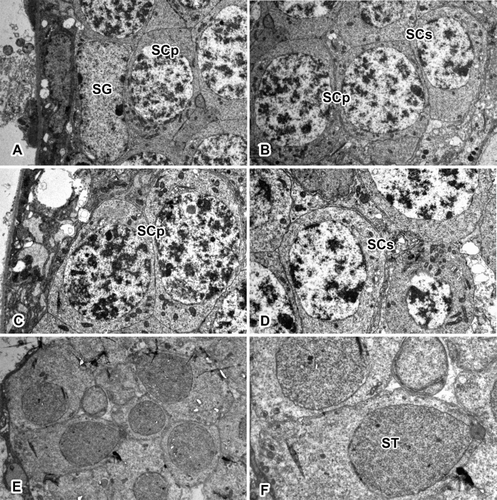
In the microscopic sections, simple squamous epithelial cells were shown to form the basal layer of the testicular membrane, with cysts of various sizes and sperm balls in the tissue fluid-filled lumen. In a single transverse section of testis, about 45–50 cysts were observed all at different stages of development, with the spermatogonia at the periphery and the cells gradually increasing in maturity toward the lumen. In most cases, the cysts stay near other cysts that are in the same developmental stages.
The formation of sperm begins at the periphery of testis, at which primary spermatogonia are forming a line along the testicular membrane. Spermatogonia are large cells with a diameter of 13.06 ± 1.57 μm (mean ± SD), possessing a clear cytoplasm with oval nucleus occupying most of the space (). The chromatic material was dispersed all over the nucleus. Their sizes varied according to the number of divisions that have occurred; however, usually the nucleus is the largest after the last division.
Table 1. Numerical data about germ cell morphology.
When the spermatogonia initiate the meiotic prophase, they transform into primary spermatocytes, which are slightly larger cells (15.38 ± 1.41 μm) with a large nucleus that occupies most of the cytoplasm with granular chromatin. Although cysts at this stage are usually located beneath spermatogonia, some may be found beside the spermatogonia as well. These cells ultimately give rise to the secondary spermatocytes, which are characterized by nuclear reduction (7.25 ± 0.79 μm) and intense cell division (). Chromatic material is well condensed, showing a lot darker staining compared to the primary spermatocytes.
Upon spermiogenesis, the cells in the cyst no longer maintain their spherical shapes, but rather have elongated cytoplasm and become spermatids (2.42 ± 0.26 μm) (). The nuclei of spermatids are filled with a great condensation of chromatin. The cystic membrane ruptures at the end of spermiogenesis. When spermiogenesis is over, the germ cells differentiate into spermatozoon without additional cell division. One of the unique characteristics of spermatozoa in the testis is that they do not have an intact testicular cyst. Germ cells are in the shape of a sperm-ball, coated with a separate single protein layer. They are kept in the lumen of the testis and vas deferens until ejaculation takes place.
To acquire additional numerical data, 3D image was rendered using the serial microsections. The images used for this analysis contained about less than 20 intact cysts, filled with germ cells at various developmental stages. Although cysts with spermatids were also found, cystic membranes in the late spermatid stage was often found to be ruptured. In the lumen, produced spermatozoa were in the form of the typical ring-shaped sperm-ball. In order to obtain a full 3D cyst, over 100 images were taken. The images were then roughly aligned to locate intact cyst samples, and only manually chosen images were used in rendering, since an accurate 3D image can only be rendered with the cysts with complete membranes. One whole cyst corresponded to approximately 12–15 microscopic sections, with an average thickness of about 70 μm. Selected images were then edited to produce a set of trimmed grayscale images, which was performed to increase the sensitivity of the program ().
Figure 3. Image editing steps prior to rendering. (a) Light microscopic sectioned images with basic H&E staining. (b) Images converted into grayscale mode due to sensitivity of the program. (c) Unwanted portions may be trimmed to increase accuracy. (d) Color inversion completed in order to eliminate background in the rendering program.
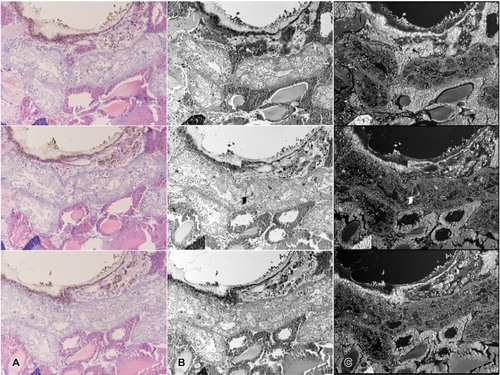
Edited images were aligned along the right axes, then the portion of spermatocytes and testicular cysts were labeled accordingly in the aligned image stack (). Afterwards, the labeled areas were rendered separately, then reconstructed into 3D images to visualize the cyst and spermatocytes ( and ).
Figure 4. Image loading steps using the Amira program. (a) Set of images loaded in Amira. When the axes are aligned, serial images can be viewed through the Orthoslices tool. (b) Image labeled using the Segmentation editor. Yellow colored area indicates a labeled cyst. Spermatocytes were labeled separately so as not to interrupt the channels. (c–d) Images before applying the Alignslices tool (c). Arrowhead indicates unaligned portion of the image. Aligned images after applying the Alignslices tool (d).
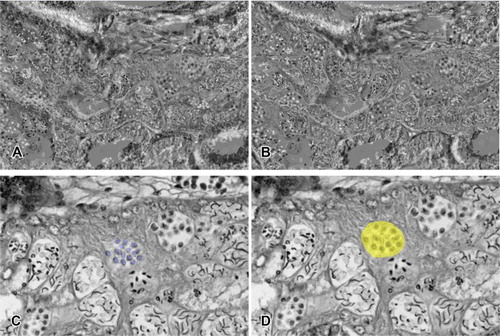
Figure 5. Testicular cyst rendered into 3D image. (a) Images were taken while rotating every 30 degrees on the XY axis. (b) Images were taken while rotating every 30 degrees on the YZ axis. (c) Images were taken while rotating every 30 degrees on the XZ axis.
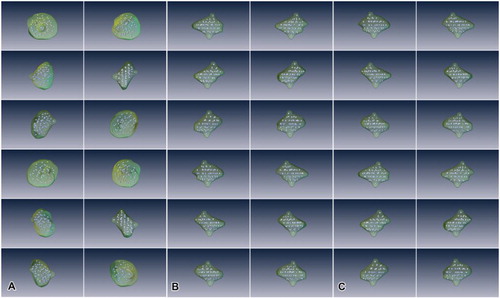
Figure 6. Rendered image viewed in different mode and angle. (a) Rendered cyst and spermatocytes in comparison with the images used. TO indicates transverse orthoslice, SO indicates sagittal orthoslice, CO indicates coronal orthoslice. SO and CO are produced after image alignment. (b) Lateral view of rendered testicular cyst with testis along the XZ axis. (c) Lateral view of rendered testicular cyst with testis along the XY axis. (d) Lateral view of rendered testicular cyst with testis along the YZ axis. *Rendered red portion indicates comparative volume of testis used in the reconstruction.
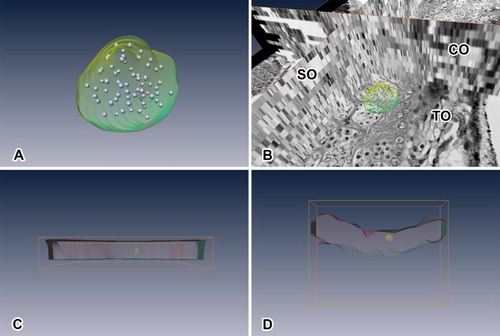
Based on the rendered model of the testicular cyst, the number of secondary spermatocytes per cyst was 64 (26) ( and ). Thus, single spermatogonia undergoes six cell divisions to produce the secondary spermatocytes, and seven cell divisions to produce spermatozoa. Since the secondary spermatocyte undergoes one more cell division to produce spermatid, which becomes spermatozoon without any further division, the final number of sperms per cyst could be calculated as 128 (27).
Discussion
The features of male reproductive system in arachnids have drawn a great interest in many biologists for centuries. As a result, a large amount of data are accumulated in the field, providing a wealth of information, particularly on the genitalia and germ-cell development (Burger et al. Citation2006; Michalik & Ramirez Citation2014). However, when it comes to germ-cell development, most of the studies are focused on the spermiogenesis, covering acrosome formation (Michalik & Huber Citation2006; Costa-Ayub & Faraco Citation2007), mitochondria and filament arrangement in flagellum (Baccetti et al. Citation1970; Phillips Citation1974; Zizzari et al. Citation2008) as a potential phylogenetic inference.
One of the interesting characteristics of spider sperm is that the sperm has a coiled tail and spherical shape upon the completion of development (Foelix Citation2011). Because of the unique morphological characteristics of spider sperm, there have been limits in analyzing spermatogenetic processes solely based on 2D image analysis. By this reason, secondary spermatocytes of L. cornutus were selected in this study to render 3D image and estimate the exact number of cells with the most stable properties for 3D volume rendering procedures (Kim et al. Citation2014).
Using 3D image analysis in certain field of biology has lately become one of the common approaches, using tomographic devices such as CT and MRI. However, small organisms with difficulties in using such devices still have to undergo histological processes to create specific images. There are already several 3D approach models in bees, wasps, locusts and so on, rendering brain and nerve systemic models (Rohlfing et al. Citation2001; Smid et al. Citation2003; Kurylas et al. Citation2008; el Jundi et al. Citation2010; Rybak et al. Citation2010).
Most of the known rendering models are insects, and even the species with greatest amount of spermatogenetic knowledge accumulated are insects as well. The spermatogenesis in insects, germ cells undergoes developmental processes in the form of a bundle. They are interconnected, and the mature sperms become elongated and filamentous, with average length of 300 µm (Gullan & Cranston Citation2010). The bundles containing sperms elongate as well upon maturation of sperms, and this makes the sperm cells lie parallel to each other. This characteristic provides the exact number of sperms per bundle and such data have been well established in a dataset (Phillips Citation1970).
On the other hand, there has not been a comprehensive dataset on spider sperm numbers. According to the present study, the number of secondary spermatocytes of testicular cyst in L. cornutus was determined to be 64 (26) cells. This means each cyst ultimately produces 128 (27) spermatozoa, which presumably arose from five synchronous mitotic divisions and an additional two meiotic divisions. Our finding of exactly 128 cells per cyst without any exceptions suggests that there is almost no loss of germ cells during spermatogenetic development in spiders.
As the number of divisions in a cyst appeared to be constant for a given species, the number of spermatids per cyst may be a characteristic trait for each species and can be expressed as 2n (Virkki Citation1969; Phillips Citation1970). It is known that the number of sperm cells produced per cyst may lead to a better understanding of correlation between sperm number and sperm competition (Gomez Montono et al. Citation2011). Since sperm competition is a powerful selective force that has shaped male reproductive behavior, physiology, reproductive tract morphology, and gamete phenotype (Birkhead & Meller Citation1998; Simmons Citation2001; Birkhead et al. Citation2009), any factors that may affect sperm competition could be considered to have its own evolutionary drive.
The results of this study together with comparisons to our previous studies of the sperm number per cyst (Kim et al. Citation2014, Citation2015) contribute novel biological and evolutionary knowledge of these sperm number and their competition. This study shows that the furrow orb-web spider L. cornutus (Araneae, Araneidae) produces 128 spermatozoa from one testicular cyst. However, previous studies have shown that both of the lycosid spider Pardosa laura (Kim et al. Citation2014) and theridiid spider Achaearanea tepidariorum (Kim et al. Citation2015) were composed of 64 sperms per cyst.
Our failure to find a direct association between the exact number of spider sperms per cyst and their phylogeny indicates that the direct comparison among the taxa of spiders cannot be explained mainly because of shortage of accumulated data in this area of research. Nevertheless, it seems that the number of sperms is interconnected with several traits during its lifespan including the difference in habitats and evolutionary consequences (Gomez Montono et al. Citation2011; Tourmente et al. Citation2011). Virkki (Citation1969) suggested in insects that the more advanced orders and specialized groups are characterized by smaller number of sperms than the archaic or the less specialized groups. However, our findings obtained through serial researches among the familial levels of spiders do not seem to coincide with Virkki’s proposal since a wandering lycosid spiders P. laura (Kim et al. Citation2014) exhibited smaller number per cyst compared to a specialized group of web-building araneid spiders L. cornutus (Kim et al. Citation2015).
It has been reported that some male species of web-building spiders show a variety of behavioral and morphological features including a male sacrifice behavior (Foellmer & Fairbairn Citation2004) and permanent sperm depletion during copulation (Cristenson Citation1989). While the gonial and meiotic divisions occur in pupal or nymphal stages of the life cycle, the imaginal testis only contains spermatids and spermatozoa (Michalik & Rittschof Citation2011). However, various stages of spermatogenetic cells were observed simultaneously in the testis of the adult males of L. cornutus. This indicates that active spermatogenetic development still occurs in adult males in spite of their completion of the maturation molt. Similarly, it has also been reported that meiotic and even gonial divisions also occur in the imaginal testis of certain species of insects with prolonged longevity including beetles and dragonflies (Phillips Citation1970).
Disclosure statement
No potential conflict of interest was reported by the authors.
Additional information
Funding
References
- Alberti G. 1990. Comparative spermatology of Araneae. Acta Zool Fennica. 190:17–34.
- Aberti G. 2000. Chelicerata. In: Jamieson BGM, Adiyodi KG, Adiyodi RG, editors. Reproductive biology of the invertebrates. New York (NY): Oxford & IBH/Wiley; p. 311–388.
- Alberti G, Coyle FA. 1991. Ultrastructure of the primary male genital system, spermatozoa, and spermiogenesis of Hypochilus pococki (Araneae, Hypochilidae). J Arachnol. 19:136–149.
- Baccetti B, Dallai R, Rosati F. 1970. The spermatozoon of Arthropoda. 8. The 9 + 3 flagellum of spider sperm cells. J Cell Biol. 44:681–682. doi: 10.1083/jcb.44.3.681
- Birkhead TR, Hosken DJ, Pitnick S. 2009. Sperm biology: an evolutionary perspective. Burlinton (MA): Academic Press.
- Birkhead TR, Meller AP. 1998. Sperm competition and sexual selection. San Diego (CA): Academic Press.
- Bowen RH. 1925. Studies on insect spermatogenesis. V. On the formation of the sperm in Lepidoptera. Quart J Micro Sci. 66:595–611.
- Burger M, Michalk P, Graber W, Jacob A, Nentwig W, Kropf C. 2006. Complex genital system of a haplogyne spider (Arachnida, Araneae, Tetrablemmidae) indicates internal fertilization and full female control over transferred sperm. J Morphol. 267:166–186. doi: 10.1002/jmor.10394
- Christenson TE. 1989. Sperm depletion in the orb-weaving spider Nephila clavipes (Araneae, Araneidae). J Arachnol. 17:115–118.
- Costa-Ayub CLS, Faraco CD. 2007. Ultrastructural aspects of spermiogenesis and synspermia in the brown spider Loxosceles intermedia (Araneae: Sicariidae). Arthropod Struct Dev. 36:41–51. doi: 10.1016/j.asd.2006.07.004
- Dallai R, Frati F, Luppetti P, Adis J. 2003a. Sperm ultrastructure of Mantophasma zephyra (Insecta, Mantophasmatodea). Zoomorphology. 122:211–220. doi: 10.1007/s00435-003-0087-y
- Dallai R, Beani L, Kathirithamby J, Lupetti P, Afzlius BA. 2003b. New findings on sperm ultrastructure of Xenos vesparum (Rossi) (Strepsiptera, Insecta). Tissue Cell. 35:19–27. doi: 10.1016/S0040-8166(02)00099-X
- Foelix RF. 2011. Biology of spiders. 3rd ed. Oxford: Oxford University Press.
- Foellmer MW, Fairbairn DJ. 2004. Males under attack: sexual cannibalism and its consequences for male morphology and behavior in an orb-weaving spider. Evol Ecol Res. 6:163–181.
- Gomez Montono L, Magana C, Tourmente M, Martin-Coello J, Crespo C, Luque-Larena JJ, Gormendio M, Roldan ER. 2011. Sperm competition, sperm numbers and sperm quality in muroid rodents. PLos ONE. 6(3):e18173. doi: 10.1371/journal.pone.0018173
- Gullan PJ, Cranston PS. 2010. The insects: an outline of entomology. 4th ed. Oxford: Wiley-Blackwell.
- el Jundi B, Heinze S, Lenschow C, Kurylas A, Rohlfing R, Homberg U. 2010. The locust standard brain: a 3D standard of the central complex as a platform for neural network analysis. Front Syst Neurosci. 3(21):1–15.
- Kurylas AE, Rohlfing T, Krofczik S, Jenett A, Homberg U. 2008. Standardized Atlas of the brain of the desert locust, Schistocerca gregaria. Cell Tissue Res. 333:125–145. doi: 10.1007/s00441-008-0620-x
- Kim H, Kim JK, Moon MJ. 2014. Microstructural analysis on the testicular cyst of the wolf spider by 3D volume rendering. Entomol Res. 44:31–38. doi: 10.1111/1748-5967.12047
- Kim H, Jeong SR, Moon MJ. 2015. Structural analysis of theridiid spider’s testicular cyst using 3D reconstruction rendering. Entomol Res. 45:261–267. doi: 10.1111/1748-5967.12121
- Klann AE, Peretti AF, Alberti G. 2005. Ultrastructure of male genital system and spermatozoa of a Mexican camel-spider of the Eremobates pallipes species groupo (Arachnida, Solifugae). J Arachnol. 33:613–621. doi: 10.1636/04-111.1
- Marotta R, Ruhberg H. 2004. Sperm ultrastructure of an oviparous and an ovoviviparous onychophoran species (Peripatopsidae) with some phylogenetic considerations. J Zool Syst Evol Res. 42:313–322. doi: 10.1111/j.1439-0469.2004.00278.x
- Meisner AD, Klaus AV, O’Leary MA. 2005. Sperm head morphology in 36 species of artiodactylans, perissodactylans, and cetaceans (Mammalia). J Morphol. 263:179–202. doi: 10.1002/jmor.10297
- Michalik P, Dallai R, Giusti F, Albert G. 2004a. The ultrastructure of the peculiar synspermia of some Dysderidae (Araneae, Arachnida). Tissue Cell. 36:447–460. doi: 10.1016/j.tice.2004.08.001
- Michalik P, Haupt J, Alberti G. 2004b. On the occurrence of coenospermia in mesothelid spiders (Araneae: Heptathelidae). Arthropod Struct Dev. 33:173–181. doi: 10.1016/j.asd.2004.02.001
- Michalik P, Huber BA. 2006. Spermiogenesis in Psilochorus simony (Berland, 1911) (Pholcidae, Araneae): evidence for considerable within-family variation in sperm structure and development. Zoology. 109:14–25. doi: 10.1016/j.zool.2005.09.010
- Michalik P, Ramirez MJ. 2014. Evolutionary morphology of the male reproductive system, spermatozoa and seminal fluid of spiders (Arenaea, Arachnida) current knowledge and future directions. Arthropod Struct Dev. 43:291–322. doi: 10.1016/j.asd.2014.05.005
- Michalik P, Rittschof CC. 2011. A comparative analysis of the morphology and evolution of permanent sperm depletion in spiders. PLoS ONE. 6:e16014. doi: 10.1371/journal.pone.0016014
- Moon MJ, Tillinghast EK. 2013. Immunoreactivity of glutamic acid decarboxylase (GAD) isoforms in the central nervous system of the barn spider, Araneus cavaticus. Entomol Res. 43:47–54. doi: 10.1111/j.1748-5967.2012.00475.x
- Owusu-Daaku KO, Butler RD, Wood RJ. 1993. Spermiogenesis and meiotic drive in Aedes aegypti. Proceedings of the first international conference on urban pests. Wildey KB, Robinson WmH, editors; p. 345–354.
- Park JG, Moon MJ. 2014. Fine structural analysis on triad spinning spigots of an orb-web spider's capture threads. Entomol Res. 44:121–129. doi: 10.1111/1748-5967.12057
- Phillips DM. 1970. Insect sperm: their structure and morphogenesis. J Cell Biol. 44:243–277. doi: 10.1083/jcb.44.2.243
- Phillips DM. 1974. Nuclear shaping in the absence of microtubules in scorpion spermatids. J Cell Biol. 62:911–917. doi: 10.1083/jcb.62.3.911
- Rohlfing T, Brandt R, Maurer CR Jr., Menzel R. 2001. Bee brains, B-splines and computational democracy: generating an average shape atlas. Proceeding of IEEE workshop on Mathematical Methods in Biomedical Image Analysis; p. 187–194.
- Rybak J, Kuß A, Lamecker H, Zachow S, Hege HC, Lienhard M, Singer J, Neubert K, Menzel R. 2010. The digital bee brain: integrating and managing neurons in a common 3D reference system. Front Syst Neurosci. 4(30):1–15.
- Scheltinga DM, Wilkinson M, Jamieson BGM, Oommen OV. 2003. Ultrastructure of the mature spermatozoa of caecilians (Amphibia: Gymnophiona). J Morphol. 258:179–192. doi: 10.1002/jmor.10139
- Simmons LW. 2001. Sperm competition and its evolutionary consequences in the insects. Princeton (NJ): Princeton University Press.
- Šrámek M. 2006. 20 years of volume rendering. In: Spring Conference on Computer Graphics: Conference Proceedings; Comenius University, Bratislava; p. 11–19.
- Smid HM, Bleeker MA, van Loon JJ. 2003. Three-dimensional organization of the glomeruli in the antennal lobe of the parasitoid wasps Cotesia glomerata and C. rubecula. Cell Tissue Res. 312:237–248.
- Tourmente M, Gomendio M, Roldan ERS. 2011. Sperm competition and the evolution of sperm design in mammals. BMC Evol Biol. 11(12):1–10.
- Virkki N. 1969. Sperm bundles and phylogenies. Z Zellforsch. 101:13–27. doi: 10.1007/BF00335583
- Zizzari ZV, Lupetti P, Mencarelli C, Dallai R. 2008. Sperm ultrastructure and spermiogenesis of Coniopterygidae (Neuroptera, Insecta). Arthropod Struct Dev. 37:410–417. doi: 10.1016/j.asd.2008.03.001