ABSTRACT
The cartilage vascularization and chondrocyte survival are essential for endochondral ossification which occurs in the process of antler growth. Angiopoietins (Ang) is a family of major angiogenic growth factors and involved in regulating the vascularization. However, the expression and regulation of Angs in the antler are still unknown. The aim of this study is to localize the expression of Ang-1, Ang-2 and their receptor Tie-2 in sika deer antler using in situ hybridization and focused on analyzing the regulation of testosterone, estrogen, all-trans-retinoic acid (ATRA) and 9cRA on their expression in antler chondrocytes. The results showed that Ang-1, Ang-2 and Tie-2 were highly expressed in antler chondrocytes. Administration of testosterone to antler chondrocytes led to a notable increase in the expression of Ang-1 and Tie-2, and a reduction in the expression of Ang-2. The similar result was also observed after estrogen treatment. In contrast, ATRA and 9cRA could inhibit the expression of Ang-1 in antler chondrocytes and heighten the expression of Ang-2. Simultaneously, ATRA could downregulate the expression of Tie-2 in antler chondrocytes at 12 and 24 h, while 9cRA upregulate the expression of Tie-2 at 3 and 6 h. Collectively, Ang-1, Ang-2 and Tie-2 are expressed in antler chondrocytes and their expression can be affected by testosterone, estrogen, ATRA and 9cRA.
Introduction
Deer antlers are bony appendages that develop from permanent outgrowth of the frontal bone known as pedicle (Kierdorf et al. Citation2009). It is not only one of the most important medicines, but also a unique mammalian organ that can fully grow back once lost from its pedicle (Sui et al. Citation2014; Deb-Choudhury et al. Citation2015). Therefore, antlers probably offer the most pertinent model for studying organ regeneration in mammals. Although accumulating evidence has demonstrated that testosterone, estrogen and retinoic acid might participate in the process of antler growth, their underlying mechanisms remain poorly understood (Lincoln & Tyler Citation1999; Kierdorf & Bartos Citation1999; Li et al. Citation2009; Bartos et al. Citation2009). Antler regeneration involves endochondral ossification which includes cartilage development, calcification, removal and replacement by bone (Gomez et al. Citation2013; Jing et al. Citation2015). Moreover, the new blood vessels invasion into cartilage may be important for endochondral ossification, especially in the cartilage development (Bluteau et al. Citation2007). The antler cartilage zone contains columns of chondrocytes and large blood vessels which feed and nourish the rapidly growing antler (Clark et al. Citation2006a). Therefore, cartilage vascularization may be essential for antler regeneration. It has been previously reported that vascular endothelial growth factor (VEGF), which was a major angiogenic factor and might modulate new blood vessels invasion into the cartilage, was highly expressed in antler chondrocytes (Tink & Barnstable Citation2004; Clark et al. Citation2006b). Further evidence determined that VEGF effects were complemented and coordinated by angiopoietins (Ang) (Valable et al. Citation2005; Makinde et al. Citation2006).
Ang is involved in the maturation, stabilization and remodeling of vessels (Toyama et al. Citation2009). Four members of the Ang family have been discovered and the best studied are angiopoietin-1 (Ang-1) and Ang-2 which regulate the angiogenesis in the process of endochondral ossification and participate in cartilage vascularization and chondrocyte maturation (Jing et al. Citation2013; Kim et al. Citation2013). Ang-1 and Ang-2 exert their biological effects through activating or blocking the activation of their receptor tyrosine kinase with immunoglobulin and epidermal growth factor homology domains (Tie-2). Ang-1 can induce the autophosphorylation of Tie-2, promote endothelial cells survival and induce their migration and sprouting. In contrast, Ang-2 acts as a natural antagonist for Ang-1 to disrupt the angiogenesis (Maisonpierre et al. Citation1997). It binds to Tie-2 with a similar affinity of Ang-1, but does not induce receptor phosphorylation. Indeed, Ang-2 competitively inhibits the activation of Tie-2 receptor and plays an opposing effect with Ang-1 (Maisonpierre et al. Citation1997). Although previous study had showed that Ang-1 and Ang-2 were highly expressed in rabbit condylar chondrocytes (Jing et al. Citation2013), there were no reports whether they were also expressed in antler cartilage. The present study was undertaken to examine the expression of Ang-1, Ang-2 and Tie-2 in sika deer antler and then focused our attention on analyzing the regulation of testosterone, estrogen, all-trans-retinoic acid (ATRA) and 9-cis-retinoic acid (9cRA) on their expression in antler chondrocytes.
Materials and methods
Tissue collection
Antler tissues from three-year-old health sika deer as growing for about 60 days were collected as previously described (Zhang et al. Citation2017). Briefly, the distal 5 cm of growing tip was removed and sectioned sagittally along the longitudinal axis. A part of the tip was flash frozen in liquid nitrogen and then stored at −70°C for in situ hybridization. The remaining tip was used for isolation of antler chondrocytes.
Isolation and treatment of antler chondrocytes
The antler chondrocytes from sika deer were isolated and cultured as previously described (Zhang et al. Citation2017). Cultured chondrocytes were treated with testosterone (10−10 g/ml), estradiol-17β (10−11 g/ml), ATRA (1 nM) or 9cRA (1 nM) for 3, 6, 12 and 24 h. Testosterone and estradiol-17β were dissolved in dimethyl sulfoxide, while ATRA and 9cRA were melted in ethanol. Controls received the vehicle only.
In situ hybridization
Total RNA from antler cartilage was reverse-transcribed and amplified with the corresponding primers (). The cloned fragment of different gene was inserted into pGEM-T plasmid and then amplified with the primers for T7 and SP6 to prepare templates which were used to label antisense and sense cRNA probes according to the instructions of DIG RNA labeling kit (Roche Diagnostics GmbH, Mannheim, Germany).
Table 1. Primers used in this study.
Frozen sections (10 μm) were mounted on 3-aminopropyltriethoxy-silane (Sigma)-coated slides and fixed in 4% paraformaldehyde solution in phosphate-buffered saline. Hybridization was performed as described previously (Li et al. Citation2016). The digoxigenin-labeled sense probe was used as a negative control. Endogenous alkaline phosphatase activity was inhibited with 2 mM levamisole. All of the sections were counterstained with 1% methyl green. The positive signal was visualized as a dark brown color.
Real-time PCR
Total RNAs from cultured cells were isolated using TRIPURE reagent (Roche) according to the manufacturer’s protocol and reverse-transcribed into cDNA using moloney murine leukemia virus reverse-transcriptase (Promega). The expression levels of different genes were validated by real-time PCR analysis using FS Universal SYBR Green Real Master (Roche) on BIO-RAD CFX96TM Real-Time Detection System according to manufacturer’s instructions. Data were normalized to Gapdh expression and analyzed using the 2−ΔΔCt method. All reactions were repeated at least three times. Primer sequences used for real-time PCR were listed in .
Statistics
All data were presented as mean ± SEM. Each experiment was independently repeated at least three times. The significance of difference was analyzed by Independent-Samples T-Test. A P-value <0.05 was considered as statistically significant (*P < 0.05).
Results
Expression of Ang-1, Ang-2 and Tie-2 mRNA in sika deer antler
In situ hybridization was used to localize the distribution of Ang-1, Ang-2 and Tie-2 mRNA in sika deer antler. The results showed that Ang-1 mRNA was localized in mesenchymal cells ((A)). Simultaneously, a significantly high level of Ang-1 expression was noted in antler chondrocytes ((B)). Ang-2 expression was visualized in the chondrocytes, while no visible signal was found in mesenchymal cells ((C,D)). The expression pattern of Tie-2 mRNA in the antler was very similar to that of Ang-2 ((E,F)).
Figure 1. In situ hybridization of Ang-1, Ang-2 and Tie-2 expression in sika deer antler. (A), Ang-1 expression in antler mesenchyme. (B), Ang-1 expression in antler cartilage. (C), Ang-2 expression in antler mesenchyme. (D), Ang-2 expression in antler cartilage. (E), Tie-2 expression in antler mesenchyme. (F), Tie-2 expression in antler cartilage. M, mesenchymal cells; C, chondrocytes. Bar = 60 μm.
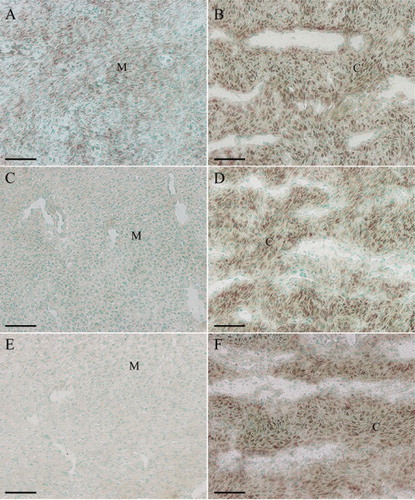
Effects of testosterone on the expression of Ang-1, Ang-2 and Tie-2 mRNA in antler chondrocytes
In antler chondrocytes, testosterone could significantly induce the expression of Ang-1 mRNA at 6, 12 and 24 h (, (A)). In contrast, administration of testosterone to the chondrocytes led to a notable reduction in the expression of Ang-2, which reached a nadir at 24 h (, (B)). In the meantime, a gradual increase in the level of Tie-2 mRNA was observed between 3 and 24 h and reached the highest level at 24 h (, (C)).
Figure 2. Effects of testosterone on the expression of Ang-1, Ang-2 and Tie-2 in antler chondrocytes. After antler chondrocytes were treated with testosterone for 3, 6, 12 and 24 h, the expression of Ang-1 (A), Ang-2 (B) and Tie-2 (C) was determined by real-time PCR. Data are shown mean ± SEM. Asterisks denote significance (P < 0.05).
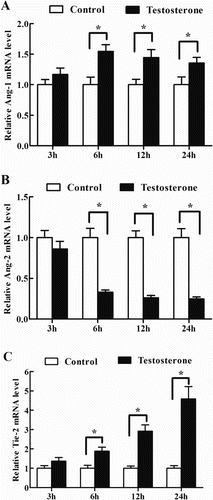
Table 2. Effects of testosterone on the expression of Ang-1, Ang-2 and Tie-2 mRNA in antler chondrocytes.
Effects of estrogen on Ang-1, Ang-2 and Tie-2 mRNA expression in antler chondrocytes
To evaluate the regulation of estrogen on the expression of Ang-1, Ang-2 and Tie-2, antler chondrocytes were treated with estrogen for 3, 6, 12 and 24 h. The results found that a gradual increase in the level of Ang-1 mRNA was observed between 3 and 24 h after estrogen treatment and reached a peak at 24 h (, (A)). Conversely, Ang-2 mRNA expression was gradually decreased and reached a nadir at 24 h (, (B)). After antler chondrocytes were treated with estrogen, Tie-2 mRNA expression was obviously enhanced at 12 and 24 h (, (C)).
Figure 3. Effects of estrogen on the expression of Ang-1, Ang-2 and Tie-2 in antler chondrocytes. After antler chondrocytes were treated with estrogen for 3, 6, 12 and 24 h, the expression of Ang-1 (A), Ang-2 (B) and Tie-2 (C) was determined by real-time PCR.
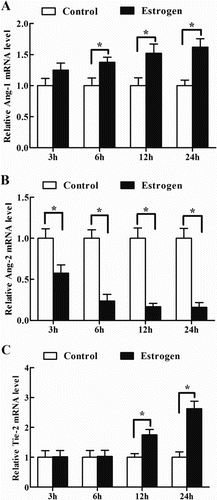
Table 3. Effects of estrogen on the expression of Ang-1, Ang-2 and Tie-2 mRNA in antler chondrocytes.
Effects of ATRA on Ang-1, Ang-2 and Tie-2 mRNA expression in antler chondrocytes
Because ATRA is considered to be the biologically active isomer of RA, we treated antler chondrocytes with ATRA to see its regulation on the expression of Ang-1, Ang-2 and Tie-2. The result revealed that ATRA could time-dependently inhibit the expression of Ang-1 which reached the minimum at 24 h, and heighten the expression of Ang-2 at 12 and 24 h (, (A,B)). In the meantime, ATRA treatment resulted in a marked decline in the expression of Tie-2 at 12 and 24 h (, (C)).
Figure 4. Effects of ATRA on the expression of Ang-1, Ang-2 and Tie-2 in antler chondrocytes. After antler chondrocytes were treated with ATRA for 3, 6, 12 and 24 h, the expression of Ang-1 (A), Ang-2 (B) and Tie-2 (C) was determined by real-time PCR.
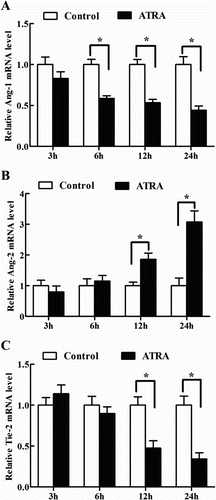
Table 4. Effects of ATRA on the expression of Ang-1, Ang-2 and Tie-2 mRNA in antler chondrocytes.
Effects of 9cRA on Ang-1, Ang-2 and Tie-2 mRNA expression in antler chondrocytes
Administration of 9cRA to antler chondrocytes caused downregulation of Ang-1 expression at 12 and 24 h (, (A)). On the contrary, the levels of Ang-2 mRNA showed an increase at 12 and 24 h after 9cRA treatment (, (B)). Interestingly, Tie-2 expression was gradually decreased and reached a level similar to that of the control at 24 h, although 9cRA could stimulate the expression of Tie-2 in antler chondrocytes at 3 and 6 h compared with that in the control cells (, (C)).
Figure 5. Effects of 9cRA on the expression of Ang-1, Ang-2 and Tie-2 in antler chondrocytes. After antler chondrocytes were treated with 9cRA for 3, 6, 12 and 24 h, the expression of Ang-1 (A), Ang-2 (B) and Tie-2 (C) was determined by real-time PCR.
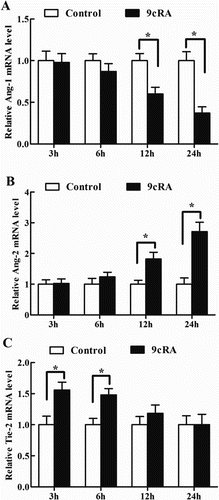
Table 5. Effects of 9cRA on the expression of Ang-1, Ang-2 and Tie-2 mRNA in antler chondrocytes.
Discussion
The Ang family has been confirmed to be involved in vasculature development and angiogenesis (Daponte et al. Citation2013). In this study, we examined the expression of Ang-1, Ang-2 and Tie-2 in sika deer antler using in situ hybridization. The results revealed that Ang-1 mRNA was highly expressed in antler chondrocytes. This was consistent with the previous evidence that VEGF mRNA signal was observed in antler chondrocytes (Clark et al. Citation2006b). Based on the expression pattern of VEGF and Ang-1 in antler cartilage, we conjectured that they might play a similar role of promoting new blood vessel invasion into cartilage and keeping chondrocytes survival. Meanwhile, Ang-1 might activate Tie-2 which was also highly expressed in antler chondrocytes, and further stimulate blood vessel invasion into hypertrophic zone of cartilage (Jing et al. Citation2013; Kim et al. Citation2013). On the contrary, Ang-2 was a naturally occurring antagonist of Ang-1 and could competitively inhibit Ang-1-induced activation of Tie-2 (Escobar et al. Citation2004). The present study found that Ang-2 was visualized in antler chondrocytes, implying that Ang-2 might antagonize the role of Ang-1, prevent blood vessels excessive invasion and induce chondrocyte apoptosis. The viewpoint was consistent with the evidence that Ang-2 could exert the foundation of inducing endothelial cells and astrocyte apoptosis and vessel regression (Lobov et al. Citation2002; Oshima et al. Citation2005; Yun et al. Citation2016).
It is well established that testosterone is crucial for antler growth. Castration of prepubertal male deer abolishes further pedicle and antler formation, while castration of adult males prevents full antler calcification. Moreover, administration of exogenous testosterone could overcome the abnormalities of castration (Li et al. Citation2009). In antler chondrocytes, testosterone could promote the expression of Ang-1 and Tie-2, and inhibit the expression of Ang-2, suggesting that testosterone might regulate cartilage vascularization and chondrocytes survival in growing antler through Ang-1, Ang-2 and Tie-2. This evidence was consistent with the previous finding that dihydrotestosterone could enhance neovascularization in the context of ischemic injury of the male mouse and induce endothelial cell survival (Sieveking et al. Citation2010; Cai et al. Citation2011).
It has previously showed that testosterone could convert into estrogen which was the most potent steroid causing maturation and mineralization of antlers in intact and castrated deer (Lincoln & Tyler Citation1999; Bubenik et al. Citation2005). In buck antler, estrogen could also be compounded (Kierdorf et al. Citation2009). Moreover, estrogen could upregulate the expression of Ang-1 and Tie-2 in antler chondrocytes, and downregulate the expression of Ang-2, indicating that estrogen might produce the similar biological effects with that of testosterone. Indeed, estrogen could induce angiogenesis in adenomyosis development via activating the E2-Slug-VEGF pathway (Huang et al. Citation2014).
RA is the biologically active metabolite of vitamin A and exists as several geometric isomers including ATRA, 9cRA, 13cRA, etc. (Thatcher & Isoherranen Citation2009). Injection of ATRA into the growing pedicle led to a significant increase in first antler volume (Kierdorf & Bartos Citation1999), suggesting a role of ATRA in antler growth. The present study showed that ATRA could induce the expression of Ang-2, and suppress the expression of Ang-1 and Tie-2, demonstrating that ATRA might play a role in preventing cartilage excessive vascularization in the antler growth. The notion was further confirmed in cancer cells (Kini et al. Citation2001; Hoffmann et al. Citation2007; Vourtsis et al. Citation2013; Liang et al. Citation2014). 9cRA also appears to have biological activity and has been used for treatment of Kaposi’s sarcoma and chronic hand eczema (Nelson et al. Citation2013). In antler chondrocytes, regulation of Ang-1, Ang-2 and Tie-2 by 9cRA reveals a role of 9cRA in cartilage vascularization. Together these data evidenced that ATRA and 9cRA might synergistically inhibit the vascularization of antler cartilage and seem to have an antagonistic role of testosterone and estrogen. Indeed, in human microvascular endothelial cells, ATRA and 9cRA might withstand the effect of testosterone on the formation of capillary-like tubular structures (Lansink et al., Citation1998). Meanwhile, the inhibitory action of RA on the survival of breast cancer cell also could be antagonized by estrogen (Saumet et al., Citation2012). These results suggested that ATRA and 9cRA might govern the vascularization of antler cartilage together with estrogen and testosterone to maintain normal antler growth.
In summary, Ang-1, Ang-2 and Tie-2 are expressed in antler chondrocytes and their expression can be affected by testosterone, estrogen, ATRA and 9cRA.
Disclosure statement
No potential conflict of interest was reported by the authors.
ORCID
Hong-Liang Zhang http://orcid.org/0000-0002-2834-4839
Additional information
Funding
References
- Bartos L, Schams D, Bubenik GA. 2009. Testosterone, but not IGF-1, LH, prolactin or cortisol, may serve as antler-stimulating hormone in red deer stags (Cervus elaphus). Bone. 44:691–698. doi: 10.1016/j.bone.2008.12.004
- Bluteau G, Julien M, Magne D, Mallein-Gerin F, Weiss P, Daculsi G, Guicheux J. 2007. VEGF and VEGF receptors are differentially expressed in chondrocytes. Bone. 40:568–576. doi: 10.1016/j.bone.2006.09.024
- Bubenik GA, Miller KV, Lister AL, Osborn DA, Bartos L, van der Kraak GJ. 2005. Testosterone and estradiol concentrations in serum, velvet skin, and growing antler bone of Male White-tailed deer. J Exp Zool A Comp Exp Biol. 303:186–192. doi: 10.1002/jez.a.139
- Cai J, Hong Y, Weng C, Tan C, Imperato-McGinley J, Zhu YS. 2011. Androgen stimulates endothelial cell proliferation via an androgen receptor/VEGF/cyclin A-mediated mechanism. Am J Physiol Heart Circ Physiol. 300:H1210–H1221. doi: 10.1152/ajpheart.01210.2010
- Clark DE, Li C, Wang W, Martin SK, Martin SK, Suttie JM. 2006a. Vascular localization and proliferation in the growing tip of the deer antler. Anat Rec A Discov Mol Cell Evol Biol. 288:973–981. doi: 10.1002/ar.a.20364
- Clark DE, Lord EA, Suttie JM. 2006b. Expression of VEGF and pleiotrophin in deer antler. Anat Rec A Discov Mol Cell Evol Biol. 288:1281–1293. doi: 10.1002/ar.a.20393
- Daponte A, Deligeoroglou E, Pournaras S, Tsezou A, Garas A, Anastasiadou F, Hadjichristodoulou C, Messinis IE. 2013. Angiopoietin-1 and angiopoietin-2 as serum biomarkers for ectopic pregnancy and missed abortion-A case–control study. Clin Chim Acta. 415:145–151. doi: 10.1016/j.cca.2012.10.031
- Deb-Choudhury S, Wang W, Clerens S, McMahon C, Dyer JM, Li C. 2015. Direct localisation of molecules in tissue sections of growing antler tips using MALDI imaging. Mol Cell Biochem. 409:225–241. doi: 10.1007/s11010-015-2527-7
- Escobar E, Rodríguez-Reyna TS, Arrieta O, Sotelo J. 2004. Angiotensin II, cell proliferation and angiogenesis regulator: biologic and therapeutic implications in cancer. Curr Vasc Pharmacol. 2:385–399. doi: 10.2174/1570161043385556
- Gomez S, Garcia AJ, Luna S, Kierdorf U, Kierdorf H, Gallego L, Landete-Castillejos T. 2013. Labeling studies on cortical bone formation in the antlers of red deer (Cervus elaphus). Bone. 52:506–515. doi: 10.1016/j.bone.2012.09.015
- Hoffmann S, Rockenstein A, Ramaswamy A, Celik I, Wunderlich A, Lingelbach S, Hofbauer LC, Zielke A. 2007. Retinoic acid inhibits angiogenesis and tumor growth of thyroid cancer cells. Mol Cell Endocrinol. 264:74–81. doi: 10.1016/j.mce.2006.10.009
- Huang TS, Chen YJ, Chou TY, Chen CY, Li HY, Huang BS, Tsai HW, Lan HY, Chang CH, Twu NF, et al. 2014. Oestrogen-induced angiogenesis promotes adenomyosis by activating the slug-VEGF axis in endometrial epithelial cells. J Cell Mol Med. 18:1358–1371. doi: 10.1111/jcmm.12300
- Jing J, Hinton RJ, Feng JQ. 2015. Bmpr1a signaling in cartilage development and endochondral bone formation. Vitam Horm. 99:273–291. doi: 10.1016/bs.vh.2015.06.001
- Jing Z, Gu Z, Feng J. 2013. Forward mandibular positioning enhances the expression of Ang-1 and Ang-2 in rabbit condylar chondrocytes. Mol Med Rep. 8:1094–1098.
- Kierdorf U, Bartos L. 1999. Treatment of the growing pedicle with retinoic acid increased the size of first antlers in fallow deer (Dama dama L.). Comp Biochem Physiol C Pharmacol Toxicol Endocrinol. 124:7–9. doi: 10.1016/S0742-8413(99)00038-9
- Kierdorf U, Li C, Price JS. 2009. Improbable appendages: deer antler renewal as a unique case of mammalian regeneration. Semin Cell Dev Biol. 20:535–542. doi: 10.1016/j.semcdb.2008.11.011
- Kim S, Lee JC, Cho ES, Kwon J. 2013. COMP-Ang1 accelerates chondrocyte maturation by decreasing HO-1 expression. J Cell Biochem. 114:2513–2521. doi: 10.1002/jcb.24596
- Kini AR, Peterson LA, Tallman MS, Lingen MW. 2001. Angiogenesis in acute promyelocytic leukemia: induction by vascular endothelial growth factor and inhibition by all-trans retinoic acid. Blood. 97:3919–3924. doi: 10.1182/blood.V97.12.3919
- Lansink M, Koolwijk P, van Hinsbergh V, Kooistra T. 1998. Effect of steroid hormones and retinoids on the formation of capillary-like tubular structures of human microvascular endothelial cells in fibrin matrices is related to urokinase expression. Blood. 92:927–938.
- Li C, Yang F, Sheppard A. 2009. Adult stem cells and mammalian epimorphic regeneration-insights from studying annual renewal of deer antlers. Curr Stem Cell Res Ther. 4:237–251. doi: 10.2174/157488809789057446
- Li DD, Zhao SY, Yang ZQ, Duan CC, Guo CH, Zhang HL, Geng S, Yue ZP, Guo B. 2016. Hmgn5 functions downstream of Hoxa10 to regulate uterine decidualization in mice. Cell Cycle. 15:2792–2805. doi: 10.1080/15384101.2016.1220459
- Liang C, Guo S, Yang L. 2014. Effects of all-trans retinoic acid on VEGF and HIF-1α expression in glioma cells under normoxia and hypoxia and its anti-angiogenic effect in an intracerebral glioma model. Mol Med Rep. 10:2713–2719.
- Lincoln GA, Tyler NJ. 1999. Role of oestradiol in the regulation of the seasonal antler cycle in female reindeer, Rangifer tarandus. J Reprod Fertil. 115:167–174. doi: 10.1530/jrf.0.1150167
- Lobov IB, Brooks PC, Lang RA. 2002. Angiopoietin-2 displays VEGF-dependent modulation of capillary structure and endothelial cell survival in vivo. Proc Natl Acad Sci U S A. 99:11205–11210. doi: 10.1073/pnas.172161899
- Maisonpierre PC, Suri C, Jones PF, Bartunkova S, Wiegand SJ, Radziejewski C, Compton D, McClain J, Aldrich TH, Papadopoulos N, et al. 1997. Angiopoietin-2, a natural antagonist for Tie2 that disrupts in vivo angiogenesis. Science. 277:55–60. doi: 10.1126/science.277.5322.55
- Makinde T, Murphy RF, Agrawal DK. 2006. Immunomodulatory role of vascular endothelial growth factor and angiopoietin-1 in airway remodeling. Curr Mol Med. 6:831–841. doi: 10.2174/156652406779010795
- Nelson CH, Buttrick BR, Isoherranen N. 2013. Therapeutic potential of the inhibition of the retinoic acid hydroxylases CYP26A1 and CYP26B1 by xenobiotics. Curr Top Med Chem. 13:1402–1428. doi: 10.2174/1568026611313120004
- Oshima Y, Oshima S, Nambu H, Kachi S, Takahashi K, Umeda N, Shen J, Dong A, Apte RS, Duh E, et al. 2005. Different effects of angiopoietin-2 in different vascular beds: new vessels are most sensitive. FASEB J. 19:963–965.
- Saumet A, Vetter G, Bouttier M, Antoine E, Roubert C, Orsetti B, Theillet C, Lecellier CH. 2012. Estrogen and retinoic acid antagonistically regulate several microRNA genes to control aerobic glycolysis in breast cancer cells. Mol Biosyst. 8:3242–3253. doi: 10.1039/c2mb25298h
- Sieveking DP, Lim P, Chow RW, Dunn LL, Bao S, McGrath KC, Heather AK, Handelsman DJ, Celermajer DS, Ng MK. 2010. A sex-specific role for androgens in angiogenesis. J Exp Med. 207:345–352. doi: 10.1084/jem.20091924
- Sui Z, Zhang L, Huo Y, Zhang Y. 2014. Bioactive components of velvet antlers and their pharmacological properties. J Pharm Biomed Anal. 87:229–240. doi: 10.1016/j.jpba.2013.07.044
- Thatcher JE, Isoherranen N. 2009. The role of CYP26 enzymes in retinoic acid clearance. Expert Opin Drug Metab Toxicol. 5:875–886. doi: 10.1517/17425250903032681
- Tink JT, Barnstable CJ. 2004. Osteoblasts and osteoclasts express PEDF, VEGF-A isoforms, and VEGF receptors: possible mediators of angiogenesis and matrix remodeling in the bone. Biochem Biophys Res Commun. 316:573–579. doi: 10.1016/j.bbrc.2004.02.076
- Toyama K, Honmou O, Harada K, Suzuki J, Houkin K, Hamada H, Kocsis JD. 2009. Therapeutic benefits of angiogenetic gene-modified human mesenchymal stem cells after cerebral ischemia. Exp Neurol. 216:47–55. doi: 10.1016/j.expneurol.2008.11.010
- Valable S, Montaner J, Bellail A, Berezowski V, Brillault J, Cecchelli R, Divoux D, Mackenzie ET, Bernaudin M, Roussel S, Petit E. 2005. VEGF-induced BBB permeability is associated with an MMP-9 activity increase in cerebral ischemia: both effects decreased by Ang-1. J Cereb Blood Flow Metab. 25:1491–1504. doi: 10.1038/sj.jcbfm.9600148
- Vourtsis D, Lamprou M, Sadikoglou E, Giannou A, Theodorakopoulou O, Sarrou E, Magoulas GE, Bariamis SE, Athanassopoulos CM, Drainas D, et al. 2013. Effect of an all-trans-retinoic acid conjugate with spermine on viability of human prostate cancer and endothelial cells in vitro and angiogenesis in vivo. Eur J Pharmacol. 698:122–130. doi: 10.1016/j.ejphar.2012.11.007
- Yun JH, Park SW, Kim JH, Park YJ, Cho CH, Kim JH. 2016. Angiopoietin 2 induces astrocyte apoptosis via αvβ5-integrin signaling in diabetic retinopathy. Cell Death Dis. 7:e2101. doi: 10.1038/cddis.2015.347
- Zhang HL, Cao H, Yang ZQ, Geng S, Wang K, Yu HF, Guo B, Yue ZP. 2017. 13cRA regulates the differentiation of antler chondrocytes through targeting Runx3. Cell Biol Int. 41:296–308. doi: 10.1002/cbin.10724