ABSTRACT
Parabens are generally used as preservatives in foods, pharmaceuticals, and various other commercial products. Among them, ethylparaben has weaker estrogenic characteristics than endogenous estrogen. However, growing evidence indicates that ethylparaben has an adverse effect on various human tissues. Here, we investigated whether ethylparaben induces cell death by affecting cell viability, cell proliferation, cell cycle, and apoptosis using the human placenta cell line BeWo. Ethylparaben significantly decreased cell viability in a dose-dependent manner. It caused cell cycle arrest at sub-G1 by reducing the expression of cyclin D1, whereas it decreased the cell proportion at the G0/G1 and S phases. Furthermore, we verified that ethylparaben induces apoptotic cell death by enhancing the activity of Caspase-3. Taken together, our results suggest that ethylparaben exerts cytotoxic effects in human placental BeWo cells via cell cycle arrest and apoptotic pathways.
Introduction
Parabens, also known as alkyl esters of 4-hydroxybenzoic acid, are preservatives that are often used in consumer products, including foods and pharmaceuticals owing to their antimicrobial and antifungal properties (Soni et al. Citation2005). Humans are generally exposed to parabens through ingestion, inhalation, and dermal absorption (Smith et al. Citation2013). Parabens are quickly metabolized in the human body and are mostly excreted through urine (Janjua et al. Citation2008). The parent compounds found in urine can, thus, be convincing biomarkers of parabens exposure (Ye et al. Citation2006).
Although parabens are officially declared as safe to use, toxicological concerns regarding their use have been raised in the past 20 years (Andersen Citation2008). Growing evidence suggests that parabens acting as xenoestrogens have an adverse effect on the endocrine system (Okubo et al. Citation2001; Darbre and Harvey Citation2008; Golden et al. Citation2008) even if they have a lower estrogen receptor binding affinity than endogenous estrogen. The functional activity of parabens in antimicrobial and estrogenic processes tends to increase with the alkyl substituent length. Ethylparaben has a shorter alkyl group and lower ability to inhibit microbial growth than other parabens (Bledzka et al. Citation2014). A previous study performed in fish showed that the estrogenic activity of ethylparaben is approximately 60-fold weaker than those of propylparaben and butylparaben (Pedersen et al. Citation2000). The Scientific Commission on Consumer Safety (SCCS) stated that ethylparaben is regarded to be safe (SCCS Citation2013). There is no evidence regarding the negative effect of ethylparaben on the reproductive system (Taxvig et al. Citation2008). However, it has been reported that ethylparaben exists in the intact form in human breast cancer tissue (Darbre et al. Citation2004). More recent evidence has shown that ethylparaben may become an oxidative stress biomarker (Nishizawa et al. Citation2006; Kang et al. Citation2013). Despite these increasing evidences suggesting the toxicological relevance of parabens for human health, the safety of parabens for human consumption is still controversial.
Recent studies have focused on the potential of parabens exposure to human fetuses via the placenta. The adverse effects of parabens can result in severe and irreversible consequences for the developing fetus, which still has an immature detoxification system. Recent studies have detected parabens in the serum, breast milk, amniotic fluid, human placenta, cord blood, and urine of neonates (Vela-Soria et al. Citation2014; Pycke et al. Citation2015; Azzouz et al. Citation2016). High detection frequencies of ethylparaben were also revealed in cord blood plasma and maternal urine (Pycke et al. Citation2015). Therefore, although studies have shown that paraben exposure of the mother can adversely affect a fetus, there is little research on whether ethylparaben can directly affect the development and physiology of the placenta in humans.
In the present study, we investigated the cytotoxic effects of ethylparaben on the human placental cell line BeWo. We assessed the effect of ethylparaben on cell viability using the MTS and live/dead assays. Furthermore, we investigated the effects of ethylparaben on cell cycle progression and induction of apoptosis in BeWo cells.
Materials and methods
Chemicals and reagents
Ethylparaben (cat. no. 111988) and propidium iodide (cat. no. P4170) were obtained from Sigma-Aldrich (Oakville, Ontario, Canada); 1 M stock solution of ethylparaben was prepared and diluted in 100% EtOH. We used 0.1, 0.25, 0.5, and 1 mM of ethylparaben in DMEM containing 10% FBS for this study. LIVE/DEAD™ Viability/Cytotoxicity kit (cat. no. L3224, Carlsbad, CA) was purchased from Invitrogen. FITC Annexin-V Apoptosis Detection kit I (cat. no. 556547, La Jolla, CA) was obtained from BD pharmingenTM. Cell Titer 96 AQueous one solution cell proliferation assay kit (MTS) was procured from Promega Corporation (cat. no. G3581, Madison, USA). Antibodies against Ki-67 (cat. no. ab15580) was procured from Abchem, Cyclin B1 (cat. no. ab32053) was purchased from Santa Cruz Biotechnology (CA, USA) and Cyclin D1 (cat. no. 2978S) were obtained from Cell Signaling Technology Inc. (Beverly, MA). Goat anti-rabbit secondary antibody (cat. no. 111-095-144) was purchased from Jackson ImmunoResearch (West Grove, PA).
Cell cultures
All the cell culture reagents used in this study were purchased from Welgene (Seoul, Republic of Korea). BeWo cells, human placenta cell line, were purchased from the Korean Cell Line Bank (Seoul, Republic of Korea). Cells were maintained in Dulbecco’s modified Eagle medium (DMEM) supplemented with 10% fetal bovine serum (FBS), 1% penicillin/streptomycin at 37°C in an incubator with 5% CO2 at humidified atmosphere. For the experiments, the coverslips were treated with 0.1 mg/ml of poly-D lysine for 6 h at room temperature and it was placed in 100-mm cell culture dishes. Cells were seeded at a density of 2.5 × 106 cells per well in 100-mm cell culture dishes. After 24 h incubation, cells were treated with different concentration of ethylparaben in media supplemented with 10% FBS without antibiotics for 24 or 48 h. Coverslips with cells were used for Live/Dead cell assay. The other cells in 100-mm cell culture dishes were gently washed with Dulbecco’s phosphate-buffered saline without calcium and magnesium, and then cells were trypsinized. Cells were processed according to the different experimental analysis. In each assay, 0.6% EtOH was used as a negative control.
Cell viability assay
For the determination of cell viability, cells were seeded at the density of 2 × 104 cells per well in 48-well plates. After overnight incubation, cells were treated with the different concentrations of ethylparaben (0.1, 0.25, 0.5, 1 mM) in 100 μl culture medium containing 10% FBS for the indicated time points. The solvent of 0.6% of EtOH treated cells were served as control. Then, cell viability was assessed using CellTiter 96® AQueous One Solution Cell Proliferation Assay (Promega, Madison, USA). Twenty microliter of MTS (3-(4, 5-dimethylthiazol-2-yl)-5-(3-carboxymethoxyphenyl)-2-(4-sulfophenyl)-2H-tetrazolium) solution was added and the cells were incubated for 1 h at 37°C. The resultant cell viability was determined by measuring absorbance at 490 nm using Multiskan GO microplate reader (Waltham, MA).
Morphological changes and live/dead cell assay
To measure the effect of ethylparaben on cytotoxicity, cells were seeded in coated coverslip. Cells were treated with the indicated ethylparaben in different concentrations for the indicated time points. For this experiment, cells on the coverslip were placed to 24-well plate containing 300 μl of PBS. Morphological changes observed under the inverted microscope. Cells were stained with a mixture of two dyes: 0.3 μM calcein-AM, 3 μM of EthD-1. Dyes were prepared immediately in 1X PBS before use and added to the cells. After 30 min incubation in dark condition, dye mixture was not washed out and images were captured using Nikon Eclipse TE300 Inverted Fluorescence Microscope (Nikon Corp., Tokyo, Japan)
Annexin V-FITC/propidium iodide (PI) apoptosis assay
To assess the apoptosis, the cells were washed with PBS and collected by centrifugation for 3 min at 3000 rpm. Apoptosis was performed using FITC Annexin-V Apoptosis Detection Kit I (BD pharmingenTM, La Jolla, CA) according to the manufacturer’s instructions. Briefly, the collected cells were washed with 1X PBS and resuspended in 100 μl of 1X Annexin-V binding buffer (10 mM HEPES/NaOH; pH 7.4, 140 mM NaCl, 2.5 mM CaCl2); 5 μl of Annexin-V Alexa Fluor 488 and/or 5 μl of PI were added and incubated for 15 min incubation in dark condition. 1X Annexin-V binding buffer of 400 μl was added to wash the Annexin-V/PI stained cells. A minimum of 10,000 cells per sample was analyzed using BD Accuri™ C6 Plus (BD FACS, San Jose, CA).
Cell cycle analysis
Cell cycle analysis was performed using propidium iodide (PI) staining (Sigma). At the end of treatment, cells were trypsinized, and suspended cells were centrifuged at 3000 rpm for 3 min. The cells were fixed for 1 h in 70% ethanol with rotator at 4°C. After fixation, cells were centrifuged at 3000 rpm for 3 min, and the pellets were washed with ice-cold PBS. Cell pellets were then resuspended in 0.2 ml of PBS containing 0.25 μg/μl RNase A for 1 h at 37°C. After that, 10 μg/ml of PI was added and then incubated for 15 min at room temperature in a dark condition. Finally, 1X PBS was added into the PI-stained cells and were analyzed by BD Accuri™ C6 Plus (BD FACS, San Jose, CA). At least 10,000 cells were used for each analysis, and the results were displayed as histograms. The percentage of cell distribution in Sub-G1, G0/G1, S and G2/M phase were measured, and the results were analyzed by the BD Accuri™ C6 Plus software for cell cycle profile.
Immunostaining for FACS analysis
Cells were fixed for 5 h in 1% paraformaldehyde with rotator at 4°C and centrifuged at 3000 rpm for 3 min. Cell pellets were washed once with ice-cold 1X PBS. Cells were resuspended with 1X PBS and aliquoted each 100 μl of sample in 96-well round plate and centrifuged at 3000 rpm for 3 min. After removed supernatant, cells were resuspended with solution A (0.1% saponin, 0.1% BSA, 75 mM sodium acetate, 25 mM HEPES, final pH 7.2) containing the respective primary antibodies. Rabbit primary antibodies against Cyclin B1 (1:200), Cyclin D1 (1:200), Ki-67 (1:400), and cleaved Caspase-3 (1:200) were incubated for 1 h at 23°C in thermocycler with 300 rpm. And then, cells were washed and incubated with the secondary FITC-labeled goat anti-rabbit antibody (1:200) for 30 min at room temperature in a dark condition. Expression of the target protein was analyzed by BD Accuri™ C6 Plus (BD FACS, San Jose, CA). At least 10,000 cells were used for each analysis, and the results were displayed as histograms. FACS results were analyzed by the BD Accuri™ C6 Plus software. The percentage of the cell population was measured by FITC positive cells.
Statistical analysis
Values are presented as mean ± S.E.M. of experiments performed in triplicate. Data were analyzed by two-way ANOVA followed by Tukey’s multiple comparison test using GraphPad Prism software version 5.01 (California, USA). Differences between groups were considered as significant at p < .05.
Results
Ethylparaben treatment reduced the viability of human placenta BeWo cells
To assess the cytotoxic effects of ethylparaben, the cell viability of BeWo was first examined after treating them with various concentrations of ethylparaben at different time points ((A)). BeWo cells were cultured with increasing concentrations of ethylparaben (0.1–10 mM); cell viability was, then, assessed at 24 and 48 h using the MTS assay. Ethylparaben treatment induced a significant decrease in cell viability in a dose- and time-dependent manner ((A)). Compared to the untreated cells, cells treated with ethylparaben for 24 h showed decreased growth with an IC50 value of 0.9 mM, which further decreased to 0.6 mM at 48 h. Based on these results, we selected the ethylparaben concentration range of 0.1–1 mM for subsequent experiments. BeWo cells were treated with 0.1, 0.25, 0.5, and 1 mM of ethylparaben for the indicated time periods, and morphological changes were confirmed using phase contrast microscopy ((B)). Ethylparaben induced critical morphological changes in BeWo cells, which are typically seen during cell death, such as cell shrinkage, cell rounding, and membrane blebbing, in a dose-dependent manner ((B)). The number of cells also decreased greatly in ethylparaben-treated groups. Furthermore, cell viability was examined using the live/dead assay after the ethylparaben treatment of BeWo cells. Ethylparaben treatment significantly increased the number of dead cells in a dose- and time-dependent manner ((C)). These results showed that ethylparaben has cytotoxic effects in human placenta BeWo cells.
Figure 1. Effect of ethylparaben on cell morphology and viability of BeWo cells. (A) BeWo cell viability was measured using the MTS assay. Cells were treated with 0.1–10 mM of ethylparaben for 24 or 48 h. Data are presented as the percentage of the values obtained for the untreated cells used as the control. (B) Cell morphology was observed using phase-contrast microscopy at the indicated time points, for various concentrations of ethylparaben. (C) Cell viability was analyzed using the live/dead assay. Cells were stained with two dyes (Calcein-AM, 0.3 μM; EthD-1, 3 μM) for 30 min at room temperature (approximately 25°C) and stained cells were detected by fluorescence microscopy. Images represent three independent experiments. Scale bars represent 200 μm.
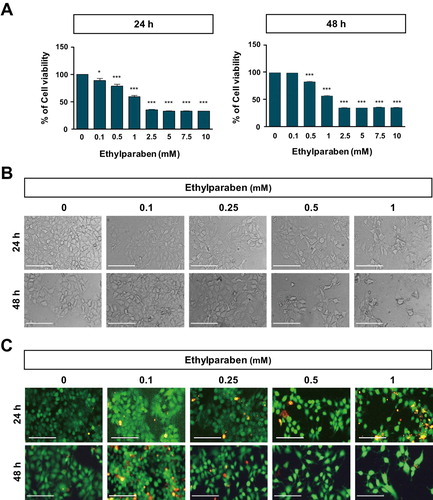
Ethylparaben treatment has no effect on BeWo cell proliferation and cell cycle arrest in the sub-G1 phase
Ki-67 is a common biomarker for cell proliferation and is used to examine the ratio of dividing cells for grading multiple types of cancers (Sun and Kaufman Citation2018). To test the effect of ethylparaben on the proliferation of BeWo cells, the protein expression of Ki-67 in BeWo cells was measured using flow cytometry analysis after treating them with ethylparaben. Cells were first treated with different concentrations of ethylparaben for the indicated time periods. After immunostaining with anti-Ki-67 antibody, the level of Ki-67 expression was measured using FACS analysis. Although ethylparaben slightly induced Ki-67 positive cells at both time points, the number of Ki-67 positive cells were not significantly changed (1.2–1.7-fold compared to the untreated control) (SD 1). To determine whether ethylparaben affects the cell cycle, BeWo cells were treated with different concentrations (0.1–1 mM) of ethylparaben at the indicated time points. Treatment with 1 mM ethylparaben for 24 h gradually increased the number of cells in the sub-G1 (12.5%) phase compared with the control (4.0%), while it decreased the number of cells in the G0/G1 and S phases (, SD 2). Moreover, the sub-G1 population (26.6% at 1 mM) increased at 48 h of ethylparaben treatment in a dose-dependent manner (). These results suggest that ethylparaben treatment increases the sub-G1 population in BeWo cells.
Figure 2. Changes in BeWo cell cycle upon treatment with ethylparaben. BeWo cells were incubated with 0.1–10 mM of ethylparaben for 24 or 48 h. Then, cells were fixed with 70% EtOH for 1 h at 4°C for flow cytometry analysis. The cell cycle was analyzed using FACS using propidium iodide (PI) staining, as described in Materials and methods (SD 2). Data are presented as the percentage of cells in the sub-G1, G0/G1, S, and G2/M phases and are presented as mean ± S.E.M. of three independent experiments (n = 6) measured in triplicate.
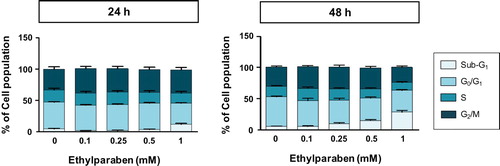
Ethylparaben changed the expression levels of Cyclin B1 and Cyclin D1
To further examine the molecular mechanisms underlying cell cycle arrest at the sub-G1 phase by ethylparaben, the expression patterns of cell cycle markers were verified using flow cytometry analysis. Cells were treated with different concentrations (0.1–1 mM) of ethylparaben at different time points. After immunostaining with anti-Cyclin B1 antibody, Cyclin B1 expressing cells were counted using FACS analysis. The Cyclin B1 positive population representing the G0/G1 phase was significantly increased by ethylparaben treatment in a dose-dependent manner (). In addition, the expression of Cyclin D1, denoting G2/M phase progression was also analyzed after ethylparaben treatment. Ethylparaben reduced the Cyclin D1-positive population as in 0.5% at 48 h in BeWo cells (). These results showed that the cell cycle was arrested at the sub-G1 phase by ethylparaben treatment.
Figure 3. Expression of Cyclin B1 was increased upon ethylparaben treatment in BeWo cells. To assess the effect of ethylparaben treatment on the protein expression level of Cyclin B1, cells were treated with 0.1–1 mM of ethylparaben for 24 or 48 h. Cells were, then, fixed with 1% PFA and were stained with an antibody against Cyclin B1 (upper panel). Data are expressed as the percentage of Cyclin B1 positive cells (lower panel). Data represent the mean ± S.E.M. of three independent experiments (n = 6) measured in triplicate (***, p < .001 compared with cells treated with 0.6% EtOH as a negative control).
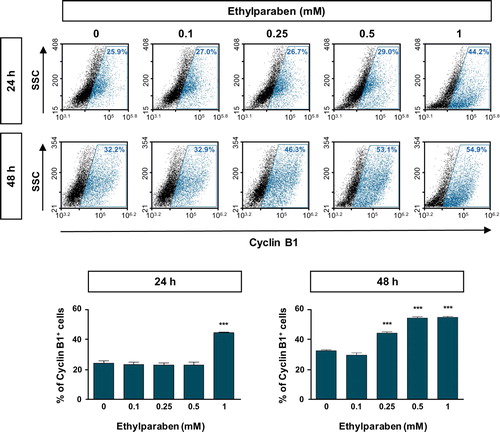
Figure 4. Ethylparaben treatment reduced the expression of Cyclin D1 protein in BeWo cells. The effect of ethylparaben treatment on the protein expression level of Cyclin D1 was analyzed using flow cytometry. BeWo cells were treated with 0.1–1 mM of ethylparaben for 24 or 48 h. Cells were fixed with 1% PFA and were stained with the antibody against Cyclin D1 (upper panel). Data are expressed as the percentage of Cyclin D1 positive cells (lower panel). Data represent the mean ± S.E.M. of three independent experiments (n = 6) measured in triplicate (*, p < .05; **, p < .01; ***, p < .001 compared with cells treated with 0.6% EtOH as a negative control).
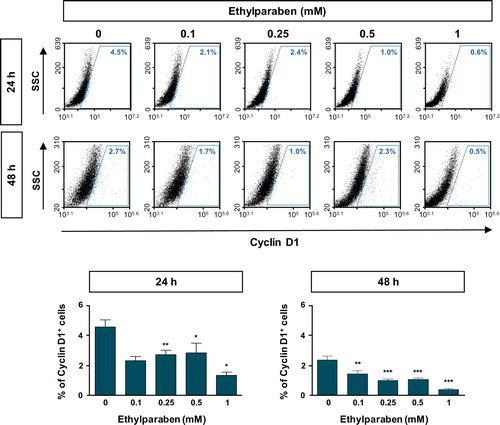
Ethylparaben induced apoptosis of BeWo cells through the Caspase-3 pathway
To investigate whether ethylparaben causes apoptosis in human placenta cells, BeWo cells were treated with ethylparaben in a dose- and time-dependent manner. After incubation for the indicated time periods, cells were stained with Annexin-V and PI to detect apoptotic cells using flow cytometry analysis. Ethylparaben treatment dramatically increased the number of apoptotic cells in a dose-dependent manner (). The number of Annexin-V positive cells was 4.8-fold greater after 1 mM ethylparaben treatment for 24 h than in the untreated control group. Annexin-V positive and PI negative populations representing early apoptosis also increased slightly after 1 mM ethylparaben treatment for 48 h (12.1%). These results showed that ethylparaben induces apoptotic cell death in BeWo cells. To further examine the mechanisms underlying apoptotic cell death induced by ethylparaben, we assessed the effect of ethylparaben on Caspase-3 activity using flow cytometry analysis. The active form of Caspase-3 was measured by immunostaining with anti-cleaved Caspase-3 protein. Ethylparaben significantly increased the cleaved Caspase-3 levels in BeWo cells in a dose- and time-dependent manner. After ethylparaben treatment, Caspase-3 activation was enhanced by approximately 2- to 3- fold at 48 h compared to that at 24 h, in BeWo cells ().
Figure 5. Ethylparaben treatment induced apoptotic cell death in BeWo cells. Cells were incubated with 0.1–1 mM of ethylparaben for 24 or 48 h. Then, cells were stained with Annexin V-FITC and propidium iodide (PI). The proportion of apoptotic cells was assessed using flow cytometry analysis (upper panel). The scatter plots represent PI (y-axis) vs. Annexin V-FITC (x-axis) stained cells. Values express the percentage of cells at the live, early-, late-apoptosis and necrosis stages and represent the mean ± S.E.M. of three independent experiments (n = 6) measured in triplicate (lower panel).
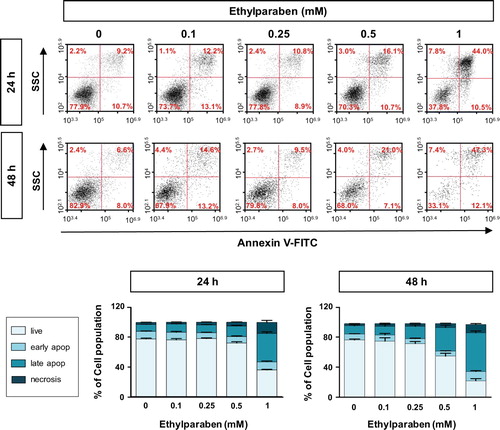
Figure 6. Cleaved Caspase-3 level was increased by ethylparaben treatment in BeWo cells. Functional Caspase-3 level after treatment with ethylparaben was analyzed by the detection of cleaved forms of Caspase-3 using flow cytometry. After treatment with 0.1–1 mM of ethylparaben for 24 or 48 h, BeWo cells were fixed with 1% PFA and stained with the antibody against cleaved-Caspase-3 (c-Caspase-3; upper panel). Data are expressed as the percentage of c-Caspase-3 positive cells (lower panel). Data represent the mean ± S.E.M. of three independent experiments (n = 6) measured in triplicate (***, p < .001 compared with cells treated with 0.6% EtOH as a negative control).
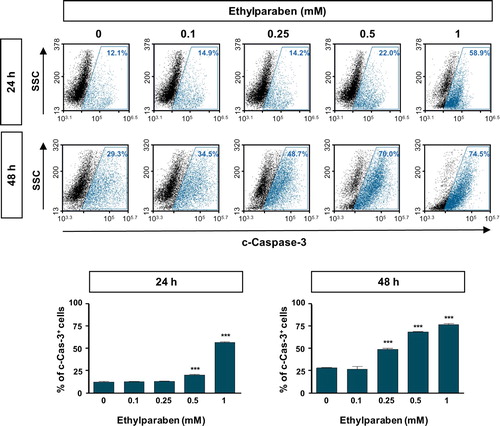
Discussion
Exposure of humans to parabens is mostly through preservatives in commercial products, as well as fruits and vegetables containing naturally occurring parabens (Soni et al. Citation2005; Bledzka et al. Citation2014). However, there have been extensive studies to assess the adverse effects on human health caused by the increasing frequency of exposure to parabens. Recent studies have focused on the potential effects of paraben exposure during the prenatal and neonatal periods in humans. The aim of this study was to evaluate the cytotoxicity of ethylparaben for human placental cells in an in vitro system.
Here, we demonstrated that ethylparaben inhibits the viability of human placental BeWo cells by inducing apoptotic cell death. To determine the harmful effect of ethylparaben on BeWo cells, we first examined cell viability using established in vitro tests, such as the MTS and live/dead assays, which assess the metabolic activity of ethylparaben-treated cells. Both assays indicated a gradual suppression of cell growth upon treatment with ethylparaben in a dose- and time-dependent manner (). Recently, a report demonstrated the genotoxic effects of ethylparaben in the mouse lymphoma cell line L5178Y, the human lymphoblastoid cell line TK6, and human primary lymphocytes (Finot et al. Citation2017). In addition, it has been demonstrated that exposure to ethylparaben during early pregnancy may enhance the risk of gestational diabetes mellitus (Liu et al. Citation2019). To further investigate the molecular mechanisms underlying the inhibition of cell growth induced by ethylparaben treatment, the cell cycle of BeWo cells was analyzed using PI staining. We verified that ethylparaben treatment at the indicated time points induced cell cycle arrest in a dose-dependent manner (). We found that ethylparaben treatment induces a sub-G1 phase arrest of the cell cycle in human placental BeWo cells. This may have been caused by the accumulation of Cyclin B1 and the reduction in Cyclin D1 due to ethylparaben ( and ). Previous studies have demonstrated that different types of parabens induce cell death in different cell types (Perez Martin et al. Citation2010; Dubey et al. Citation2017; Yang et al. Citation2018). However, there is no report regarding the adverse effect of ethylparaben on cellular lifespan. In the present study, we showed that ethylparaben induces apoptotic cell death by promoting the activation of Caspase-3 in BeWo cells ( and ). To the best of our knowledge, this study is the first to identify the effect of ethylparaben on cell death in human placenta cells.
Ethylparaben is officially considered as safe to use in commercial products, and recent reports have shown that the number of products using ethylparaben at low concentrations has increased (Andersen Citation2008). However, discussions on the safety of parabens have only been underway over the past two decades. The potential effect of parabens on developing fetuses has also been debated (Kolatorova et al. Citation2017). Collectively, the results of the present study demonstrated that ethylparaben has harmful effects on human placental BeWo cells via the dysregulation of cell cycle progression and induces cell apoptosis by the activation of Caspase-3. These results indicate the adverse influence of parabens exposure of the mother on a fetus, and suggest that exposure of pregnant females to ethylparaben is a cause for concern.
Acknowledgements
The authors would like to thank Editage (www.editage.co.kr) for English language editing.
Disclosure statement
No potential conflict of interest was reported by the authors.
Correction Statement
This article has been republished with minor changes. These changes do not impact the academic content of the article.
Additional information
Funding
References
- Andersen A. 2008. Final amended report on the safety assessment of metylparaben, ethylparaben, propylparaben, isopropylparaben, butylparaben, isobutylparaben, and benzylparaben as used in cosmentic products. Int J Toxicol. 27(4):1–82.
- Azzouz A, Rascon AJ, Ballesteros E. 2016. Simultaneous determination of parabens, alkylphenols, phenylphenols, bisphenol A and triclosan in human urine, blood and breast milk by continuous solid-phase extraction and gas chromatography-mass spectrometry. J Pharm Biomed Anal. 119:16–26. doi: 10.1016/j.jpba.2015.11.024
- Bledzka D, Gromadzinska J, Wasowicz W. 2014. Parabens. from environmental studies to human health. Environ Int. 67:27–42. doi: 10.1016/j.envint.2014.02.007
- Darbre PD, Aljarrah A, Miller WR, Coldham NG, Sauer MJ, Pope GS. 2004. Concentrations of parabens in human breast tumours. J Appl Toxicol. 24:5–13. doi: 10.1002/jat.958
- Darbre PD, Harvey PW. 2008. Paraben esters: review of recent studies of endocrine toxicity, absorption, esterase and human exposure, and discussion of potential human health risks. J Appl Toxicol. 28:561–578. doi: 10.1002/jat.1358
- Dubey D, Chopra D, Singh J, Srivastav AK, Kumari S, Verma A, Ray RS. 2017. Photosensitized methyl paraben induces apoptosis via caspase dependent pathway under ambient UVB exposure in human skin cells. Food Chem Toxicol. 108:171–185. doi: 10.1016/j.fct.2017.07.056
- Finot F, Kaddour A, Morat L, Mouche I, Zaguia N, Cuceu C, Souverville D, Negrault S, Cariou O, Essahli A, et al. 2017. Genotoxic risk of ethyl-paraben could be related to telomere shortening. J Appl Toxicol. 37:758–771. doi: 10.1002/jat.3425
- Golden R, Gandy J, Vollmer G. 2008. A review of the endocrine activity of parabens and implications for potential risks to human health. Crit Rev Toxicol. 35:435–458. doi: 10.1080/10408440490920104
- Janjua NR, Frederiksen H, Skakkebaek NE, Wulf HC, Andersson AM. 2008. Urinary excretion of phthalates and paraben after repeated whole-body topical application in humans. Int J Androl. 31:118–130. doi: 10.1111/j.1365-2605.2007.00841.x
- Kang S, Kim S, Park J, Kim HJ, Lee J, Choi G, Choi S, Kim S, Kim SY, Moon HB, et al. 2013. Urinary paraben concentrations among pregnant women and their matching newborn infants of Korea, and the association with oxidative stress biomarkers. Sci Total Environ. 461–462:214–221. doi: 10.1016/j.scitotenv.2013.04.097
- Kolatorova L, Duskova M, Vitku J, Starka L. 2017. Prenatal exposure to bisphenols and parabens and impacts on human physiology. Physiol Res. 66:S305–S315. doi: 10.33549/physiolres.933723
- Liu W, Zhou Y, Li J, Sun X, Liu H, Jiang Y, Peng Y, Zhao H, Xia W, Li Y, et al. 2019. Parabens exposure in early pregnancy and gestational diabetes mellitus. Environ Int. 126:468–475. doi: 10.1016/j.envint.2019.02.040
- Nishizawa C, Takeshita K, Ueda J, Nakanishi I, Suzuki KT, Ozawa T. 2006. Reaction of para-hydroxybenzoic acid esters with singlet oxygen in the presence of glutathione produces glutathione conjugates of hydroquinone, potent inducers of oxidative stress. Free Radic Res. 40:233–240. doi: 10.1080/10715760500485036
- Okubo T, Yokoyama Y, Kano K, Kano I. 2001. ER-dependent estrogenic activity of parabens assessed by proliferation of human breast cancer MCF-7 cells and expression of ERa and PR. Food Chem Toxicol. 39:1225–1232. doi: 10.1016/S0278-6915(01)00073-4
- Pedersen KL, Pedersen SN, Christiansen LB, Korsgaard B, Bjerregaard P. 2000. The preservatives ethyl-, propyl- and butylparaben are oestrogenic in an in vivo fish assay. Pharmacol Toxicol. 86:110–113. doi: 10.1034/j.1600-0773.2000.d01-20.x
- Perez Martin JM, Peropadre A, Herrero O, Fernandez Freire P, Labrador V, Hazen MJ. 2010. Oxidative DNA damage contributes to the toxic activity of propylparaben in mammalian cells. Mutat Res. 702:86–91. doi: 10.1016/j.mrgentox.2010.07.012
- Pycke BF, Geer LA, Dalloul M, Abulafia O, Halden RU. 2015. Maternal and fetal exposure to parabens in a multiethnic urban U.S. population. Environ Int. 84:193–200. doi: 10.1016/j.envint.2015.08.012
- SCCS. 2013. Opinion on parabens: updated request for a scientific opinion on propyl- and butylparaben. (COLIPA n° P82).
- Smith KW, Souter I, Dimitriadis I, Ehrlich S, Williams PL, Calafat AM, Hauser R. 2013. Urinary paraben concentrations and ovarian aging among women from a fertility center. Environ Health Perspect. 121:1299–1305. doi: 10.1289/ehp.1205350
- Soni MG, Carabin IG, Burdock GA. 2005. Safety assessment of esters of p-hydroxybenzoic acid (parabens). Food Chem Toxicol. 43:985–1015. doi: 10.1016/j.fct.2005.01.020
- Sun X, Kaufman PD. 2018. Ki-67: more than a proliferation marker. Chromosoma. 127:175–186. doi: 10.1007/s00412-018-0659-8
- Taxvig C, Vinggaard AM, Hass U, Axelstad M, Boberg J, Hansen PR, Frederiksen H, Nellemann C. 2008. Do parabens have the ability to interfere with steroidogenesis? Toxicol Sci. 106:206–213. doi: 10.1093/toxsci/kfn148
- Vela-Soria F, Rodriguez I, Ballesteros O, Zafra-Gomez A, Ballesteros L, Cela R, Navalon A. 2014. Simplified matrix solid phase dispersion procedure for the determination of parabens and benzophenone-ultraviolet filters in human placental tissue samples. J Chromatogr A. 1371:39–47. doi: 10.1016/j.chroma.2014.10.063
- Yang C, Lim W, Bazer FW, Song G. 2018. Butyl paraben promotes apoptosis in human trophoblast cells through increased oxidative stress-induced endoplasmic reticulum stress. Environ Toxicol. 33:436–445. doi: 10.1002/tox.22529
- Ye X, Bishop AM, Reidy JA, Needham LL, Calafat AM. 2006. Parabens as urinary biomarkers of exposure in humans. Environ Health Perspect. 114:1843–1846. doi: 10.1289/ehp.9413