ABSTRACT
Cytoplasmic FMR1-interacting protein 2 (CYFIP2) is an evolutionarily conserved multifunctional protein that regulates the neuronal actin cytoskeleton, mRNA translation and transport, and mitochondrial morphology and function. Supporting its critical roles in proper neuronal development and function, human genetic studies have repeatedly identified variants of the CYFIP2 gene in individuals diagnosed with neurodevelopmental disorders. Notably, a few recent studies have also suggested a mechanistic link between reduced CYFIP2 level and Alzheimer's disease (AD). Specifically, in the hippocampus of 12-month-old Cyfip2 heterozygous mice, several AD-like pathologies were identified, including increased levels of Tau phosphorylation and gliosis, and loss of dendritic spines in CA1 pyramidal neurons. However, detailed pathogenic mechanisms, such as cell types and their circuits where the pathologies originate, remain unknown for AD-like pathologies caused by CYFIP2 reduction. In this study, we aimed to address this issue by examining whether the cell-autonomous reduction of CYFIP2 in CA1 excitatory pyramidal neurons is sufficient to induce AD-like phenotypes in the hippocampus. We performed immunohistochemical, morphological, and biochemical analyses in 12-month-old Cyfip2 conditional knock-out mice, which have postnatally reduced CYFIP2 expression level in CA1, but not in CA3, excitatory pyramidal neurons of the hippocampus. Unexpectedly, we could not find any significant AD-like phenotype, suggesting that the CA1 excitatory neuron-specific reduction of CYFIP2 level is insufficient to lead to AD-like pathologies in the hippocampus. Therefore, we propose that CYFIP2 reduction in other neurons and/or their synaptic connections with CA1 pyramidal neurons may be critically involved in the hippocampal AD-like phenotypes of Cyfip2 heterozygous mice.
Introduction
The two members of the cytoplasmic FMR1-interacting protein family, CYFIP1 and CYFIP2, are evolutionarily conserved proteins whose genetic variants are causally associated with numerous brain disorders, including autism spectrum disorders, intellectual disability, schizophrenia, and epilepsy (Schenck et al. Citation2001; Abekhoukh and Bardoni Citation2014; Zhang, Lee, et al. Citation2019). Specifically, in the case of CYFIP2, de novo variants have recently been identified in individuals diagnosed with neurodevelopmental disorders and early-onset epileptic encephalopathy characterized by developmental regression, intellectual disability, seizures, muscular hypotonia, and microcephaly (Nakashima et al. Citation2018; Peng et al. Citation2018; Lee et al. Citation2019; Zhong et al. Citation2019; Zweier et al. Citation2019; Begemann et al. Citation2021; Kang et al. Citation2023).
At the molecular level, CYFIP1 and CYFIP2 have a high amino acid sequence homology and both are involved in the regulation of cellular actin cytoskeleton dynamics as a critical component of the heteropentameric Wiskott–Aldrich syndrome protein family verprolin-homologous protein (WAVE) regulatory complex (WRC) (Lee Y et al. Citation2017; Rottner et al. Citation2021). Additional functions in neurons, such as regulation of mRNA translation and transport (Napoli et al. Citation2008; De Rubeis et al. Citation2013; Cioni et al. Citation2018), and regulation of mitochondrial function and morphology (Kanellopoulos et al. Citation2020; Kim GH et al. Citation2020) have also been identified, but unlike actin regulation via the WRC, these functions may be mediated by both shared and distinct interactors of CYFIP1 and CYFIP2, respectively (Lee Y et al. Citation2020; Ma et al. Citation2022). Collectively, these molecular functions of CYFIP1 and CYFIP2 can contribute to the morphological and functional changes in neuronal synapses observed in both Cyfip1 and Cyfip2 mutant mice (Bozdagi et al. Citation2012; Pathania et al. Citation2014; Han et al. Citation2015; Davenport et al. Citation2019; Lee SH et al. Citation2020; Zhang et al. Citation2020; Kim NS et al. Citation2022).
Moreover, several lines of evidence indicate the differential roles of CYFIP1 and CYFIP2 in vivo, including the embryonic and perinatal lethality of Cyfip1-null mice and Cyfip2-null mice, respectively (Chung et al. Citation2015; Han et al. Citation2015; Zhang et al. Citation2019a), and different brain regional and cell-type distributions of CYFIP1 and CYFIP2 mRNAs and proteins (Zhang, Kang, et al. Citation2019b; Ma et al. Citation2022). In particular, CYFIP1, but not CYFIP2, is also expressed in non-neuronal cells, such as astrocytes, microglia, and oligodendrocytes, in the brain (Dominguez-Iturza et al. Citation2019; Silva et al. Citation2019; Habela et al. Citation2020; Haan et al. Citation2021; Ma et al. Citation2022).
Beyond the genetic association between CYFIP2 and neurodevelopmental disorders, recent studies have suggested a mechanistic association between the reduction of CYFIP2 and Alzheimer's disease (AD) (Tiwari et al. Citation2016; Ghosh et al. Citation2020). Specifically, CYFIP2 protein levels were reduced in the postmortem forebrain of patients with AD and in the hippocampus and cortex of AD model mice (Tiwari et al. Citation2016). Moreover, the protein levels of amyloid precursor protein (APP), β-site APP cleaving enzyme 1 (BACE1), and calcium/calmodulin-dependent protein kinase IIα (CaMKIIα) were up-regulated in the hippocampal synaptosomal fraction of conventional Cyfip2 heterozygous (Cyfip2+/–, het) mice. Consistently, in the hippocampus of aged (12-month-old) Cyfip2 het mice, several AD-like pathologies have been observed, including increased levels of Tau phosphorylation and gliosis, and significant loss of dendritic spines in CA1 pyramidal neurons (Ghosh et al. Citation2020). Mechanistically, it has been proposed that reduced expression level of CYFIP2 induces aberrant local mRNA translation of several AD-related proteins (i.e. APP, BACE1, and CaMKIIα) at the synaptic compartment, thereby leading to the overproduction of Aβ and hyperphosphorylation of Tau in Cyfip2 het mice (Ghosh et al. Citation2020). Under normal conditions, CYFIP2, as shown for CYFIP1 (Napoli et al. Citation2008; De Rubeis et al. Citation2013), may repress the translation of these mRNAs by forming an inhibitory complex with the RNA-binding protein fragile X messenger ribonucleoprotein (FMRP) and the eukaryotic initiation factor 4E (eIF4E).
However, compared to CYFIP2 function and dysfunction in the developing brain, its role in AD-like pathologies in aged mice remains largely unknown. In particular, considering the complex interactions between different neuronal and non-neuronal cell types and the trans-synaptic spread of pathology in AD (Tzioras et al. Citation2023), investigating the cell types and their circuits where CYFIP2-dependent pathologies originate is a critical step toward understanding the pathogenic mechanisms. In this study, we aimed to address this issue by examining the AD-like phenotypes of aged (12-month-old) Cyfip2 conditional knock-out (cKO) mice, which have postnatally reduced CYFIP2 expression level selectively in CA1, but not in CA3, excitatory pyramidal neurons of the hippocampus. Surprisingly, unlike Cyfip2 het mice, there was no overt AD-like phenotype in the hippocampal CA1 region of aged Cyfip2 cKO mice. Therefore, our results suggest that cell-autonomous reduction of CYFIP2 is insufficient for AD-like pathologies in CA1 pyramidal neurons and that other neurons and/or their synaptic connections with CA1 pyramidal neurons are also critically involved in the hippocampal AD-like phenotypes of Cyfip2 het mice.
Materials and methods
Mice
The Cyfip2 cKO mice and Thy1-YFP mice used in this study were previously described (Lee SH et al. Citation2020; Zhang et al. Citation2020). The mice were fed ad libitum and housed under a 12 h light–dark cycle. All experiments were performed using aged (12-month-old) male Cyfip2 cKO mice and their littermate controls.
Fluorescence immunohistochemistry
Fluorescence immunohistochemistry was performed as previously described (Lee B et al. Citation2017; Yu et al. Citation2021). Mice were anesthetized with isoflurane and transcardially perfused with heparinized (20 units/mL) phosphate-buffered saline (PBS), followed by 4% paraformaldehyde (PFA) in PBS. The brains were extracted and post-fixed overnight in 4% PFA. Following post-fixation, brain tissue was washed with PBS and cryoprotected with 30% sucrose in PBS for 48 h. The brain tissues were frozen in an O.C.T compound (SAKURA Tissue-Tek, 4583) and sectioned (60 µm) using a cryostat microtome (Leica, CM3050S). The primary antibodies used for immunohistochemistry were AT-8 (Phospho-Tau [Ser202, Thr205], Invitrogen, #MN1020), CYFIP1 (Sigma-Aldrich, #AB6046), CYFIP2 (Abcam, #ab95969), GFAP (Abcam, #ab4674), Iba1 (Synaptic System, #234-006), NeuN (Abcam, #ab177487; Millipore, #MAB377). F-actin was visualized by Alexa Fluor 488-conjugated Phalloidin (Invitrogen, #A-12379). The samples were washed with 0.1% Triton X-100 in PBS and blocked with PBS containing 3% bovine serum albumin (BSA) and 0.5% Triton X-100. The high-resolution image acquisition was performed using a Zeiss LSM800 confocal microscope equipped with a 20×/0.8 objective lens, 8-bit image depth, and snapshot mode focused on maximum intensity. Whole brain regions were obtained using a slide scanner (Zeiss Axio Scan.Z1). The regions of the stratum oriens (SO) and stratum radiatum (SR) were defined as 100 µm and 100–200 µm away from the cell body area (stratum pyramidale, SP) of CA1, respectively. The values of at least two brain sections were averaged for each mouse.
Dendritic spine analysis
The dendritic spine analysis was performed as previously described (Han et al. Citation2013; Choi et al. Citation2015; Hong et al. Citation2022). Mice were deeply anesthetized with isoflurane and transcardially perfused with heparinized (20 units/mL) PBS followed by 4% PFA in PBS. The brains were extracted and post-fixed overnight in 4% PFA. After post-fixation, coronal sections (100 µm thickness) of the hippocampal region were obtained using a vibratome (VT1000S, Leica). The sections were collected and stored in 50% glycerol in 2 × PBS at −20◦C until further processed. Blocking, permeabilization, and anti-GFP (Abcam, #AB13970) primary and Alexa Fluorconjugated (anti-chicken Alexa Fluor-488, Jackson ImmunoResearch Labs, #703-545-155) secondary antibody incubation were performed as described above. Finally, the sections were mounted on slide glasses with mounting media (Biomeda, M02). Images of dendritic spines in the secondary or tertiary branches (apical or basal dendrites of YFP-positive CA1 pyramidal neurons in the hippocampus) were acquired by confocal microscopy (Zeiss LSM800) using 63×/1.2 water immersion objective lens, 8-bit image depth, and Z-stack function with 0.93 µm intervals, followed by Z-stack projection of maximum intensity. Images were analyzed using ImageJ software. For quantification of dendritic spines, mushroom spines were defined as protrusions with heads and with a width greater than length. Stubby spines were defined as protrusions without a neck. The rest of the protrusions with heads were categorized as thin spines. The values of six to eight neurons were averaged for each mouse.
Additional information of Materials and Methods is included in supporting online material.
Results
CA1 excitatory pyramidal neuron-specific reduction of CYFIP2 in the hippocampus of aged Cyfip2 cKO mice
To investigate whether cell-autonomous CYFIP2 reduction in excitatory pyramidal neurons is sufficient to induce AD-like phenotypes in the hippocampal CA1 region, we crossed floxed-Cyfip2 mice with CaMKIIα-Cre mice to generate Cyfip2 cKO (Cyfip2floxed/floxed; CaMKIIα-Cre) mice as previously described (Zhang et al. Citation2020). The CaMKIIα-Cre line (T29-1) used in this study starts expressing Cre recombinases in forebrain excitatory neurons during the third to fourth postnatal weeks, and especially in the hippocampus, Cre expression is restricted mainly to the CA1 region (Tsien et al. Citation1996). Notably, to securely obtain control (Cyfip2floxed/floxed) and Cyfip2 cKO progeny mice, we crossed male Cyfip2floxed/floxed mice with female Cyfip2floxed/floxed;CaMKIIα-Cre mice ((A)) to avoid unwanted germline recombination, which was recently reported in male, but not female, T29-1 mice (Luo et al. Citation2020). Furthermore, we designed an additional primer set for genotyping PCR to detect germline deletion of floxed exon 6 of the Cyfip2 gene (Lee SH et al. Citation2020), which indeed produced an expected PCR band from some portions of progeny mice (i.e. Cyfip2floxed/Δexon6 mice) when we crossed male Cyfip2floxed/floxed;CaMKIIα-Cre mice with female Cyfip2floxed/floxed mice as a test ((B) and (C)). Using this primer set, we confirmed that the control and Cyfip2 cKO mice used in this study did not have a germline deletion of the floxed Cyfip2 exon 6.
Figure 1. No AD-like immunohistological phenotype in the hippocampal CA1 region of aged Cyfip2 cKO mice. (A) The breeding scheme for the control (Cyfip2f/f) and Cyfip2 conditional knock-out (Cyfip2f/f;CaMKIIα-Cre, cKO) mice. (B) The breeding scheme to test the partial germline recombination of male CaMKIIα-Cre mice. (C) Design of primers to detect floxed and Δexon6 Cyfip2 alleles (left panel). Results of PCR for the tail genomic DNA isolated from Cyfip2f/f and Cyfip2floxed/Δexon6 mice (right panel). Note that the primer set (a + c) does not produce the expected ∼1.1 kbp band from the DNA sample of Cyfip2f/f mice due to the short elongation time of the PCR. (D) Fluorescence immunohistochemistry images and quantification showing CA1-specific reduction of CYFIP2 in the hippocampus of aged Cyfip2 cKO mice. CA, cornu ammonis; DG, dentate gyrus; NS, not significant; SO, stratum oriens; SP, stratum pyramidale; SR, stratum radiatum. (E, F) Normal CYFIP1 and phospho-Tau (AT-8) levels in the hippocampal CA1 region of aged Cyfip2 cKO mice. (G, H) Normal density and total intensity of astrocytes (GFAP-positive) and microglia (Iba1-positive) in the hippocampal CA1 region of aged Cyfip2 cKO mice. N = 7–8 mice.
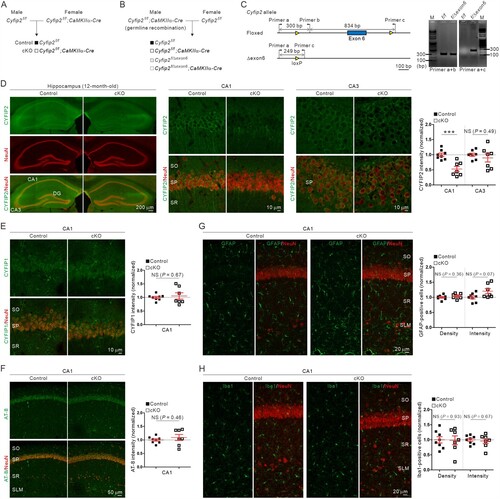
Fluorescence immunohistochemical analysis showed that CYFIP2 protein levels were selectively reduced in the hippocampal CA1, but not CA3, of 12-month-old Cyfip2 cKO mice ((D)), which was expected from CA1-restricted Cre expression in T29-1 mice. Meanwhile, CYFIP1 protein levels in the CA1 region were comparable between control and Cyfip2 cKO mice, suggesting that there is no compensatory increase in CYFIP1 level in the hippocampus of aged Cyfip2 cKO mice ((E)).
No AD-like immunohistological phenotype in the hippocampal CA1 region of aged Cyfip2 cKO mice
A previous study showed that several AD-like immunohistological phenotypes were significantly exacerbated in the hippocampal CA1 region of 12-month-old Cyfip2 het mice compared with age-matched wild-type (WT) mice (Ghosh et al. Citation2020). These phenotypes include increased levels of phospho-Tau immunoreactivity (measured by monoclonal AT-8 antibody) and gliosis (both for astrocytes and microglia, as measured by glial fibrillary acidic protein [GFAP] and ionized calcium-binding adapter molecule 1 [Iba1] antibodies, respectively). Therefore, we performed fluorescence immunohistochemical analyses of these AD markers in the hippocampal CA1 region of 12-month-old Cyfip2 cKO mice and their littermate controls. However, there were no significant differences in phospho-Tau levels between control and Cyfip2 cKO mice ((F)). Moreover, neither GFAP nor Iba1 positive cell number or total intensity was significantly altered in Cyfip2 cKO mice compared to control mice ((G) and (H)).
Reduced number of immature dendritic spines and increased F-actin levels in the basal dendrites of CA1 pyramidal neurons of aged Cyfip2 cKO mice
Dendritic spines are small dendritic protrusions that represent the most excitatory postsynapses in the brain (Penzes et al. Citation2011). Loss of dendritic spines is another key feature of AD (Dorostkar et al. Citation2015) and was significantly aggravated in CA1 pyramidal neurons of 12-month-old Cyfip2 het mice compared to age-matched WT mice (Ghosh et al. Citation2020). In particular, the number of mature-type mushroom spines, but not that of immature-type thin spines, was reduced in the apical dendrites of CA1 pyramidal neurons in aged Cyfip2 het mice. Therefore, we analyzed the dendritic spines of CA1 pyramidal neurons in 12-month-old control and Cyfip2 cKO mice. Dendritic spines were visualized by crossing Cyfip2 cKO mice with Thy1-YFP mice (Feng et al. Citation2000) that sparsely express yellow fluorescent protein (YFP) in CA1 pyramidal neurons ((A)). We separately analyzed the basal and apical dendrites of the neurons ((B)). In the basal dendrites, we found that the total number of dendritic spines was significantly reduced in Cyfip2 cKO neurons compared to control neurons ((C)). However, unlike the mushroom spine-specific reduction in Cyfip2 het mice (Ghosh et al. Citation2020), the spine reduction in Cyfip2 cKO mice was mainly attributed to a decrease in immature-type thin spines. Moreover, in the apical dendrites, neither total density nor morphologically-based categorization of dendritic spines was altered in Cyfip2 cKO neurons compared to control neurons ((C)), suggesting that there is no AD-like dendritic spine phenotype in CA1 pyramidal neurons of aged Cyfip2 cKO mice. We also compared head size for both thin and mushroom spines between the control and Cyfip2 cKO neurons and found no significant differences in the basal or apical dendrites ((D)).
Figure 2. Dendritic spine and F-actin changes in the hippocampal CA1 region of aged Cyfip2 cKO mice. (A) Visualization of CA1 pyramidal neurons in Thy1-YFP mice by sparse expression of yellow fluorescent protein (YFP). CA, cornu ammonis; DG, dentate gyrus; SO, stratum oriens; SP, stratum pyramidale; SR, stratum radiatum. (B) Representative confocal images of dendritic spines in the basal and apical dendrites of CA1 pyramidal neurons of aged control and Cyfip2 cKO mice. Examples of dendritic spines in each morphologically-based categorization (thin, stubby, and mushroom) are indicated by arrows with different colors. (C) Quantification of dendritic spine number in the basal (upper panel) and apical (lower panel) dendrites. NS, not significant. (D) Quantification of dendritic spine head size in basal (upper panel) and apical (lower panel) dendrites. (E) Representative confocal images and quantification of F-actin levels in the hippocampal CA1 region of aged control and Cyfip2 cKO mice. N = 4–8 mice.
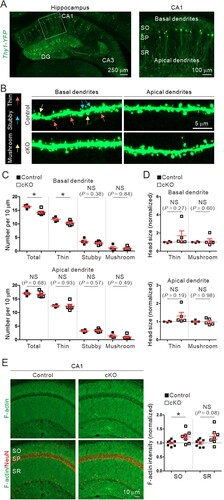
F-actin is a key cytoskeletal component of dendritic spines and is directly associated with their formation, maintenance, and dynamics (Spence and Soderling Citation2015). As a critical component of the WRC, CYFIP2 is involved in actin regulation in various cellular compartments, including neuronal dendritic spines (Rottner et al. Citation2021). Specifically, it has been previously shown increased F-actin levels in the medial prefrontal cortex (mPFC) of young adult Cyfip2 het and Cyfip2 cKO mice (Lee SH et al. Citation2020; Zhang et al. Citation2020). Therefore, we also measured F-actin levels in the hippocampal CA1 region and found an increase in the stratum oriens (SO), but not in the stratum radiatum (SR), of aged Cyfip2 cKO mice compared to control mice ((E)).
Normal expression of synaptosomal APP and CaMKIIα in the hippocampal CA1 region of aged Cyfip2 cKO mice
Overexpression of AD-related proteins, such as APP and CaMKIIα, in the synaptic compartment due to aberrant local mRNA translation, has been proposed as a molecular mechanism underlying AD-like pathologies in Cyfip2 het mice (Tiwari et al. Citation2016; Ghosh et al. Citation2020). Therefore, we analyzed the protein levels of APP and CaMKIIα in the hippocampus of 12-month-old control and Cyfip2 cKO mice. We prepared a crude synaptosomal fraction from the dissected hippocampal CA1 region and performed immunoblotting ((A)). Consistent with the immunohistological analysis, CYFIP2 levels were reduced in hippocampal CA1 synaptosomal lysates from Cyfip2 cKO mice compared to control mice ((B)). As expected, the WAVE1 protein, another component of the WRC, was also reduced in Cyfip2 cKO lysates because the stability of WAVE1 is inter-dependent with that of CYFIP2 (Han et al. Citation2015; Zhang et al. Citation2020; Kang et al. Citation2023). In contrast, CYFIP1 levels were comparable between control and Cyfip2 cKO mice. Notably, we found that neither APP nor CaMKIIα protein levels were significantly altered in Cyfip2 cKO mice compared to control mice ((B)). Furthermore, the levels of PSD-95, another synaptic protein whose mRNA stability and translation are regulated by FMRP (Zalfa et al. Citation2007), and FMRP itself were normal in the CA1 synaptosome of aged Cyfip2 cKO mice. Taken together, these results suggest that there is no overt AD-like pathology in the hippocampal CA1 region of 12-month-old Cyfip2 cKO mice.
Figure 3. Normal synaptosomal expression levels of AD-related proteins, APP and CaMKIIα, in the hippocampal CA1 region of aged Cyfip2 cKO mice. (A) Schematic diagram showing the dissection of the CA1 region of the mouse hippocampus. (B) Representative immunoblot images and quantification of the expression levels of CYFIP2, WAVE1, CYFIP1, APP, CaMKIIα, PSD-95, and FMRP proteins in CA1 synaptosomal fraction of aged Cyfip2 cKO mice compare to control mice. Protein levels were normalized by either a neuron-specific protein, neuron-specific enolase (NSE), or GAPDH. NS, not significant. N = 5 mice.
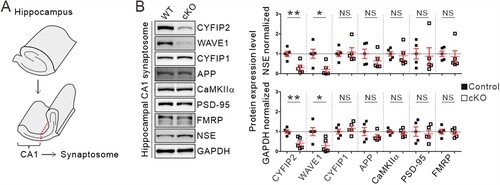
Discussion
In this study, we combined immunohistochemical, morphological, and biochemical approaches to understand whether the cell-autonomous reduction of CYFIP2 in excitatory pyramidal neurons is sufficient to induce AD-like pathologies in the hippocampal CA1 region. However, none of the results showed a significant AD-like phenotype in aged Cyfip2 cKO mice, in contrast to the severe phenotypes observed in Cyfip2 het mice (Tiwari et al. Citation2016; Ghosh et al. Citation2020). Therefore, our results suggest that other neurons and/or their synaptic connections with CA1 pyramidal neurons are also critically involved in the hippocampal AD-like phenotypes of Cyfip2 het mice. Additional genetic or viral tools to reduce CYFIP2 protein levels in specific or combinatorial neurons of the hippocampal circuit will help us further address this issue.
Based on previous findings, we speculate on some mechanisms that explain the lack of an AD-like phenotype in Cyfip2 cKO mice (). CYFIP2 mRNAs and proteins are predominantly expressed in neurons compared to non-neuronal cells in the brain and are detected in both excitatory and local inhibitory neurons (Zhang, Kang, et al. Citation2019b; Lee SH et al. Citation2020; Ma et al. Citation2022). Moreover, CYFIP2 is expressed in neurons of the hippocampal CA3 region as well as of other brain regions that can directly form synaptic connections with CA1 excitatory pyramidal neurons (Han et al. Citation2015; Lee SH et al. Citation2020). As CYFIP2 regulates axonal and presynaptic development and function (Cioni et al. Citation2018; Kim GH et al. Citation2020), it is conceivable that both presynaptic and postsynaptic compartments are functionally affected in CA1 pyramidal neurons of Cyfip2 het mice, thereby ultimately leading to synaptic loss. Meanwhile, the postsynapse-specific CYFIP2 reduction in CA1 pyramidal neurons of Cyfip2 cKO mice may be insufficient to induce such changes. Furthemore, considering the concept of trans-synaptic propagation of pathology in AD (Tzioras et al. Citation2023), bi-directional spread of AD pathology through synaptic connections with other abnormal neurons may synergistically worsen the phenotypes of CA1 pyramidal neurons of Cyfip2 het mice compared to those of Cyfip2 cKO mice.
Figure 4. Schematic diagrams summarizing the different hippocampal phenotypes among aged wild-type (WT), Cyfip2 het, and Cyfip2 cKO mice. CYFIP2 protein levels are reduced in both CA1 and CA3 excitatory and inhibitory neurons in Cyfip2 het mice, but they are only reduced in CA1 excitatory neurons in Cyfip2 cKO mice. In the hippocampal CA1 region, AD-like pathologies, such as gliosis and dendritic spine loss, are observed in Cyfip2 het mice. In contrast, no AD-like phenotype is observed in Cyfip2 cKO mice. Ex., excitatory; Inh., inhibitory.
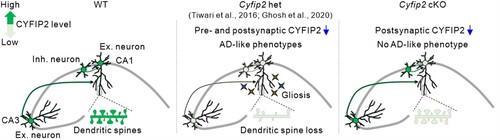
At the molecular level, local mRNA translation of AD-related proteins by the CYFIP2-FMRP-eIF4E complex can be differentially affected in the CA1 pyramidal neurons of Cyfip2 het and Cyfip2 cKO mice (Ghosh et al. Citation2020). Indeed, we observed normal synaptosomal levels of APP and CaMKIIα proteins in the hippocampal CA1 of Cyfip2 cKO mice, unlike their increased levels in Cyfip2 het mice (Tiwari et al. Citation2016). The interaction of CYFIP1 with FMRP and eIF4E is regulated by synaptic activity (De Rubeis et al. Citation2013). Therefore, it can be speculated that even with CYFIP2 reduction, relatively normal synaptic functions in neurons of Cyfip2 cKO mice could preserve local mRNA translation within the normal range. However, in Cyfip2 het mice, more profound changes in synaptic activity, due to both presynaptic and postsynaptic reduction of CYFIP2, and changes in the CYFIP2-FMRP-eIF4E complex may congruently lead to aberrant mRNA translation and overproduction of AD-related proteins. Further investigations of the molecular composition and function of the CYFIP2-FMRP-eIF4E complex in Cyfip2 het and Cyfip2 cKO neurons are needed to test this hypothesis.
Considering the aforementioned speculations regarding the mechanisms involved, another plausible explanation for the observed phenotypic difference between Cyfip2 het and Cyfip2 cKO CA1 pyramidal neurons could be the delayed onset of disease in Cyfip2 cKO mice compared to Cyfip2 het mice. Due to the preservation of normal presynaptic inputs in CA1 pyramidal neurons of Cyfip2 cKO mice, it is possible that the neurons will take a longer time to exhibit AD-like phenotypes compared to the neurons of Cyfip2 het mice. This hypothesis can be examined by investigating the hippocampus of older (e.g. 18-month-old) Cyfip2 cKO mice and age-matched control mice.
We observed a reduced number of thin, but normal stubby and mushroom spines in the basal dendrites of CA1 pyramidal neurons of aged Cyfip2 cKO mice. However, there was no significant change in the number of thin, stubby, and mushroom spines in the apical dendrites. The basal dendrite-specific decrease in dendritic spine number was also observed in the layer 5 neurons of the mPFC of young adult Cyfip2 cKO mice (Zhang et al. Citation2020), although the underlying mechanism remains unknown. Additionally, a previous study found that overexpression of CYFIP2 increases excitatory synapse number in cultured hippocampal neurons at 14 days in vitro (Davenport et al. Citation2019), suggesting that CYFIP2 dosage may be an important factor regulating the development and maintenance of excitatory synapses in hippocampal neurons. Our results also indicate that regulation of the F-actin dynamics via the WRC may be a key mechanism, as we observed changes in F-actin levels and downregulation of WAVE1 in the hippocampal CA1 region of Cyfip2 cKO mice.
In conclusion, our results suggest that excitatory neuron-specific reduction of CYFIP2 is insufficient to induce AD-like pathologies in the hippocampal CA1 region, warranting further investigation into the neuronal circuit-dependent mechanisms of AD pathology induced by CYFIP2 reduction. Beyond identifying the pathogenic mechanisms of AD, these investigations will potentially provide critical neuronal targets in the brain for novel therapeutic approaches to AD.
Supplemental Material
Download MS Word (18.7 KB)Disclosure statement
No potential conflict of interest was reported by the author(s).
Additional information
Funding
References
- Abekhoukh S, Bardoni B. 2014. CYFIP family proteins between autism and intellectual disability: links with fragile X syndrome. Front Cell Neurosci. 8:81.
- Begemann A, Sticht H, Begtrup A, Vitobello A, Faivre L, Banka S, Alhaddad B, Asadollahi R, Becker J, Bierhals T, et al. 2021. New insights into the clinical and molecular spectrum of the novel CYFIP2-related neurodevelopmental disorder and impairment of the WRC-mediated actin dynamics. Genet Med. 23(3):543–554.
- Bozdagi O, Sakurai T, Dorr N, Pilorge M, Takahashi N, Buxbaum JD. 2012. Haploinsufficiency of Cyfip1 produces fragile X-like phenotypes in mice. PLoS One. 7(8):e42422.
- Choi SY, Pang K, Kim JY, Ryu JR, Kang H, Liu Z, Kim WK, Sun W, Kim H, Han K. 2015. Post-transcriptional regulation of SHANK3 expression by microRNAs related to multiple neuropsychiatric disorders. Mol Brain. 8(1):74.
- Chung L, Wang X, Zhu L, Towers AJ, Cao X, Kim IH, Jiang YH. 2015. Parental origin impairment of synaptic functions and behaviors in cytoplasmic FMRP interacting protein 1 (Cyfip1) deficient mice. Brain Res. 1629:340–350.
- Cioni JM, Wong HH, Bressan D, Kodama L, Harris WA, Holt CE. 2018. Axon-Axon interactions regulate topographic optic tract sorting via CYFIP2-dependent WAVE complex function. Neuron. 97(5):1078–1093.e6.
- Davenport EC, Szulc BR, Drew J, Taylor J, Morgan T, Higgs NF, Lopez-Domenech G, Kittler JT. 2019. Autism and schizophrenia-associated CYFIP1 regulates the balance of synaptic excitation and inhibition. Cell Rep. 26(8):2037–2051.e6.
- De Rubeis S, Pasciuto E, Li KW, Fernandez E, Di Marino D, Buzzi A, Ostroff LE, Klann E, Zwartkruis FJ, Komiyama NH, et al. 2013. CYFIP1 coordinates mRNA translation and cytoskeleton remodeling to ensure proper dendritic spine formation. Neuron. 79(6):1169–1182.
- Dominguez-Iturza N, Lo AC, Shah D, Armendariz M, Vannelli A, Mercaldo V, Trusel M, Li KW, Gastaldo D, Santos AR, et al. 2019. The autism- and schizophrenia-associated protein CYFIP1 regulates bilateral brain connectivity and behaviour. Nat Commun. 10(1):3454.
- Dorostkar MM, Zou C, Blazquez-Llorca L, Herms J. 2015. Analyzing dendritic spine pathology in Alzheimer’s disease: problems and opportunities. Acta Neuropathol. 130(1):1–19.
- Feng G, Mellor RH, Bernstein M, Keller-Peck C, Nguyen QT, Wallace M, Nerbonne JM, Lichtman JW, Sanes JR. 2000. Imaging neuronal subsets in transgenic mice expressing multiple spectral variants of GFP. Neuron. 28(1):41–51.
- Ghosh A, Mizuno K, Tiwari SS, Proitsi P, Gomez Perez-Nievas B, Glennon E, Martinez-Nunez RT, Giese KP. 2020. Alzheimer’s disease-related dysregulation of mRNA translation causes key pathological features with ageing. Transl Psychiatry. 10(1):192.
- Haan N, Westacott LJ, Carter J, Owen MJ, Gray WP, Hall J, Wilkinson LS. 2021. Haploinsufficiency of the schizophrenia and autism risk gene Cyfip1 causes abnormal postnatal hippocampal neurogenesis through microglial and Arp2/3 mediated actin dependent mechanisms. Transl Psychiatry. 11(1):313.
- Habela CW, Yoon KJ, Kim NS, Taga A, Bell K, Bergles DE, Maragakis NJ, Ming GL, Song H. 2020. Persistent Cyfip1 expression is required to maintain the adult subventricular zone neurogenic niche. J Neurosci. 40(10):2015–2024.
- Han K, Chen H, Gennarino VA, Richman R, Lu HC, Zoghbi HY. 2015. Fragile X-like behaviors and abnormal cortical dendritic spines in cytoplasmic FMR1-interacting protein 2-mutant mice. Hum Mol Genet. 24(7):1813–1823.
- Han K, Holder JL, Schaaf CP, Lu H, Chen H, Kang H, Tang J, Wu Z, Hao S, Cheung SW, et al. 2013. SHANK3 overexpression causes manic-like behaviour with unique pharmacogenetic properties. Nature. 503(7474):72–77.
- Hong N, Park JS, Kim HJ. 2022. Synapto-protective effect of lithium on HIV-1 Tat-induced synapse loss in rat hippocampal cultures. Anim Cells Syst (Seoul). 26(1):1–9.
- Kanellopoulos AK, Mariano V, Spinazzi M, Woo YJ, McLean C, Pech U, Li KW, Armstrong JD, Giangrande A, Callaerts P, et al. 2020. Aralar sequesters GABA into hyperactive mitochondria, causing social behavior deficits. Cell. 180(6):1178–1197.e20.
- Kang M, Zhang Y, Kang HR, Kim S, Ma R, Yi Y, Lee S, Kim Y, Li H, Jin C, et al. 2023. CYFIP2 p.Arg87Cys causes neurological defects and degradation of CYFIP2. Ann Neurol. 93(1):155–163.
- Kim GH, Zhang Y, Kang HR, Lee SH, Shin J, Lee CH, Kang H, Ma R, Jin C, Kim Y, et al. 2020. Altered presynaptic function and number of mitochondria in the medial prefrontal cortex of adult Cyfip2 heterozygous mice. Mol Brain. 13(1):123.
- Kim NS, Ringeling FR, Zhou Y, Nguyen HN, Temme SJ, Lin YT, Eacker S, Dawson VL, Dawson TM, Xiao B, et al. 2022. CYFIP1 dosages exhibit divergent behavioral impact via diametric regulation of NMDA receptor complex translation in mouse models of psychiatric disorders. Biol Psychiatry. 92(10):815–826.
- Lee B, Zhang Y, Kim Y, Kim S, Lee Y, Han K. 2017. Age-dependent decrease of GAD65/67 mRNAs but normal densities of GABAergic interneurons in the brain regions of Shank3-overexpressing manic mouse model. Neurosci Lett. 649:48–54.
- Lee SH, Zhang Y, Park J, Kim B, Kim Y, Lee SH, Kim GH, Huh YH, Lee B, Kim Y, et al. 2020. Haploinsufficiency of Cyfip2 causes lithium-responsive prefrontal dysfunction. Ann Neurol. 88(3):526–543.
- Lee Y, Kim D, Ryu JR, Zhang Y, Kim S, Kim Y, Lee B, Sun W, Han K. 2017. Phosphorylation of CYFIP2, a component of the WAVE-regulatory complex, regulates dendritic spine density and neurite outgrowth in cultured hippocampal neurons potentially by affecting the complex assembly. Neuroreport. 28(12):749–754.
- Lee Y, Zhang Y, Kang H, Bang G, Kim Y, Kang HR, Ma R, Jin C, Kim JY, Han K. 2020. Epilepsy-and intellectual disability-associated CYFIP2 interacts with both actin regulators and RNA-binding proteins in the neonatal mouse forebrain. Biochem Biophys Res Commun. 529(1):1–6.
- Lee Y, Zhang Y, Ryu JR, Kang HR, Kim D, Jin C, Kim Y, Sun W, Han K. 2019. Reduced CYFIP2 stability by Arg87 variants causing human neurological disorders. Ann Neurol. 86(5):803–805.
- Luo L, Ambrozkiewicz MC, Benseler F, Chen C, Dumontier E, Falkner S, Furlanis E, Gomez AM, Hoshina N, Huang WH, et al. 2020. Optimizing nervous system-specific gene targeting with Cre driver lines: prevalence of germline recombination and influencing factors. Neuron. 106(1):37–65.e5.
- Ma R, Pang K, Kang H, Zhang Y, Bang G, Park S, Hwang E, Ryu JR, Kwon Y, Kang HR, et al. 2022. Protein interactome and cell-type expression analyses reveal that cytoplasmic FMR1-interacting protein 1 (CYFIP1), but not CYFIP2, associates with astrocytic focal adhesion. J Neurochem. 162(2):190–206.
- Nakashima M, Kato M, Aoto K, Shiina M, Belal H, Mukaida S, Kumada S, Sato A, Zerem A, Lerman-Sagie T, et al. 2018. De novo hotspot variants in CYFIP2 cause early-onset epileptic encephalopathy. Ann Neurol. 83(4):794–806.
- Napoli I, Mercaldo V, Boyl PP, Eleuteri B, Zalfa F, De Rubeis S, Di Marino D, Mohr E, Massimi M, Falconi M, et al. 2008. The fragile X syndrome protein represses activity-dependent translation through CYFIP1, a new 4E-BP. Cell. 134(6):1042–1054.
- Pathania M, Davenport EC, Muir J, Sheehan DF, Lopez-Domenech G, Kittler JT. 2014. The autism and schizophrenia associated gene CYFIP1 is critical for the maintenance of dendritic complexity and the stabilization of mature spines. Transl Psychiatry. 4:e374.
- Peng J, Wang Y, He F, Chen C, Wu LW, Yang LF, Ma YP, Zhang W, Shi ZQ, Xia K, et al. 2018. Novel West syndrome candidate genes in a Chinese cohort. CNS Neurosci Ther. 24(12):1196–1206.
- Penzes P, Cahill ME, Jones KA, VanLeeuwen JE, Woolfrey KM. 2011. Dendritic spine pathology in neuropsychiatric disorders. Nat Neurosci. 14(3):285–293.
- Rottner K, Stradal TEB, Chen B. 2021. WAVE regulatory complex. Curr Biol. 31(10):R512–R517.
- Schenck A, Bardoni B, Moro A, Bagni C, Mandel JL. 2001. A highly conserved protein family interacting with the fragile X mental retardation protein (FMRP) and displaying selective interactions with FMRP-related proteins FXR1P and FXR2P. Proc Natl Acad Sci USA . 98(15):8844–8849.
- Silva AI, Haddon JE, Ahmed Syed Y, Trent S, Lin TE, Patel Y, Carter J, Haan N, Honey RC, Humby T, et al. 2019. Cyfip1 haploinsufficient rats show white matter changes, myelin thinning, abnormal oligodendrocytes and behavioural inflexibility. Nat Commun. 10(1):3455.
- Spence EF, Soderling SH. 2015. Actin out: regulation of the synaptic cytoskeleton. J Biol Chem. 290(48):28613–28622.
- Tiwari SS, Mizuno K, Ghosh A, Aziz W, Troakes C, Daoud J, Golash V, Noble W, Hortobagyi T, Giese KP. 2016. Alzheimer-related decrease in CYFIP2 links amyloid production to tau hyperphosphorylation and memory loss. Brain. 139(Pt 10):2751–2765.
- Tsien JZ, Chen DF, Gerber D, Tom C, Mercer EH, Anderson DJ, Mayford M, Kandel ER, Tonegawa S. 1996. Subregion- and cell type-restricted gene knockout in mouse brain. Cell. 87(7):1317–1326.
- Tzioras M, McGeachan RI, Durrant CS, Spires-Jones TL. 2023. Synaptic degeneration in Alzheimer disease. Nat Rev Neurol. 19(1):19–38.
- Yu X, Zhang R, Wei C, Gao Y, Yu Y, Wang L, Jiang J, Zhang X, Li J, Chen X. 2021. MCT2 overexpression promotes recovery of cognitive function by increasing mitochondrial biogenesis in a rat model of stroke. Anim Cells Syst (Seoul). 25(2):93–101.
- Zalfa F, Eleuteri B, Dickson KS, Mercaldo V, De Rubeis S, di Penta A, Tabolacci E, Chiurazzi P, Neri G, Grant SG, et al. 2007. A new function for the fragile X mental retardation protein in regulation of PSD-95 mRNA stability. Nat Neurosci. 10(5):578–587.
- Zhang Y, Kang H, Lee Y, Kim Y, Lee B, Kim JY, Jin C, Kim S, Kim H, Han K. 2019a. Smaller body size, early postnatal lethality, and cortical extracellular matrix-related gene expression changes of Cyfip2-null embryonic mice. Front Mol Neurosci. 11:482.
- Zhang Y, Kang HR, Han K. 2019b. Differential cell-type-expression of CYFIP1 and CYFIP2 in the adult mouse hippocampus. Animal Cells Syst (Seoul). 23(6):380–383.
- Zhang Y, Kang HR, Lee SH, Kim Y, Ma R, Jin C, Lim JE, Kim S, Kang Y, Kang H, et al. 2020. Enhanced prefrontal neuronal activity and social dominance behavior in postnatal forebrain excitatory neuron-specific Cyfip2 knock-out mice. Front Mol Neurosci. 13:574947.
- Zhang Y, Lee Y, Han K. 2019c. Neuronal function and dysfunction of CYFIP2: from actin dynamics to early infantile epileptic encephalopathy. BMB Rep. 52(5):304–311.
- Zhong M, Liao S, Li T, Wu P, Wang Y, Wu F, Li X, Hong S, Yan L, Jiang L. 2019. Early diagnosis improving the outcome of an infant with epileptic encephalopathy with cytoplasmic FMRP interacting protein 2 mutation: case report and literature review. Medicine (Baltimore). 98(44):e17749.
- Zweier M, Begemann A, McWalter K, Cho MT, Abela L, Banka S, Behring B, Berger A, Brown CW, Carneiro M, et al. 2019. Spatially clustering de novo variants in CYFIP2, encoding the cytoplasmic FMRP interacting protein 2, cause intellectual disability and seizures. Eur J Hum Genet. 27(5):747–759.