ABSTRACT
Nesfatin-1, a polypeptide hormone derived from the nucleobindin 2 (NUCB2) precursor protein, is known to regulate appetite and energy metabolism. Recent studies have also shown that NUCB2/nesfatin-1 is expressed in the reproductive organs of mice. However, the expression and potential role of NUCB2/nesfatin-1 in the mouse epididymis remain unclear. Therefore, we investigated the expression of NUCB2/nesfatin-1 in the mouse epididymis and its potential function. NUCB2/nesfatin-1 was detected in the epididymis by qRT-PCR and western blotting, and high expression levels were observed in epididymal epithelial cells by immunohistochemical staining. Pregnant mare’s serum gonadotropin (PMSG) and human chorionic gonadotropin (hCG) injections significantly increased NUCB2/nesfatin-1 expression in the epididymis. After castration, NUCB2/nesfatin-1 expression in the epididymis decreased, but was significantly increased by testosterone injection. Nesfatin-1-binding sites were found in the middle piece of testicular sperm, but were scarcely detected in the sperm head. By contrast, nesfatin-1 binding sites were identified on the sperm head within the epididymis. Furthermore, nesfatin-1 treatment inhibited the acrosome reaction in epididymal sperm. These results suggest that the nesfatin-1 protein produced in the epididymis binds to nesfatin-1 binding sites on the sperm head and plays a role in suppressing the acrosome reaction before ejaculation.
1. Introduction
Nesfatin-1 was initially discovered to regulate appetite by suppressing food intake and inducing satiety in the hypothalamus and central nervous system of rodents (Oh-I et al. Citation2006). Recent reports demonstrated that nesfatin-1 is also expressed in peripheral organs of humans and rodents, and functions as a regulatory peptide (Dore et al. Citation2017). In rats, nesfatin-1 immunoreactivity has been identified in the gastric tract, suggesting that it has a role in gastrointestinal function (Li et al. Citation2013; Bertucci et al. Citation2019). Nesfatin-1 is expressed in ghrelin-secreting X/A-like cells in the stomach, which stimulate appetite (Stengel et al. Citation2010). In pancreatic β-cells of mice and rats, nesfatin-1 co-localizes with insulin, suggesting it has a role in glucose homeostasis (Gonzalez et al. Citation2009; Foo et al. Citation2010). Nesfatin-1 has also been detected in human and rodent adipose tissue, with higher expression levels in subcutaneous fat than in visceral fat (Ramanjaneya et al. Citation2010). Additionally, nesfatin-1 is synthesized and secreted by human and rat cardiomyocytes to regulate glucose metabolism in the heart (Feijóo-Bandín et al. Citation2013).
On the other hand, several reports demonstrated the expression of nesfatin-1 protein in the testes and ovaries of humans, mice, and fishes (García-Galiano et al. Citation2010; Gao et al. Citation2016; Sundarrajan et al. Citation2016; Rajeswari et al. Citation2020). Immunohistochemical staining confirmed the localization of nesfatin-1 in interstitial cells, including Leydig cells in the mouse testis (García-Galiano et al. Citation2012). Our previous reports also showed that nucleobindin 2 (NUCB2)/nesfatin-1 is expressed in the testis and is implicated in maintenance of spermiogenesis (Kim et al. Citation2011; Kim et al. Citation2014). Nesfatin-1 in the testes also directly promotes spermatogenesis and steroidogenesis in pre-pubertal male mice (Ranjan et al. Citation2019a). Moreover, nesfatin-1 stimulates testicular function by increasing sperm viability and enhancing the transport of energy substrates through paracrine mechanisms (Ranjan et al. Citation2019b). Additionally, nesfatin-1 preserves spermatogenic cells via the AKT and CREB signaling pathways, as well as by balancing pro- and anti-inflammatory cytokines (Arabaci Tamer et al. Citation2018; Jung et al. Citation2022). Furthermore, nesfatin-1 improves testicular dysfunction and insulin sensitivity in type 2 diabetes mellitus mice by promoting testosterone production and fertility (Ranjan et al. Citation2020). Nesfatin-1 also significantly enhances plasma follicle-stimulating hormone, luteinizing hormone, and testosterone levels without altering the plasma gonadotropin-releasing hormone level in rats (Schalla and Stengel Citation2021).
However, it remains unclear whether NUCB2/nesfatin-1 is expressed in the epididymis and if a nesfatin-1 binding site exists in sperm. In this study, we aimed to investigate the expression of NUCB2 mRNA and nesfatin-1 protein in the epididymis. Furthermore, to investigate the potential function of the nesfatin-1 protein in the epididymis, we also examined the expression of nesfatin-1 binding sites in sperm from the epididymis and its potential role in sperm maturation.
2. Materials and methods
2.1. Animals
Eight-week-old male ICR mice were purchased from Raon Bio (Seoul, Korea) and housed individually in the cage with controlled illumination and temperature. Animal care and experimental procedures were approved by the Institutional Animal Care and Use Committee at the Seoul Women’s University in accordance with guidelines established by the Korea Food and Drug Administration.
2.2. Castration and hormone treatment
Mice were administered intraperitoneal injections of pregnant mare’s serum gonadotropin (PMSG) (10 IU/mouse, Sigma-Aldrich, USA) and human chorionic gonadotropin (hCG) (10 IU/mouse, Sigma-Aldrich, USA). Epididymis tissues were collected three days later. The epididymis tissues were divided into caput, corpus, and cauda parts and then analyzed for mRNA and protein expression levels. For castration, mice were anesthetized by intraperitoneal injection of a combination of Zoletil and Rompun. A dorsal incision was made through the mouse’s flank skin, and the testes were carefully excised, while the epididymis was preserved intact. One day post-castration, one group of mice received an intraperitoneal injection (100 μl/mouse) of vehicle (saline) alone. Another group was administered PMSG and hCG. Additionally, a separate group received a subcutaneous injection of vehicle (sesame oil) alone or testosterone (2 mM/100 μl, Sigma-Aldrich, USA) dissolved in sesame oil (Sigma-Aldrich, USA).
2.3. RNA extraction, conventional PCR, and quantitative real-time PCR (qRT-PCR)
Testis and epididymis were obtained from mice and homogenized, followed by total RNA extraction using chloroform and isopropyl alcohol precipitation. The extracted RNA was used for cDNA synthesis with oligo dT primers, dNTPs (BIO BASIC Inc., Canada), and reverse transcriptase (Invitrogen, USA) in RT buffer (Invitrogen, USA). Conventional PCR was conducted using 2X RbTaqTM PreMIX-Tenuto HOT (Enzynomics, Korea), template cDNA, and specific primers in a Gene Pro thermal cycler (Bioneer, China). Reaction products were separated on a 2% agarose gel and visualized to confirm the size of the amplified cDNA. The relative DNA levels were analyzed using Image J software (National Institutes of Health, USA). qRT-PCR was performed using a buffer solution containing template cDNA, SYBR Green (Dyne Bio, Korea), and specific primers in the Light Cycler 480 Real-time PCR System (Roche, Germany). The primer pairs used are listed in Supplementary Data 1.
2.4. Western blotting
Testis and epididymis were collected from mice, and proteins were extracted using RIPA lysis buffer (Rockland, USA). The samples were then subjected to SDS-PAGE and subsequently transferred to a PVDF membrane. The membrane was blocked with a blocking solution. To assess nesfatin-1 protein expression, the membrane was incubated with a rabbit anti-rat nesfatin-1 polyclonal antibody (H-003-22, Phoenix Pharmaceuticals Inc., USA) and a rabbit anti-β-actin polyclonal antibody (sc-130656, Santa Cruz Biotechnology, USA), followed by incubation with an HRP-conjugated goat anti-rabbit IgG polyclonal antibody (A120-101P, Bethyl Laboratories, USA). The membrane was then visualized with ECL reagents (ThermoFisher, UK), and the relative protein levels were analyzed using Image J software (National Institutes of Health, USA).
2.5. Immunohistochemical staining
Epididymal tissues were fixed in paraformaldehyde buffer saline, rinsed in an ethanol series, and embedded in paraffin blocks. The tissue blocks were sectioned, deparaffinized, and rehydrated through a graded xylene-alcohol series. The sections were incubated with a rabbit anti-rat nesfatin-1 polyclonal antibody (H-003-22, Phoenix Pharmaceuticals Inc., USA). For negative controls, the sections were processed without the primary antibody. Subsequently, the sections were incubated with an Alexa Fluor 488 conjugated goat anti-rabbit IgG (AB_2338052, Jackson ImmunoResearch Laboratories Inc., USA). The sections were then counterstained with DAPI, mounted using a mounting medium (Vector Laboratories, Inc., USA), and observed under a fluorescence microscope (Axioskop2, Carl Zeiss, Germany).
2.6. Localization of nesfatin-1 binding sites in sperm
Testis and epididymal caput, corpus, and cauda were harvested from mice, and sperm were extracted from the tissues. The sperm were incubated with FITC-conjugated rat nesfatin-1 (FG-G-003-22B; Phoenix Pharmaceuticals Inc., USA), counterstained with DAPI, and observed under a fluorescence microscope (Axioskop2, Carl Zeiss, Germany). To assess the percentage of sperm stained with FITC-conjugated nesfatin-1 in the head, midpiece, and tail regions, 200 sperm were counted on each slide in a blind manner.
2.7. Sperm acrosome reaction assay
Sperm were collected from the epididymis and placed in a culture dish containing capacitation media then incubated for 1 h at 37°C. The sperm were treated with 10 μM A23187 (Sigma-Aldrich, USA), 1 μM acetyl tetrapeptide-1 (Cayman Chemical, USA), and 100 nM nesfatin-1 before being incubated overnight at 37°C. To assess the acrosomal status of sperm, they were incubated with acrosome-specific FITC-PNA (Sigma-Aldrich, USA) and Hoechst 33342 (Sigma-Aldrich, USA) and examined under a fluorescence microscope (Axioskop2, Carl Zeiss, Germany). To evaluate the percentage of acrosome-reacted sperm, 200 sperm were counted on each slide in a blind manner.
2.8. Statistical analysis
The results were presented as the mean and the standard error of the mean (SEM). Data were analyzed by the Student t-test. A p-values of less than 0.05 were considered statistically significant.
3. Results
3.1. Expression of nesfatin-1 protein and NUCB2 mRNA in the testis and epididymis
Nesfatin-1 protein was identified in the testis and the epididymal caput, corpus, and cauda regions using western blotting analysis. Levels of nesfatin-1 protein were higher in the epididymal caput, corpus, and cauda compared to the testis (A). In addition, NUCB2 mRNA was detected in the caput, and corpus using conventional PCR and qRT-PCR. Consistent with the western blotting results, NUCB2 mRNA expression was higher in the epididymal caput, corpus, and cauda than the testis ((B, C)). Immunohistochemical staining revealed that nesfatin-1 was expressed in epithelial cells of the epididymal caput, corpus, and cauda (D).
Figure 1. NUCB2 mRNA and nesfatin-1 protein expression in the mouse epididymis. (A) Conventional RT-PCR analysis revealed the presence of NUCB2 mRNA in the testis and the caput, corpus, and cauda of epididymis. (B) Western blotting showed the existence of nesfatin-1 protein in the same samples. (C) NUCB2 mRNA expression levels were analyzed in the same samples by qRT-PCR. The expression levels were higher in all regions of the epididymis than in the testis. (D) Localization of nesfatin-1 protein in the epididymis. Nesfatin-1 protein was expressed in many epithelial cells in the caput, corpus, and cauda of the epididymis. Original magnification, 100× and 400×. A statistically significant difference was considered when p < 0.05 compared to the testes (* p < 0.05).
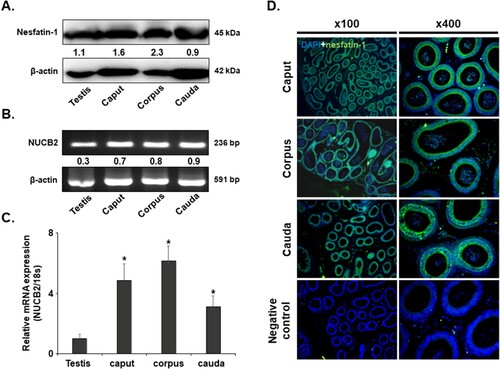
3.2. NUCB2 mRNA expression in the epididymis after PMSG and hCG injections
To investigate whether gonadotropin regulates NUCB2 mRNA expression in the epididymis, we injected PMSG and hCG into mice and measured NUCB2 mRNA levels in the epididymis by qRT-PCR. NUCB2 mRNA expression was significantly higher in mice treated with both PMSG and hCG than in the control group. Additionally, we examined nesfatin-1 protein expression in the epididymis after PMSG and hCG injections by western blotting. Consistent with the qRT-PCR results, nesfatin-1 protein expression in the epididymis was higher in mice treated with PMSG and hCG than in the control group ((A, B)). To assess whether NUCB2 mRNA expression is regulated by testosterone, mice were castrated and injected with testosterone. NUCB2 mRNA expression was significantly decreased in the caput, corpus, and cauda after castration. However, when castrated mice were injected with testosterone, NUCB2 mRNA expression significantly increased in the caput and cauda, but not in the corpus. Additionally, NUCB2 mRNA expression in the epididymis did not increase after the injection of castrated mice with PMSG and hCG (C).
Figure 2. NUCB2 mRNA and nesfatin-1 protein expression in the epididymis after PMSG and hCG treatment. (A, B) NUCB2 mRNA and nesfatin-1 protein expression in the epididymis was assessed by qRT-PCR and western blotting after mice were injected with PMSG and hCG. NUCB2 mRNA and nesfatin-1 protein expression in the epididymis increased following co-injection with PMSG and hCG. (C) NUCB2 mRNA expression in the epididymis was assessed by qRT-PCR after injection of castrated mice with PMSG + hCG and testosterone. NUCB2 mRNA expression significantly decreased in the caput, corpus, and cauda after castration. However, when castrated mice were injected with testosterone, NUCB2 mRNA expression significantly increased in the caput and cauda, but not in the corpus. Additionally, NUCB2 mRNA expression in the epididymis did not increase after co-injection of castrated mice with PMSG and hCG. All data are presented as mean ± SEM (n = 5). Differences between values were considered statistically significant when p < 0.05.
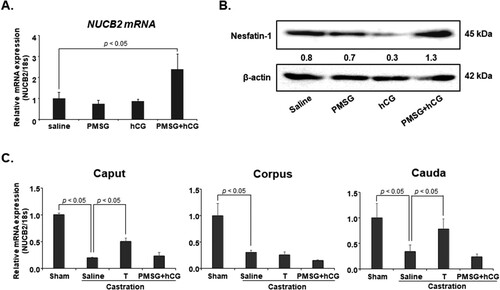
3.3. Localization of nesfatin-1 binding sites in sperm
Before investigating the effects of nesfatin-1 on the acrosome reaction of sperm in the epididymis, we first assessed the presence of nesfatin-1 binding sites in sperm. We found that nesfatin-1 binding sites were present in sperm from both the epididymis and testis. Interestingly, in the epididymis, nesfatin-1 binding sites were observed in the middle part and head of sperm. In contrast, in the testis, these sites were only present in the middle part of sperm, not in the head (A). Currently, the receptor structure for nesfatin-1 has not been identified, making antibodies against the nesfatin-1 receptor unavailable. Instead, many studies have used fluorescent conjugated nesfain-1 to determine the binding site of this peptide. Sperm staining with FITC-conjugated nesfatin-1 in the head, midpiece, and tail regions exhibited distinctive patterns. In testicular sperm, the staining in the head region was extremely low, almost negligible. In contrast, nearly 100% of epididymal sperm displayed staining in the head. Staining in the midpiece region was consistently high for both testicular and epididymal sperm, with over 80% stained. However, staining in the tail region was notably low for both types of sperm, with less than 15% stained (B).
Figure 3. Localization of nesfatin-1 binding sites in sperm. (A) Representative images of testicular and epididymal sperm stained with FITC-conjugated nesfatin-1 to identify nesfatin-1 binding sites. Nesfatin-1 binding sites were detected in both the midpiece and head regions of sperm from the epididymis. In contrast, for sperm from the testis, nesfatin-1-binding sites were observed only in the midpiece, with no binding in the head region. (B) Quantification of sperm stained with FITC-conjugated nesfatin-1 in the head, midpiece, and tail regions. The percentage of testicular sperm stained with FITC-conjugated nesfatin-1 in the head is extremely low. However, for epididymal sperm, the percentage of sperm stained in the head region is nearly 100%. A statistically significant difference was considered when p < 0.05 compared to the testes (* p < 0.05).
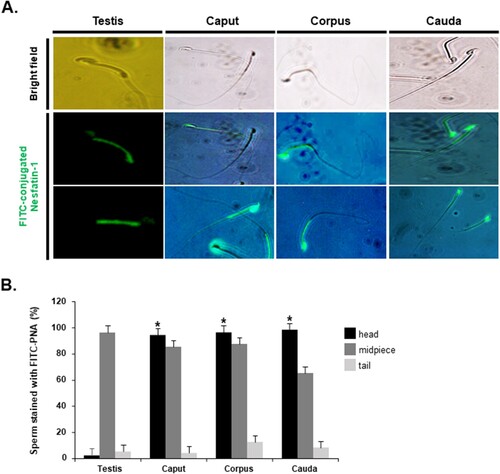
3.4. Effect of nesfatin-1 on the acrosome reaction in epididymal sperm
The effects of nesfatin-1 on the acrosome reaction were evaluated using the FITC-PNA assay. Acrosome-reacted sperm display a lack of fluorescence or a disrupted fluorescence pattern in the acrosomal region, while non-reacted sperm exhibit a bright, uniform fluorescence pattern. When sperm were exposed to A23187, a known inducer of the acrosome reaction, there was a significant increase in acrosome reactions compared with the control group. Notably, nesfatin-1 treatment of sperm with A23187-induced acrosome reactions resulted in the inhibition of the acrosome reaction. In contrast, when sperm with A23187-induced acrosome reactions were treated with acetyl tetrapeptide-1, which does not protect against the acrosome reaction, there was an increase in acrosome reactions compared with the control group (A). 58.5 ± 4.5% of sperm exhibited reacted acrosomes in the control group, but 83.5 ± 8.3% in A23187-treated group. Furthermore, when co-treated with A23187 and nesfatin-1, only 48.3 5 ± 3.9% of sperm reacted. In contrast, 81.3 ± 4.7% of sperm co-treated with A23187 and acetyl tetrapeptide-1 reacted. These results showed that nesfatin-1 treatment significantly suppressed acrosome reaction in mice sperm (B).
Figure 4. Effect of nesfatin-1 on the acrosome reaction in epididymal sperm. (A) Representative images of epididymal sperm stained with FITC-PNA to detect the acrosome after treatment with 10 μM A23187 and 100 nM nesfatin-1 for 24 h. Acrosome-intact sperm display bright green fluorescence over the acrosomal cap, while acrosome-reacted sperm exhibit no FITC-PNA staining. Sperm treated with A23187 showed weak labeling of the head, while those co-treated with A23187 and nesfatin-1 displayed strong labeling of the head, indicating that the acrosome reaction was inhibited. In contrast, sperm co-treated with acetyl tetrapeptide-1, which does not protect against the acrosome reaction, and A23187 showed weak labeling of the head. (B) Quantification of acrosome-reacted sperm after nesfatin-1 treatment. The percentage of sperm displaying the acrosome reaction increased after A23187, an inducer of the acrosome reaction. However, when sperm were co-treated with A23187 and nesfatin-1, the percentage of sperm displaying the acrosome reaction was lower than treatment with A23187 alone. In contrast, co-treatment with acetyl tetrapeptide-1, which does not protect against the acrosome reaction, increased in acrosome reactions, similar to the control group. All data are represented as mean ± SEM (n = 5). Differences between values were considered statistically significant when p < 0.05.
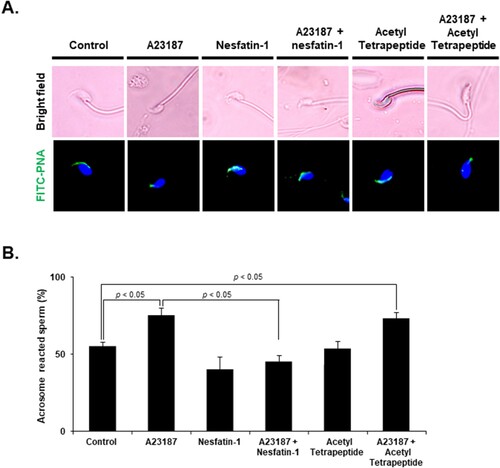
4. Discussion
Nesfatin-1, a neuronal peptide responsible for regulating food intake and inducing satiety, is expressed in the hypothalamus and central nervous system (Goebel et al. Citation2009; Goebel-Stengel et al. Citation2011). However, recent studies revealed that NUCB2/nesfatin-1 is not only expressed in the hypothalamus, but also peripheral organs such as the pancreas, stomach, intestines, liver, kidneys, and adipose and reproductive tissues (Schalla and Stengel Citation2018; Bertucci et al. Citation2019; Puzio et al. Citation2021; Goyal and Dhar Citation2022). We have also demonstrated the expression of NUCB2/nesfatin-1 in the reproductive organs of mice, including ovaries, the uterus, and testes (Kim et al. Citation2011; Kim et al. Citation2014). However, the expression and potential role of NUCB2/nesfatin-1 in the epididymis of mice remained unknown.
In this study, we demonstrated that NUCB2 mRNA and nesfatin-1 protein are expressed in the mouse epididymis for the first time. NUCB2 mRNA and nesfatin-1 protein were detected in epithelial cells of the caput, corpus, and cauda of the epididymis. The epididymis is a highly convoluted tubular structure adjacent to the testis and is divided into three main regions: the caput, corpus, and cauda (Sullivan et al. Citation2019). Sperm produced in the testes travel through the epididymis, where they acquire motility and the ability to fertilize eggs through sperm maturation (Ahn et al. Citation2022; Miyaso et al. Citation2022). The epididymis plays a vital role in this process by providing an optimal environment, including essential nutrients and various molecules secreted by epithelial cells in the epididymis (Sullivan and Mieusset Citation2016). Hundreds of epididymal proteins have been identified in the epididymal fluid of different species, many of which are common to various species (Dacheux and Dacheux Citation2013). Given this information, our findings suggest that nesfatin-1 is a novel protein produced in the epididymis that may play a role in sperm maturation.
Based on the understanding that testosterone stimulates the production of proteins related to sperm maturation in epididymal epithelial cells (Robaire et al. Citation2007), we hypothesized that testosterone may also plays a role in regulating the production of NUCB2/nesfatin-1 in the epididymis. To investigate this hypothesis, we injected mice with pregnant mare’s serum gonadotropin (PMSG) and human chorionic gonadotropin (hCG). PMSG is a complex glycoprotein obtained from the serum of pregnant mares that can supplement or substitute for follicle stimulating hormone (FSH) in both males and females (Lunenfeld Citation2011) Human chorionic gonadotropin (hCG), a hormone produced by the placenta during pregnancy, is analogous to luteinizing hormone (LH) (Cole Citation2009). Therefore, PMSG and hCG are commonly used to stimulate ovarian follicle development and E2 secretion from the ovaries, as well as sperm production and testosterone secretion from the testes. For this reason, we injected castrated mice with PMSG and hCG to eliminate the impact of testosterone on the epididymis and subsequently measured NUCB2 mRNA levels. NUCB2 mRNA expression in the epididymis was significantly decreased following castration, but its expression significantly increased after testosterone injection. Interestingly, NUCB2 mRNA expression increased in the caput and cauda, but did not increase in the corpus after testosterone injection. The corpus region of the epididymis is smaller than the caput and cauda, and it is less influenced by testosterone to express more proteins and genes involved in sperm maturation and motility (Browne et al. Citation2016; Légaré and Sullivan Citation2020). This might explain why NUCB2 mRNA expression in the corpus after testosterone administration was lower than in the other two regions. In addition, NUCB2 mRNA expression did not significantly increase when PMSG and hCG were administered to castrated mice. This finding indicates that PMSG and hCG do not directly regulate NUCB2 mRNA expression in the epididymis. Instead, they exert an indirect effect through testosterone secreted from the testes, which is controlled by PMSG and hCG.
Next, we investigated whether nesfatin-1 affects the acrosome reaction of sperm. If nesfatin-1 affects sperm, nesfatin-1 binding sites like receptors should be present on sperm. To investigate this, we conducted staining with FITC-conjugated nesfatin-1 to identify nesfatin-1 binding sites on sperm. Our findings showed that nesfatin-1 binding sites were present on epididymal and testicular sperm. Remarkably, in the epididymis, nesfatin-1 binding sites were found in the middle part and head of sperm, whereas in the testis, they were only found in the middle part of sperm, not in the head. During sperm maturation in the epididymis, significant changes occur in the sperm head, which contains the acrosome (Yoshinaga and Toshimori Citation2003). These changes involve modifications to the sperm head membrane, including alterations in the protein and lipid distribution, which affect the acrosome’s functionality. Our study demonstrated that nesfatin-1 binding sites are found in both the middle part and head of sperm in the epididymis, but only in the middle part of sperm, not in the head, in the testis. These findings suggest that nesfatin-1 plays a crucial role in facilitating sperm maturation in the epididymis, potentially by binding to its receptors on the sperm head in the epididymis.
Because we found that nesfatin-1 binds to sperm, we investigated the effects of nesfatin-1 on the acrosome reaction. Our results demonstrated that co-treatment with nesfatin-1 decreased the acrosome reaction induced by A23187, a well-known trigger of the acrosome reaction. To clarify the impact of nesfatin-1 protein on the acrosome reaction, sperm were co-treated with A23187 and acetyl tetrapeptide-1 that does not protect sperm from the acrosome reaction. This co-treatment increased in the acrosome reaction. These findings suggest that nesfatin-1, secreted from the epididymis, inhibits the acrosome reaction. Maintaining the intact acrosome until fertilization of the egg is critical for successful fertilization (Siu et al. Citation2021). Therefore, inhibiting the acrosome reaction of sperm in the epididymis until ejaculation is crucial to maintain fertility. Taken together, our findings suggest that nesfatin-1 is one of the substances that inhibit the acrosome reaction, contributing to the preservation of sperm quality and fertility.
In summary, the present study demonstrated that NUCB2/nesfatin-1 is expressed in the caput, corpus, and cauda of the epididymis, and testosterone produced by the testis regulates the expression of NUCB2/nesfatin-1. We also found that nesfatin-1 binds to sperm, suggesting that nesfatin-1 influences the function of epididymal sperm under the control of the testis. However, further research is required to elucidate the precise function of nesfatin-1 in the epididymis and its impact on sperm maturation and fertility.
Disclosure statement
No potential conflict of interest was reported by the author(s).
References
- Ahn JS, Won JH, Kim DY, Jung SE, Kim BJ, Kim JM, Ryu BY. 2022. Transcriptome alterations in spermatogonial stem cells exposed to bisphenol A. Anim Cells Syst. 26(2):70–83.
- Arabaci Tamer S, Yildirim A, Köroğlu MK, Çevik Ö, Ercan F, Yeğen BÇ. 2018. Nesfatin-1 ameliorates testicular injury and supports gonadal function in rats induced with testis torsion. Peptides. 107:1–9.
- Bertucci JI, Blanco AM, Sánchez-Bretaño A, Unniappan S, Canosa LF. 2019. Ghrelin and NUCB2/nesfatin-1 co-localization with digestive enzymes in the intestine of pejerrey (Odontesthes bonariensis). Anat Rec (Hoboken). 302(6):973–982.
- Browne JA, Yang R, Leir SH, Eggener SE, Harris A. 2016. Expression profiles of human epididymis epithelial cells reveal the functional diversity of caput, corpus and cauda regions. Mol Hum Reprod. 22(2):69–82.
- Cole LA. 2009. New discoveries on the biology and detection of human chorionic gonadotropin. Reprod Biol Endocrinol. 7:8.
- Dacheux JL, Dacheux F. 2013. New insights into epididymal function in relation to sperm maturation. Reproduction. 147(2):R27–R42.
- Dore R, Levata L, Lehnert H, Schulz C. 2017. Nesfatin-1: functions and physiology of a novel regulatory peptide. J Endocrinol. 232(1):R45–R65.
- Feijóo-Bandín S, Rodríguez-Penas D, García-Rúa V, Mosquera-Leal A, Otero MF, Pereira E, Rubio J, Martínez I, Seoane LM, Gualillo O, et al. 2013. Nesfatin-1 in human and murine cardiomyocytes: synthesis, secretion, and mobilization of GLUT-4. Endocrinology. 154(12):4757–4767.
- Foo KS, Brauner H, Ostenson CG, Broberger C. 2010. Nucleobindin-2/nesfatin in the endocrine pancreas: distribution and relationship to glycaemic state. J Endocrinol. 204(3):255–263.
- Gao X, Zhang K, Song M, Li X, Luo L, Tian Y, Zhang Y, Li Y, Zhang X, Ling Y, et al. 2016. Role of nesfatin-1 in the reproductive axis of male rat. Sci Rep. 6:32877.
- García-Galiano D, Navarro VM, Gaytan F, Tena-Sempere M. 2010. Expanding roles of NUCB2/nesfatin-1 in neuroendocrine regulation. J Mol Endocrinol. 45(5):281–290.
- García-Galiano D, Pineda R, Ilhan T, Castellano JM, Ruiz-Pino F, Sánchez-Garrido MA, Vazquez MJ, Sangiao-Alvarellos S, Romero-Ruiz A, Pinilla L, et al. 2012. Cellular distribution, regulated expression, and functional role of the anorexigenic peptide, NUCB2/nesfatin-1, in the testis. Endocrinology. 153(4):1959–1971.
- Goebel M, Stengel A, Wang L, Lambrecht NW, Taché Y. 2009. Nesfatin-1 immunoreactivity in rat brain and spinal cord autonomic nuclei. Neurosci Lett. 452(3):241–246.
- Goebel-Stengel M, Wang L, Stengel A, Taché Y. 2011. Localization of nesfatin-1 neurons in the mouse brain and functional implication. Brain Res. 1396:20–34.
- Gonzalez R, Tiwari A, Unniappan S. 2009. Pancreatic beta cells colocalize insulin and pronesfatin immunoreactivity in rodents. Biochem Biophys Res Commun. 381(4):643–648.
- Goyal SG, Dhar A. 2022. Downregulation of nesfatin-1 expression in acute kidney injury in vivo in wistar rats and in vitro in cultured cells. Life Sci. 305:e120762.
- Jung H, Kim YS, Jung DM, Lee KS, Lee JM, Kim KK. 2022. Melittin-derived peptides exhibit variations in cytotoxicity and antioxidant, anti-inflammatory and allergenic activities. Anim Cells Syst. 26(4):158–165.
- Kim J, Chung Y, Kim H, Im E, Lee H, Yang H. 2014. The tissue distribution of nesfatin-1/NUCB2 in mouse. Dev Reprod. 18(4):301–309.
- Kim J, Kim H, Kim S, Yang H, Cho H, Hwang S, Moon C, Yang H. 2011. Expression of nesfatin-1/NUCB2 and its binding site in mouse testis and epididymis. Dev Reprod. 3:249–256.
- Légaré C, Sullivan R. 2020. Differential gene expression profiles of human efferent ducts and proximal epididymis. Andrology. 8(3):625–636.
- Li Z, Mulholland M, Zhang W. 2013. Regulation of gastric nesfatin-1/NUCB2. Curr Pharm Des. 19(39):6981–6985.
- Lunenfeld B. 2011. Gonadotropin stimulation: past, present and future. Reprod Med Biol. 11(1):11–25.
- Miyaso H, Ogawa Y, Itoh M. 2022. Microenvironment for spermatogenesis and sperm maturation. Histochem Cell Biol. 157(3):273–285.
- Oh-I S, Shimizu H, Satoh T, Okada S, Adachi S, Inoue K, Eguchi H, Yamamoto M, Imaki T, Hashimoto K, et al. 2006. Identification of nesfatin-1 as a satiety molecule in the hypothalamus. Nature. 443(7112):709–712.
- Puzio I, Muszyński S, Dobrowolski P, Kapica M, Pawłowska-Olszewska M, Donaldson J, Tomaszewska E. 2021. Alterations in small intestine and liver morphology, immunolocalization of leptin, ghrelin and nesfatin-1 as well as immunoexpression of tight junction proteins in intestinal mucosa after gastrectomy in rat model. J Clin Med. 10(2):272.
- Rajeswari JJ, Hatef A, Unniappan S. 2020. Nesfatin-1-like peptide suppresses hypothalamo-pituitary-gonadal mRNAs, gonadal steroidogenesis, and oocyte maturation in fish. Biol Reprod. 103(4):802–816.
- Ramanjaneya M, Chen J, Brown JE, Tripathi G, Hallschmid M, Patel S, Kern W, Hillhouse EW, Lehnert H, Tan BK, et al. 2010. Identification of nesfatin-1 in human and murine adipose tissue: a novel depot-specific adipokine with increased levels in obesity. Endocrinology. 151(7):3169–3180.
- Ranjan A, Choubey M, Yada T, Krishna A. 2019b. Direct effects of neuropeptide nesfatin-1 on testicular spermatogenesis and steroidogenesis of the adult mice. Gen Comp Endocrinol. 271:49–60.
- Ranjan A, Choubey M, Yada T, Krishna A. 2020. Nesfatin-1 ameliorates type-2 diabetes-associated reproductive dysfunction in male mice. J Endocrinol Invest. 43(4):515–528.
- Ranjan S, Choubey M, Yada T, Krishna A. 2019a. Immunohistochemical localization and possible functions of nesfatin-1 in the testis of mice during pubertal development and sexual maturation. J Mol Histol. 50(6):533–549.
- Robaire B, Seenundun S, Hamzeh M, Lamour SA. 2007. Androgenic regulation of novel genes in the epididymis. Asian J Androl. 9(4):545–553.
- Schalla MA, Stengel A. 2018. Current understanding of the role of nesfatin-1. J Endocr Soc. 2(10):1188–1206.
- Schalla MA, Stengel A. 2021. The role of the gastric hormones ghrelin and nesfatin-1 in reproduction. Int J Mol Sci. 22(20):e11059.
- Siu KK, Serrão VHB, Ziyyat A, Lee JE. 2021. The cell biology of fertilization: Gamete attachment and fusion. J Cell Biol. 220(10):e202102146.
- Stengel A, Goebel M, Wang L, Taché Y. 2010. Ghrelin, des-acyl ghrelin and nesfatin-1 in gastric X/A-like cells: role as regulators of food intake and body weight. Peptides. 31(2):357–369.
- Sullivan R, Légaré C, Lamontagne-Proulx J, Breton S, Soulet D. 2019. Revisiting structure/functions of the human epididymis. Andrology. 7(5):748–757.
- Sullivan R, Mieusset R. 2016. The human epididymis: its function in sperm maturation. Hum Reprod Update. 22(5):574–587.
- Sundarrajan L, Blanco AM, Bertucci JI, Ramesh N, Canosa LF, Unniappan S. 2016. Nesfatin-1-like peptide encoded in nucleobindin-1 in goldfish is a novel anorexigen modulated by sex steroids, macronutrients and daily rhythm. Sci Rep. 6:e28377.
- Yoshinaga K, Toshimori K. 2003. Organization and modifications of sperm acrosomal molecules during spermatogenesis and epididymal maturation. Microsc Res Tech. 61(1):39–45.