ABSTRACT
The Hippo signaling pathway is a conserved signaling pathway that regulates cell proliferation, apoptosis, and differentiation and plays essential roles in the maintenance of organ size and tissue homeostasis. Also, this signaling pathway is a critical regulator of malignant progression in a wide range of cancers. Furthermore, it has been established that YAP and TAZ are major effectors of the Hippo signaling pathway and transcription co-activators that regulate the expressions of genes involved in cell growth and differentiation. Long non-coding RNAs (lncRNAs) are transcripts longer than 200 nucleotides involved in several biological processes, including chromatin remodeling, transcriptional regulation, and post-transcriptional processing. Evidence suggests that the aberrant expression and dysregulation of lncRNAs contribute to the development and progression of various malignancies by acting as oncogenes or tumor suppressors. In addition, many studies have shown that lncRNAs can directly or indirectly regulate the Hippo-YAP/TAZ signaling pathway in cancer. Here, we overview the roles played by lncRNAs in tumor progression with focus on their regulation of the Hippo-YAP/TAZ signaling pathway and discuss their potential usages as clinical biomarkers and therapeutic targets.
1. Introduction
Cancer is a leading cause of global mortality, which is largely attributed to challenges in early diagnosis, difficulties in radical medical procedure of treatment, and high recurrence rates (Sun et al. Citation2019; Siegel et al. Citation2022; Roh, Im, et al. Citation2023). Despite advances in screening, prevention, and treatment, the incidence of cancer is predicted to continue to rise due to societal aging and lifestyle risk factors (Torre et al. Citation2016; Son et al. Citation2017; Hulvat Citation2020). Cancer cells exhibit a variety of functional changes in growth, proliferation, apoptosis, invasiveness, and metastasis, and these changes are mediated mainly by altered expression of genes involved in signaling pathways (Hanahan and Weinberg Citation2011; Kontomanolis et al. Citation2021; Vaghari-Tabari et al. Citation2021). Therefore, it is critical to understand the detailed mechanisms responsible for oncogenic dysregulations in signaling pathways for improving the diagnosis, prognosis, and treatment of cancer (Do et al. Citation2019; Kontomanolis et al. Citation2021).
The Hippo signaling pathway is a well-known canonical signaling pathway and is frequently altered in cancer (Sanchez-Vega et al. Citation2018; Park et al. Citation2020). This pathway is an evolutionarily conserved signaling cascade that was first identified as a key regulator of Drosophila melanogaster development (Ma et al. Citation2019; Dey et al. Citation2020). In mammals, the Hippo pathway controls a variety of biological functions, including cell growth and differentiation, organ development, and tissue regeneration (Ma et al. Citation2019). On the other hand, dysregulation of this pathway has been linked to numerous human cancers, and efforts are being made to target key components for therapeutic intervention (Calses et al. Citation2019; Fu et al. Citation2022). The principal elements of the Hippo pathway in mammals are an upstream kinase cascade and downstream effectors (Ehmer and Sage Citation2016; Cheng et al. Citation2020; Mohajan et al. Citation2021). The core of the kinase cascade includes mammalian STE20-like protein kinase 1/2 (MST1/2; the ortholog of Hippo in Drosophila melanogaster), large tumor suppressor kinase 1/2 (LATS1/2), and their adaptor proteins, Salvardor family WW domain-containing protein 1 (SAV1) and MOB kinase activator 1A/B (MOB1A/B) (Halder and Johnson Citation2011; Mo et al. Citation2014). The downstream effectors, Yes-associated protein 1 (YAP) and transcriptional coactivator with PDZ-binding motif (TAZ), interact with the TEA domain transcription factor (TEAD) family as co-activators (Pocaterra et al. Citation2020; Currey et al. Citation2021). Hippo and YAP/TAZ signaling can be disrupted in various ways, which include epigenetic modification and deletion of upstream cascade component genes, amplification of downstream transcriptional activators, mutations in G protein coupled receptors, or interference with other signaling pathways, such as the Wnt signaling pathway (Plouffe et al. Citation2015). Notably, mutations in components of the Hippo-YAP/TAZ signaling pathway are relatively uncommon. For example, inactivating mutations in upstream kinases, MST1/2 and LATS1/2, which are broadly detected in many types of cancer are present in less than 10% of cancers (Cunningham and Hansen Citation2022). For this reason, it is important to focus on mutations in other regulatory factors that lead to dysregulation of major components in the Hippo-YAP/TAZ signaling pathway, such as MST1/2, LATS1/2, YAP/TAZ, and TEAD.
Long non-coding RNAs (lncRNAs) are transcripts longer than 200 nucleotides (Kapranov et al. Citation2007). lncRNAs are tissue-specifically expressed, usually at lower levels than protein-coding genes (Derrien et al. Citation2012). lncRNAs have attracted research attention because of their involvements in various biological processes, such as chromatin modification, transcriptional regulation, and post-transcriptional regulation (Mercer et al. Citation2009; Do and Kim Citation2018; Yao et al. Citation2019) and are being increasingly recognized as key players in the development and progression of cancer and brain and heart diseases (Papait et al. Citation2013; Chae et al. Citation2021; Seo et al. Citation2021). In particular, lncRNAs participate in a variety of signaling pathways, either directly or indirectly, and act as oncogenes or tumor suppressors to regulate a range of cancer cell behaviors, including cell growth, invasion, metastasis, and apoptosis (Seo et al. Citation2019; Slack and Chinnaiyan Citation2019; Chen K et al. Citation2022). Furthermore, accumulating evidence suggests that the competing endogenous RNA (ceRNA) interplay between lncRNA and microRNA (miRNA) impacts cancer development and progression (Seo et al. Citation2020; Roh et al. Citation2022). Here, we categorize and summarize the latest research findings on oncogenic and tumor-suppressive lncRNAs that regulate the Hippo-YAP/TAZ signaling pathway. In addition, we highlight several studies that demonstrate lncRNAs contribute to cancer therapy-resistance through the Hippo-YAP/TAZ pathway.
2. The association between the Hippo-YAP/TAZ signaling pathway and lncRNAs in cancer
Recently, several significant interactions between lncRNAs and the Hippo-YAP/TAZ signaling pathway have been reported in tumors. For instance, ZMIZ1 antisense RNA 1 (ZMIZ1-AS1) was reported to be an oncogenic lncRNA upregulated by downstream effector proteins of the Hippo pathway that promoted the progression of osteosarcoma (Shen et al. Citation2021; Zhou et al. Citation2022). The well-known lncRNA H19 is also found to be an oncogene elevated by YAP overexpression that contributes to the development of osteosarcoma and bladder cancer (Chan et al. Citation2014; Li et al. Citation2015). On the other hand, long intergenic non-protein coding RNA 1508 (LINC01508) is identified as a tumor-suppressive lncRNA that inhibits the progression of ovarian cancer by downregulating the expression of YAP (Xiao et al. Citation2021). lncRNA LOC554202 (also known as MIR31HG) enhances thyroid cancer cell proliferation by suppressing upstream factors of Hippo signaling (Chen C et al. Citation2022), and Gene Ontology analysis suggests that the Hippo-YAP/TAZ pathway is a downstream target of lncRNA DLGAP1 antisense RNA 2 (DLGAP1-AS2), which is reported to be correlated with poor survival in gastric cancer patients (Soltani et al. Citation2022). Small nucleolar RNA host gene 14 (SNHG14), a lncRNA overexpressed in colorectal cancer, is shown to enhance YAP-targeted KRAS expression by regulating the YAP activity (Shen et al. Citation2023) and notably, to establish a feedback loop transcriptionally activated by a common effector molecule of YAP. In a similar way, an oncogenic lncRNA, RP11-40C6.2, forms a feedback loop that promotes YAP stabilization after being transcriptionally activated by YAP/TAZ and TEAD complex in hepatitis B virus-associated hepatocellular carcinoma (Zhuo et al. Citation2023).
Although the Hippo-YAP/TAZ signaling pathway can influence cancer by modulating the expressions of lncRNAs, numerous studies have demonstrated that lncRNAs often serve as regulators of the Hippo-YAP/TAZ pathway during oncogenesis. Specifically, the roles of lncRNAs in cancer hinge on their targeting components of the Hippo-YAP/TAZ pathway and their regulatory functions (). lncRNAs that up- or downregulate oncogenic or tumor-suppressive components of the Hippo-YAP/TAZ pathway exhibit pro-tumorigenic effects, whereas lncRNAs that exert opposite regulatory effects possess anti-tumorigenic properties. Given these connections, it is crucial to systematically identify and classify oncogenic or tumor-suppressive lncRNAs that modulate the Hippo-YAP/TAZ pathway to provide insight into potential targets for cancer diagnosis and therapeutic intervention.
Figure 1. Hippo-YAP/TAZ signaling pathway regulated by lncRNAs. In the classical Hippo-YAP/TAZ signaling pathway, YAP/TAZ/TEAD are pro-tumorigenic components promoting cell proliferation, invasion, migration, stemness, and anti-apoptosis in cancer cells. MST1/2, LATS1/2, and their adaptor proteins, such as SAV1 and MOB1A/B, which can repress the transcriptional activity of YAP and TAZ, usually suppress tumors. Proto-oncogenic lncRNAs decrease the activity of MST1/2, LATS1/2, and their adaptor proteins, thereby stimulating YAP/TAZ/TEAD signaling, whereas tumor-suppressive lncRNAs increase. Some lncRNAs directly affect the activity of YAP/TAZ/TEAD, exerting their proto-oncogenic or tumor-suppressive activities.
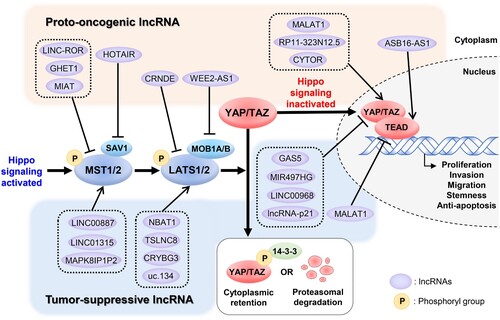
3. Regulations of MST1/2 or LATS1/2 by lncRNAs in cancer
The classical Hippo signaling pathway functions as a kinase cascade that includes MST1/2, LATS1/2, and their adaptor proteins, which all are activated by various factors, such as cell density, polarity, and mechanical signals that ultimately inhibit the transcriptional activity of YAP/TAZ complex (Meng et al. Citation2016; Kim and Jho Citation2018). In this process, phosphorylated MST1/2 kinases interact with the scaffold protein SAV1, and then phosphorylate and activate LATS1/2 kinases (Galan and Avruch Citation2016; Wang Y. et al. Citation2020; de Amorim et al. Citation2021). Bound to the scaffold protein MOB1A/B, activated LATS1/2 kinases phosphorylate YAP and TAZ, both of which interact with the protein 14-3-3 to remain in the cytoplasm (Hergovich and Hemmings Citation2009; Cornils et al. Citation2011; Gundogdu and Hergovich Citation2019). Alternatively, YAP and TAZ are marked for proteasomal degradation (Heng et al. Citation2021). Conversely, when the upstream kinase cascade is inactivated, dephosphorylated YAP and TAZ are translocated to the nucleus, where they bind to TEAD family members or other factors to turn on various genes to promote cell proliferation, differentiation, and development (Piccolo et al. Citation2014; Varelas Citation2014). Accumulating evidence indicates that malfunctioning of the components in the cascade of lncRNA-regulating kinases leads to malignancy (). Regulation of the upstream kinase cascade by lncRNAs can result in the over- or under-expression of downstream effectors. In this section, we focus on lncRNAs that specifically regulate upstream kinases and adaptor proteins in the Hippo-YAP/TAZ pathway.
Table 1. lncRNAs that regulate MST1/2 or LATS1/2 in cancer.
3.1. Positive regulations of MST1/2 or LATS1/2 by lncRNAs
In the Hippo pathway, the kinase cascade serves a tumor-suppressive function by inhibiting the activity of proto-oncogenic component, that is, YAP/TAZ complex (Avruch et al. Citation2012). Several lncRNAs have been reported to exert tumor-suppressive functions by upregulating MST1/2 or LATS1/2. In vitro analysis demonstrated that LINC00887 is downregulated in human cervical cancer cells and this lncRNA sponges miR-454-3p to increase the expression of FERM domain-containing 6 (FRMD6) gene (Li et al. Citation2021). Notably, FRMD6 is found to phosphorylate MST1/2 and the phosphorylation results in the phosphorylation of LATS1/2 and TAZ and suppresses cell proliferation and invasion (Angus et al. Citation2012; Li et al. Citation2021). Furthermore, LINC01315, poorly expressed in oral squamous cell carcinoma, is observed to competitively bind miR-211 and interfere with the interaction between miR-211 and DLG3 mRNA (Chan and Tay Citation2018). As a result, the inhibitory effect of miR-211 on the expression of DLG3 is attenuated, upregulating the level of DLG3 (Chen FB et al. Citation2020). This process facilitates the interaction between DLG3 and MST1/2 binding, which in turn activates the kinase cascade, inhibiting cell proliferation, migration and invasion, and promoting apoptosis (Li et al. Citation2020). lncRNA neuroblastoma associated transcript 1 (NBAT1) is downregulated in oxaliplatin-resistant colorectal cancer tissues, and NBAT1 is demonstrated to increase the level of WWC family member 3 (WWC3) by sponging miR-4504 (Li and Li Citation2022). Furthermore, this high level of WWC3 promotes LATS1 phosphorylation, suppressing cell proliferation and tumor growth of oxaliplatin-resistant colorectal cancer (Wennmann et al. Citation2014; Li and Li Citation2022). In addition, the overexpression of tumor-suppressive lncRNA on chromosome 8p12 (TSLNC8; also known as LINC00589) limits glioma progression by suppressing WWC3 through binding to miR-10b-5p, which activates the Hippo signaling pathway (Yang et al. Citation2020). lncRNA crystallin beta-gamma domain containing 3 (CRYBG3) interferes with mechanotransduction by inhibiting F-actin organization, which activates LATS1/2 and induces the nuclear exclusion of YAP/TAZ, thereby inhibiting lung cancer cell proliferation, invasion, and metastasis (Seo and Kim Citation2018; Zheng et al. Citation2022). Furthermore, lncRNA mitogen-activated protein kinase 8 interacting protein 1 pseudogene 2 (MAPK8IP1P2) suppresses the lymphatic metastasis of thyroid cancer by sponging miR-146b-3p and disturbing the inhibitory effects of miR-146b-3p on ras association domain family member 1/5 (RASSF1/5) and neurofibromin 2 (NF2) (Liu X et al. Citation2020). RASSF1/5 and NF2 are well-known tumor suppressors that promote the activations of MST1/2 and LATS1/2 (Bitra et al. Citation2017; Hong et al. Citation2020). Collectively, tumor-suppressive lncRNAs that activate MST1/2 and LATS1/2, the core kinases of the Hippo pathway through various mechanisms are downregulated in cancers.
3.2. Negative regulations of MST1/2 or LATS1/2 by lncRNAs
Other lncRNAs function as oncogenes by suppressing the activation of MST1/2 or LATS1/2. lncRNA myocardial infarction associated transcript (MIAT), which is overexpressed in breast cancer, is found to suppress DLG3 expression by binding to methylation proteins and subsequently to promote the methylation of CpG islands in DLG3 promoter (Li et al. Citation2020). Since DLG3 binds to MST2, activates LATS1, and inhibits the nuclear translocation of YAP, MIAT likely facilitates breast cancer progression by suppressing DLG3 expression (Liu, Li, Wang, et al. Citation2019; Li et al. Citation2020). lncRNA colorectal neoplasia differentially expressed (CRNDE) is reported to be significantly upregulated in hepatocellular carcinoma tissues and cell lines and to promote epigenetic repression of LATS2 mediated by EZH2, SUZ12, and SUV39H1 and consequently plays a pivotal role in the proliferation, migration, and chemoresistance of hepatocellular carcinoma cells (Xie et al. Citation2020). In addition, the overexpression of LINC-regulator of reprogramming (ROR) is shown to enhance the migration and invasion of pancreatic cancer cells, and a subsequent study found that LINC-ROR reduced the protein expressions of MST1/2, phospho-LATS1 (p-LATS1), p-MOB1, and p-YAP, which suggested that LINC-ROR inhibits the Hippo pathway to promote the nuclear localization of YAP (Chen W et al. Citation2020; Wang et al. Citation2023). Similarly, lncRNA gastric carcinoma proliferation enhancing transcript 1 (GHET1), which is upregulated in triple-negative breast cancer cells, significantly diminished the protein levels of MST1, p-LATS1, and p-YAP (Wang and Liu Citation2021). Meanwhile, certain lncRNAs repress the Hippo pathway by directly targeting adaptor proteins rather than the core kinases. lncRNA WEE2 antisense RNA 1 (WEE2-AS1) induces MOB1A degradation through the ubiquitin-proteasome pathway in colorectal cancer cells (Yang et al. Citation2022), and lncRNA HOX transcript antisense RNA (HOTAIR) disrupts the interaction between MST1/2 and SAV1 by directly binding to SAV1 protein in renal cell carcinoma cells (Hu et al. Citation2017). Both lncRNAs are overexpressed in cancers and promote cell proliferation and growth by inhibiting the Hippo pathway. Taken together, oncogenic lncRNAs that repress the kinase cascade of the Hippo pathway are overexpressed in cancer and promote tumor development and aggravation.
4. Regulations of YAP/TAZ complex or TEADs by lncRNAs in cancer
Unlike typical transcription factors, YAP and TAZ lack DNA-binding domains and, thus, must bind with other entities to become functional (Wei et al. Citation2023). Although several DNA binding proteins have been reported to interact with YAP and TAZ, evidence suggests that TEADs are their primary binding partners (Totaro et al. Citation2018). Transcriptional targets driven by TEADs include genes, such as c-MYC, Snail1/2, Slug, Twist1/2, and ZEB1/2, which play pivotal roles in cell growth, proliferation, metastasis, and maintaining tissue equilibrium (Yeung and Yang Citation2017; Huh et al. Citation2019; Huang et al. Citation2021). Recent studies have identified several kinases that, in addition to LATS1/2, can phosphorylate YAP and TAZ (Misra and Irvine Citation2018). For instance, Src kinases have been shown to directly phosphorylate YAP and TAZ at Tyr residues, thereby augmenting its activity (Lamar et al. Citation2019). Moreover, post-translational modifications have been reported to impact YAP activity, such as ubiquitination, sumoylation, and glycosylation (Varelas Citation2014). Although most TEADs interact with YAP/TAZ complex, phosphorylation of TEADs by p38 MAPK has been reported to reduce YAP/TAZ-dependent transcriptional activity (Lin et al. Citation2017). These findings suggest that specific YAP/TAZ or TEADs regulatory mechanisms operate independently of the Hippo signaling kinase cascade. Many studies have shown that lncRNAs are involved in these independent regulatory actions that influence cancer development and progression (). In this section, we highlight studies in which lncRNA dysregulation specifically modulates YAP/TAZ or TEADs to influence tumor development and progression.
Table 2. lncRNAs that regulate YAP/TAZ/TEAD in cancer.
4.1. Positive regulations of YAP/TAZ complex or TEADs by lncRNAs
Studies have shown that YAP/TAZ complex is widely activated in most human malignancies and plays crucial roles in tumorigenesis and malignancy (Zanconato et al. Citation2016). In this regard, lncRNAs that activate YAP/TAZ complex or their downstream transcription factors, such as TEADs, were identified as oncogenes. lncRNA metastasis associated lung adenocarcinoma transcript 1 (MALAT1), a well-studied lncRNA, is found to interact directly with YAP and increase its activity in ovarian cancer by maintaining its intranuclear localization and enhancing its stability and expression (Wu et al. Citation2020). Furthermore, MALAT1 is identified as a ceRNA that increases the expression of YAP by targeting miR-141-3p in malignant pleural mesothelioma (Wang P. et al. Citation2021). In each of these cancers, MALAT1 expression is upregulated and this upregulation enhances cell proliferation, migration, invasion, stemness, and chemoresistance and suppresses apoptosis (Wu et al. Citation2020; Wang et al. Citation2021). As an alternative mechanism, lncRNA RP11-323N12.5 enhances tumor growth and immunosuppression in gastric cancer by binding to c-MYC in the YAP promoter region, thereby facilitating the transcription of YAP mRNA (Wang et al. Citation2021). On the other hand, YAP is shown to upregulate MYC at the transcriptional and post-transcriptional levels in gastric cancer (Choi et al. Citation2018). Also, lncRNA cytoskeleton regulator RNA (CYTOR; also known as LINC00152) increases the aggressiveness of retinoblastoma by upregulating YAP and thus sponging miR-613 (Wang et al. Citation2020). In a similar way, CYTOR acts as a ceRNA for miR-125a-5p, and thus upregulates serum response factor (SRF) in tamoxifen-resistant breast cancer cell lines (Liu Y. et al. Citation2020). SRF is a transcription factor that regulates the expressions of several genes and increases TAZ expression (Liu et al. Citation2016). TAZ is also shown to be upregulated by surfactant associated 1, lncRNA (SFTA1P), which is overexpressed in non-small cell lung cancer and interacts with TAZ mRNA (Zhu et al. Citation2021). As an example of lncRNAs that activate TEAD, ASB16 antisense RNA 1 (ASB16-AS1) is shown to be overexpressed in colorectal cancer and to interact with miR-185-5p, which targets TEAD, and consequently promotes cell proliferation, migration, invasion, and stemness, while inhibiting apoptosis (Yu et al. Citation2022). Consequently, lncRNAs that activate YAP/TAZ complex or TEADs are commonly overexpressed in cancers, which suggest that they have oncogenic functions.
4.2. Negative regulation of YAP or TEADs by lncRNAs
In contrast to oncogenic lncRNAs, several lncRNAs have been shown to exert tumor-suppressive functions by inactivating YAP or TEADs (primarily YAP). lncRNA growth arrest-specific transcript 5 (GAS5) is found to attenuate colorectal cancer progression by interacting with the WW domain of YAP to promote its cytoplasmic translocation, phosphorylation, and ubiquitin-mediated degradation (Ni et al. Citation2019). Similarly, in endometrial cancer, GAS5 binds directly to YAP in tumor-associated macrophages (TAMs) and inhibits its nuclear accumulation, thereby promoting the transformation of TAMs into an antitumor phenotype (Tu et al. Citation2022). GAS5 expression is significantly reduced in both cancers, especially in colorectal cancer, and YAP targeted YTH N6-methyladenosine RNA binding protein F3 (YTHDF3) to induce m6A-modified GAS5 degradation (Ni et al. Citation2019; Tu et al. Citation2022). lncRNA mir-497-195 cluster host gene (MIR497HG), which is expressed at low levels in bladder cancer cell lines, appears to weaken YAP binding to SMAD3 through the production of miR-497 and miR-195 (Zhuang et al. Citation2020, Citation2021). This downregulates connective tissue growth factor (CTGF), which is known to promote tumor development, progression, and metastasis downstream of YAP/SMAD3 complex, suggesting that MIR497HG could inhibit the progression of bladder cancer (Zhuang et al. Citation2020; Shen et al. Citation2021; Zhuang et al. Citation2021). Concerning SMADs, LINC00968, which is downregulated in lung adenocarcinoma tissues, induces the accumulation of SMAD7 and suppresses YAP, and LINC00968 overexpression inhibits cell growth and metastasis by acting as a ceRNA for miR-21-5p, which targets SMAD7 (Zhu et al. Citation2020). In this regard, it has been suggested that SMAD7 might serve as a continuous reservoir or sequestration protein for YAP (Aragon et al. Citation2012). However, further experimental studies are needed to determine whether SMAD7 upregulation by LINC00968 in lung adenocarcinoma cells results in inhibitory binding by SMAD7 to YAP. Meanwhile, MALAT1, previously reported to be as an oncogenic lncRNA in several cancers, is shown to have the opposite function in breast cancer by inactivating TEAD. By binding directly to TEAD, MALAT1 prevents TEAD from binding to its co-activator, YAP, and target gene promoters (Kim et al. Citation2018). Furthermore, the expression of MALAT1 is negatively correlated with the ability of breast cancer to progress and metastasize. However, these findings are inconsistent with previous studies that suggest MALAT1 can act as a miRNA sponge to promote tumor growth and migration in breast cancer (Shih et al. Citation2021; Yue et al. Citation2021). Therefore, further research is warranted to confirm or refute these findings. As a result, tumor-suppressive lncRNAs that inactivate downstream effectors of the Hippo pathway are typically downregulated in cancer.
5. Clinical implications of regulation of the Hippo-YAP/TAZ signaling pathway by lncRNAs in cancer
lncRNAs that regulate cell growth, proliferation, and survival by modulating components of the Hippo-YAP/TAZ signaling pathway have also been shown to be associated with cancer prognosis and clinicopathological characteristics. For instance, higher levels of lncRNA GHET1 is found in non-small cell lung cancer tissues than in adjacent normal tissues, and this overexpression is associated with tumor node metastasis (TNM) stage, lymph node metastasis, and poor outcomes (Guan et al. Citation2018). lncRNA CRNDE, overexpressed in tumor samples from hepatocellular carcinoma patients, is also expressed at high levels in more advanced TNM stages and in case of lymph node metastasis. Also, CRNDE expression is negatively correlated with overall survival and disease-free survival. In other words, CRNDE is shown to have negative impacts on progression, metastasis, and prognosis in hepatocellular carcinoma (Xie et al. Citation2020). In contrast, the expression of lncRNA uc.134 is significantly downregulated in hepatocellular carcinoma tumor tissues compared to adjacent non-tumor tissues, and uc.134 positively regulates the expression of LATS1 by binding to CUL4A and inhibiting the CUL4A-mediated ubiquitination of LATS1. Furthermore, reduced lncRNA uc.134 expression is significantly correlated with poorer survival, lymph node metastasis, and TNM stage in hepatocellular carcinoma patients. LATS1 downregulation and YAP hypo-phosphorylation are associated with poorer overall survival (Ni et al. Citation2017). In addition, LincRNA-p21 (also known as TP53COR1) levels is significantly lower in gastric cancer cells and tissues than in normal cells and tissues, and the downregulation of LincRNA-p21 contributes to gastric cancer cell development by elevating YAP mRNA and protein levels, and is significantly associated with greater invasion depth, more distant metastasis, and an advanced TNM stage (Ni et al. Citation2017). These findings suggest that lncRNAs associated with the Hippo-YAP/TAZ pathway might be promising diagnostic and prognostic biomarkers in cancer.
Various anticancer drugs with diverse properties and targets are used for chemotherapy (Gottesman Citation2002; Kim et al. Citation2022), but drug resistance is a major obstacle to effective cancer treatment and results in more aggressive behavior, poorer clinical outcomes, metastatic progression, and recurrence (Kartal-Yandim et al. Citation2016; Lu et al. Citation2022). Recently, studies have indicated that the activation of YAP/TAZ complex, a key effector of the Hippo signaling pathway, enhances resistance to various anticancer therapies, including chemotherapy and radiotherapy (Kim and Kim Citation2017; Zhang et al. Citation2020; Wu et al. Citation2021). Several lncRNAs involved in the regulation of YAP and TAZ have been associated with drug resistance in various cancers. For example, the expression of lncRNA DLGAP1-AS2 increases progressively in squamous cell carcinoma cells after cisplatin exposure. DLGAP1-AS2 inhibits the binding of H3K27ac to the FAM3D enhancer region, thereby inhibiting FAM3D mRNA transcription and extracellular FAM3D protein secretion, and this inhibition of FAM3D interferes with its binding to Gαi protein-coupled receptor formyl peptide receptor 1/2 (FPR1/2) and leads to the activation of phospholipase D (PLD) (Nan et al. Citation2022). Furthermore, PLD activation facilitates the production of phosphatidic acids, which directly interact with LATS and NF2 and result in the nuclear translocation of YAP, which contributes to cisplatin resistance in squamous cell carcinoma cells (Han et al. Citation2018; Nan et al. Citation2022). Moreover, lncRNA MALAT1 is highly expressed in cisplatin-resistant ovarian cancer cells, and as described in the section above, MALAT1 increases cisplatin resistance in ovarian cancer cells by inhibiting the nucleus-to-cytoplasm translocation of YAP and increasing nuclear YAP levels (Wu et al. Citation2020). On the other hand, LINC01508 overexpression enhances the sensitivity of ovarian cancer cells to cisplatin by suppressing YAP expression and facilitating the cell cycle arrest and cell death (Xiao et al. Citation2021). Hypoxia-induced tumor-derived exosomal LINC-ROR promotes cell proliferation, stemness and gemcitabine resistance in pancreatic cancer by upregulating the phosphorylation of LATS1, MOB1, and YAP (Chen W et al. Citation2020; Wang et al. Citation2023). Furthermore, lncRNA CYTOR increases YAP expression in retinoblastoma cells and improved chemoresistance to carboplatin and adriamycin (Wang Ying et al. Citation2020). CYTOR also upregulates TAZ expression and is significantly elevated in tamoxifen-resistant breast cancer cell lines (Liu Y et al. Citation2020), suggesting that the consequently upregulated TAZ may cause tamoxifen-resistance in breast cancer (Liu, Li, Li, et al. Citation2019). Taken together, several lncRNAs contribute to various drug resistance mechanisms by regulating the expression or activation of YAP/TAZ and thereby influencing the efficacy of anticancer drugs.
Approximately 75% of patients with a solid malignant tumor receive radiotherapy with curative or palliative intent (Kang et al. Citation2018). Radiotherapy can be used alone or in combination with other cancer treatments, such as surgery or chemotherapy (Wolf et al. Citation1991; Forastiere et al. Citation2003; Kim et al. Citation2017; Kim W, Youn H, et al. Citation2018). Nonetheless, cancer recurrence and treatment failure still occur due to the development of radioresistance in cancer cells (Chen et al. Citation2017; Kim W, Son B, et al. Citation2018; Kim et al. Citation2019). lncRNAs associated with the transcriptional activity of YAP/TAZ complex have implications for radiotherapy. For example, radiation-induced lncRNA CRYBG3 mediates the cytoplasmic maintenance of YAP and TAZ, and thus inhibits the growth and metastasis of lung cancer cells and enhances the effectiveness of radiotherapy (Zheng et al. Citation2022). In colorectal cancer, MALAT1 enhances the nuclear translocation and transcriptional co-activation of YAP through a feedback loop involving ANKHD1 and YAP. Furthermore, activated YAP promotes the activation of AKT and upregulates MRE11, which results in the induction of DNA damage repair and radioresistance in colorectal cancer (Yao et al. Citation2022). In summary, regulation of the transcriptional activity of YAP/TAZ complex by lncRNAs affects the effectiveness of radiation therapy and suggests that lncRNAs may be potential therapeutic targets for radioresistance.
6. Conclusions
The dysregulation of lncRNAs that regulate the Hippo-YAP/TAZ signaling pathway is implicated in the pathogenesis and progression of multiple malignancies as it confers chemo- and radioresistance. Because mutations are uncommon in the Hippo-YAP/TAZ pathway, it is helpful to identify regulatory factors for the pathway in cancer environment and associated mutations. lncRNAs that modulate the Hippo-YAP/TAZ pathway have emerged as promising targets for cancer diagnosis, prognosis, and treatment, and techniques that utilize antisense oligonucleotides, RNA interference, vectors, and CRISPR/Cas9 systems offer potential means of developing lncRNA-targeted therapeutic strategies (Bhan et al. Citation2017; Roh, Kim, et al. Citation2023). However, crosstalk between lncRNAs and signaling pathways such as Wnt, TGF-β, and EGFR, which contribute to cancer in association with the Hippo-YAP/TAZ pathway, requires further exploration (Saito and Nagase Citation2015; Lee et al. Citation2018; Zinatizadeh et al. Citation2021). Furthermore, more studies are needed to determine the clinical feasibility of these lncRNAs. Therefore, it is important to systematically classify oncogenic or tumor-suppressive lncRNAs that modulate the Hippo-YAP/TAZ pathway and to determine their impacts on treatment resistance. This approach will undoubtedly provide valuable clues that guide pharmacological studies.
Disclosure statement
No potential conflict of interest was reported by the author(s).
References
- Angus L, Moleirinho S, Herron L, Sinha A, Zhang X, Niestrata M, Dholakia K, Prystowsky MB, Harvey KF, Reynolds PA, et al. 2012. Willin/FRMD6 expression activates the Hippo signaling pathway kinases in mammals and antagonizes oncogenic YAP. Oncogene. 31(2):238–250. doi: 10.1038/onc.2011.224.
- Aragon E, Goerner N, Xi Q, Gomes T, Gao S, Massague J, Macias MJ. 2012. Structural basis for the versatile interactions of Smad7 with regulator WW domains in TGF-beta pathways. Structure. 20(10):1726–1736. doi: 10.1016/j.str.2012.07.014.
- Avruch J, Zhou D, Fitamant J, Bardeesy N, Mou F, Barrufet LR. 2012. Protein kinases of the Hippo pathway: regulation and substrates. Semin Cell Dev Biol. 23(7):770–784. doi: 10.1016/j.semcdb.2012.07.002.
- Bhan A, Soleimani M, Mandal SS. 2017. Long noncoding RNA and cancer: a New paradigm. Cancer Res. 77(15):3965–3981. doi: 10.1158/0008-5472.CAN-16-2634.
- Bitra A, Sistla S, Mariam J, Malvi H, Anand R. 2017. Rassf proteins as modulators of Mst1 kinase activity. Sci Rep. 7:45020. doi: 10.1038/srep45020.
- Calses PC, Crawford JJ, Lill JR, Dey A. 2019. Hippo pathway in cancer: aberrant regulation and therapeutic opportunities. Trends Cancer. 5(5):297–307. doi: 10.1016/j.trecan.2019.04.001.
- Chae Y, Roh J, Kim W. 2021. The roles played by long non-coding RNAs in glioma resistance. Int J Mol Sci. 22:13.
- Chan JJ, Tay Y. 2018. Noncoding RNA:RNA regulatory networks in cancer. Int J Mol Sci. 19:5.
- Chan LH, Wang W, Yeung W, Deng Y, Yuan P, Mak KK. 2014. Hedgehog signaling induces osteosarcoma development through Yap1 and H19 overexpression. Oncogene. 33(40):4857–4866. doi: 10.1038/onc.2013.433.
- Chen C, Qin L, Xiao MF. 2022. Long noncoding RNA LOC554202 predicts a poor prognosis and correlates with immune infiltration in thyroid cancer. Comput Math Methods Med. 2022:3585626.
- Chen FB, Wu P, Zhou R, Yang QX, Zhang X, Wang RR, Qi SC, Yang X. 2020. LINC01315 impairs microRNA-211-dependent DLG3 downregulation to inhibit the development of oral squamous cell carcinoma. Front Oncol. 10:556084. doi: 10.3389/fonc.2020.556084.
- Chen GZ, Zhu HC, Dai WS, Zeng XN, Luo JH, Sun XC. 2017. The mechanisms of radioresistance in esophageal squamous cell carcinoma and current strategies in radiosensitivity. J Thorac Dis. 9(3):849–859. doi: 10.21037/jtd.2017.03.23.
- Chen K, Wang X, Wei B, Sun R, Wu C, Yang HJ. 2022. LncRNA SNHG6 promotes glycolysis reprogramming in hepatocellular carcinoma by stabilizing the BOP1 protein. Anim Cells Syst. 26(6):369–379. doi: 10.1080/19768354.2022.2134206.
- Chen W, Wang H, Liu Y, Xu W, Ling C, Li Y, Liu J, Chen M, Zhang Y, Chen B, et al. 2020. Linc-RoR promotes proliferation, migration, and invasion via the Hippo/YAP pathway in pancreatic cancer cells. J Cell Biochem. 121(1):632–641. doi: 10.1002/jcb.29308.
- Cheng J, Wang S, Dong Y, Yuan Z. 2020. The role and regulatory mechanism of Hippo signaling components in the neuronal system. Front Immunol. 11:281. doi: 10.3389/fimmu.2020.00281.
- Choi W, Kim J, Park J, Lee DH, Hwang D, Kim JH, Ashktorab H, Smoot D, Kim SY, Choi C, et al. 2018. YAP/TAZ initiates gastric tumorigenesis via upregulation of MYC. Cancer Res. 78(12):3306–3320. doi: 10.1158/0008-5472.CAN-17-3487.
- Cornils H, Kohler RS, Hergovich A, Hemmings BA. 2011. Downstream of human NDR kinases: impacting on c-myc and p21 protein stability to control cell cycle progression. Cell Cycle. 10(12):1897–1904. doi: 10.4161/cc.10.12.15826.
- Cunningham R, Hansen CG. 2022. The Hippo pathway in cancer: YAP/TAZ and TEAD as therapeutic targets in cancer. Clin Sci. 136(3):197–222. doi: 10.1042/CS20201474.
- Currey L, Thor S, Piper M. 2021. TEAD family transcription factors in development and disease. Development. 148:12. doi: 10.1242/dev.196675.
- de Amorim ISS, de Sousa Rodrigues MM, Mencalha AL. 2021. The tumor suppressor role of salvador family WW domain-containing protein 1 (SAV1): one of the key pieces of the tumor puzzle. J Cancer Res Clin Oncol. 147(5):1287–1297. doi: 10.1007/s00432-021-03552-3.
- Derrien T, Johnson R, Bussotti G, Tanzer A, Djebali S, Tilgner H, Guernec G, Martin D, Merkel A, Knowles DG, et al. 2012. The GENCODE v7 catalog of human long noncoding RNAs: analysis of their gene structure, evolution, and expression. Genome Res. 22(9):1775–1789. doi: 10.1101/gr.132159.111.
- Dey A, Varelas X, Guan KL. 2020. Targeting the Hippo pathway in cancer, fibrosis, wound healing and regenerative medicine. Nat Rev Drug Discov. 19(7):480–494. doi: 10.1038/s41573-020-0070-z.
- Do H, Kim D, Kang J, Son B, Seo D, Youn H, Youn B, Kim W. 2019. TFAP2C increases cell proliferation by downregulating GADD45B and PMAIP1 in non-small cell lung cancer cells. Biol Res. 52(1):35. doi: 10.1186/s40659-019-0244-5.
- Do H, Kim W. 2018. Roles of oncogenic long non-coding RNAs in cancer development. Genomics Inform. 16(4):e18. doi: 10.5808/GI.2018.16.4.e18.
- Ehmer U, Sage J. 2016. Control of proliferation and cancer growth by the Hippo signaling pathway. Mol Cancer Res. 14(2):127–140. doi: 10.1158/1541-7786.MCR-15-0305.
- Forastiere AA, Goepfert H, Maor M, Pajak TF, Weber R, Morrison W, Glisson B, Trotti A, Ridge JA, Chao C, et al. 2003. Concurrent chemotherapy and radiotherapy for organ preservation in advanced laryngeal cancer. N Engl J Med. 349(22):2091–2098. doi: 10.1056/NEJMoa031317.
- Fu M, Hu Y, Lan T, Guan KL, Luo T, Luo M. 2022. The Hippo signalling pathway and its implications in human health and diseases. Signal Transduct Target Ther. 7(1):376. doi: 10.1038/s41392-022-01191-9.
- Galan JA, Avruch J. 2016. MST1/MST2 protein kinases: regulation and physiologic roles. Biochemistry. 55(39):5507–5519. doi: 10.1021/acs.biochem.6b00763.
- Gottesman MM. 2002. Mechanisms of cancer drug resistance. Annu Rev Med. 53:615–627. doi: 10.1146/annurev.med.53.082901.103929.
- Guan ZB, Cao YS, Li Y, Tong WN, Zhuo AS. 2018. Knockdown of lncRNA GHET1 suppresses cell proliferation, invasion and LATS1/YAP pathway in non small cell lung cancer. Cancer Biomark. 21(3):557–563. doi: 10.3233/CBM-170431.
- Gundogdu R, Hergovich A. 2019. MOB (Mps one binder) proteins in the Hippo pathway and cancer. Cells. 8:6. doi: 10.3390/cells8060569.
- Halder G, Johnson RL. 2011. Hippo signaling: growth control and beyond. Development. 138(1):9–22. doi: 10.1242/dev.045500.
- Han H, Qi R, Zhou JJ, Ta AP, Yang B, Nakaoka HJ, Seo G, Guan KL, Luo R, Wang W. 2018. Regulation of the Hippo pathway by phosphatidic acid-mediated lipid-protein interaction. Mol Cell. 72(2):328–340.e328. doi: 10.1016/j.molcel.2018.08.038.
- Hanahan D, Weinberg RA. 2011. Hallmarks of cancer: the next generation. Cell. 144(5):646–674. doi: 10.1016/j.cell.2011.02.013.
- Heng BC, Zhang X, Aubel D, Bai Y, Li X, Wei Y, Fussenegger M, Deng X. 2021. An overview of signaling pathways regulating YAP/TAZ activity. Cell Mol Life Sci. 78(2):497–512. doi: 10.1007/s00018-020-03579-8.
- Hergovich A, Hemmings BA. 2009. Mammalian NDR/LATS protein kinases in hippo tumor suppressor signaling. Biofactors. 35(4):338–345. doi: 10.1002/biof.47.
- Hong AW, Meng Z, Plouffe SW, Lin Z, Zhang M, Guan KL. 2020. Critical roles of phosphoinositides and NF2 in Hippo pathway regulation. Genes Dev. 34(7-8):511–525. doi: 10.1101/gad.333435.119.
- Hu G, Dong B, Zhang J, Zhai W, Xie T, Huang B, Huang C, Yao X, Zheng J, Che J, et al. 2017. The long noncoding RNA HOTAIR activates the Hippo pathway by directly binding to SAV1 in renal cell carcinoma. Oncotarget. 8(35):58654–58667. doi: 10.18632/oncotarget.17414.
- Huang Z, Yan Y, Tang P, Cai J, Cao X, Wang Z, Zhang F, Shen B. 2021. TEAD4 as a prognostic marker promotes cell migration and invasion of urinary bladder cancer via EMT. Onco Targets Ther. 14:937–949. doi: 10.2147/OTT.S290425.
- Huh HD, Kim DH, Jeong HS, Park HW. 2019. Regulation of TEAD transcription factors in cancer biology. Cells. 8:6.
- Hulvat MC. 2020. Cancer incidence and trends. Surg Clin North Am. 100(3):469–481. doi: 10.1016/j.suc.2020.01.002.
- Kang J, Kim W, Seo H, Kim E, Son B, Lee S, Park G, Jo S, Moon C, Youn H, et al. 2018. Radiation-induced overexpression of transthyretin inhibits retinol-mediated hippocampal neurogenesis. Sci Rep. 8(1):8394. doi: 10.1038/s41598-018-26762-1.
- Kapranov P, Cheng J, Dike S, Nix DA, Duttagupta R, Willingham AT, Stadler PF, Hertel J, Hackermuller J, Hofacker IL, et al. 2007. RNA maps reveal new RNA classes and a possible function for pervasive transcription. Science. 316(5830):1484–1488. doi: 10.1126/science.1138341.
- Kartal-Yandim M, Adan-Gokbulut A, Baran Y. 2016. Molecular mechanisms of drug resistance and its reversal in cancer. Crit Rev Biotechnol. 36(4):716–726. doi: 10.3109/07388551.2015.1015957.
- Kim J, Piao HL, Kim BJ, Yao F, Han Z, Wang Y, Xiao Z, Siverly AN, Lawhon SE, Ton BN, et al. 2018. Long noncoding RNA MALAT1 suppresses breast cancer metastasis. Nat Genet. 50(12):1705–1715. doi: 10.1038/s41588-018-0252-3.
- Kim MH, Kim J. 2017. Role of YAP/TAZ transcriptional regulators in resistance to anti-cancer therapies. Cell Mol Life Sci. 74(8):1457–1474. doi: 10.1007/s00018-016-2412-x.
- Kim S, Lim SW, Choi J. 2022. Drug discovery inspired by bioactive small molecules from nature. Anim Cells Syst (Seoul). 26(6):254–265. doi: 10.1080/19768354.2022.2157480.
- Kim W, Jho EH. 2018. The history and regulatory mechanism of the Hippo pathway. BMB Rep. 51(3):106–118. doi: 10.5483/BMBRep.2018.51.3.022.
- Kim W, Kang J, Lee S, Youn B. 2017. Effects of traditional oriental medicines as anti-cytotoxic agents in radiotherapy. Oncol Lett. 13(6):4593–4601. doi: 10.3892/ol.2017.6042.
- Kim W, Lee S, Seo D, Kim D, Kim K, Kim E, Kang J, Seong KM, Youn H, Youn B. 2019. Cellular stress responses in radiotherapy. Cells. 8:9.
- Kim W, Son B, Lee S, Do H, Youn B. 2018. Targeting the enzymes involved in arachidonic acid metabolism to improve radiotherapy. Cancer Metastasis Rev. 37(2-3):213–225. doi: 10.1007/s10555-018-9742-0.
- Kim W, Youn H, Lee S, Kim E, Kim D, Sub Lee J, Lee JM, Youn B. 2018. RNF138-mediated ubiquitination of rpS3 is required for resistance of glioblastoma cells to radiation-induced apoptosis. Exp Mol Med. 50(1):e434. doi: 10.1038/emm.2017.247.
- Kontomanolis E N, Syllaios A, Schizas D, Kalagasidou S, Pagkalos A, Alatzidou D, Kantari P, Ntounis T, Fasoulakis Z. 2021. Basic principles of molecular biology of cancer cell-molecular cancer indicators. J Buon. 26(5):1723–1734.
- Lamar JM, Xiao Y, Norton E, Jiang ZG, Gerhard GM, Kooner S, Warren JSA, Hynes RO. 2019. SRC tyrosine kinase activates the YAP/TAZ axis and thereby drives tumor growth and metastasis. J Biol Chem. 294(7):2302–2317. doi: 10.1074/jbc.RA118.004364.
- Lee Y, Kim NH, Cho ES, Yang JH, Cha YH, Kang HE, Yun JS, Cho SB, Lee SH, Paclikova P, et al. 2018. Dishevelled has a YAP nuclear export function in a tumor suppressor context-dependent manner. Nat Commun. 9(1):2301. doi: 10.1038/s41467-018-04757-w.
- Li C, Li X. 2022. Antitumor activity of lncRNA NBAT-1 via inhibition of miR-4504 to target to WWC3 in oxaliplatin-resistant colorectal carcinoma. J Healthc Eng. 2022:9121554.
- Li D, Hu X, Yu S, Deng S, Yan M, Sun F, Song J, Tang L. 2020. Silence of lncRNA MIAT-mediated inhibition of DLG3 promoter methylation suppresses breast cancer progression via the Hippo signaling pathway. Cell Signal. 73:109697. doi: 10.1016/j.cellsig.2020.109697.
- Li P, Wang J, Zhi L, Cai F. 2021. Linc00887 suppresses tumorigenesis of cervical cancer through regulating the miR-454-3p/FRMD6-Hippo axis. Cancer Cell Int. 21(1):33. doi: 10.1186/s12935-020-01730-w.
- Li S, Yu Z, Chen SS, Li F, Lei CY, Chen XX, Bao JM, Luo Y, Lin GZ, Pang SY, et al. 2015. The YAP1 oncogene contributes to bladder cancer cell proliferation and migration by regulating the H19 long noncoding RNA. Urol Oncol. 33(10):427.e1–427.e10.
- Lin KC, Moroishi T, Meng Z, Jeong HS, Plouffe SW, Sekido Y, Han J, Park HW, Guan KL. 2017. Regulation of Hippo pathway transcription factor TEAD by p38 MAPK-induced cytoplasmic translocation. Nat Cell Biol. 19(8):996–1002. doi: 10.1038/ncb3581.
- Liu CY, Chan SW, Guo F, Toloczko A, Cui L, Hong W. 2016. MRTF/SRF dependent transcriptional regulation of TAZ in breast cancer cells. Oncotarget. 7(12):13706–13716. doi: 10.18632/oncotarget.7333.
- Liu J, Li J, Li P, Jiang Y, Chen H, Wang R, Cao F, Liu P. 2019. DLG5 suppresses breast cancer stem cell-like characteristics to restore tamoxifen sensitivity by inhibiting TAZ expression. J Cell Mol Med. 23(1):512–521. doi: 10.1111/jcmm.13954.
- Liu J, Li P, Wang R, Li J, Zhang M, Song Z, Liu P. 2019. High expression of DLG3 is associated with decreased survival from breast cancer. Clin Exp Pharmacol Physiol. 46(10):937–943. doi: 10.1111/1440-1681.13132.
- Liu X, Fu Q, Bian X, Fu Y, Xin J, Liang N, Li S, Zhao Y, Fang L, Li C, et al. 2020. Long Non-coding RNA MAPK8IP1P2 inhibits lymphatic metastasis of thyroid cancer by activating Hippo signaling via sponging miR-146b-3p. Front Oncol. 10:600927. doi: 10.3389/fonc.2020.600927.
- Liu Y, Li M, Yu H, Piao H. 2020. lncRNA CYTOR promotes tamoxifen resistance in breast cancer cells via sponging miR-125a-5p. Int J Mol Med. 45(2):497–509.
- Lu X, Wu Y, Cao R, Yu X, Gong J. 2022. CXCL12 secreted by pancreatic stellate cells accelerates gemcitabine resistance of pancreatic cancer by enhancing glycolytic reprogramming. Anim Cells Syst (Seoul). 26(4):148–157. doi: 10.1080/19768354.2022.2091019.
- Ma S, Meng Z, Chen R, Guan KL. 2019. The Hippo pathway: biology and pathophysiology. Annu Rev Biochem. 88:577–604. doi: 10.1146/annurev-biochem-013118-111829.
- Meng Z, Moroishi T, Guan KL. 2016. Mechanisms of Hippo pathway regulation. Genes Dev. 30(1):1–17. doi: 10.1101/gad.274027.115.
- Mercer TR, Dinger ME, Mattick JS. 2009. Long non-coding RNAs: insights into functions. Nat Rev Genet. 10(3):155–159. doi: 10.1038/nrg2521.
- Misra JR, Irvine KD. 2018. The Hippo signaling network and its biological functions. Annu Rev Genet. 52:65–87. doi: 10.1146/annurev-genet-120417-031621.
- Mo JS, Park HW, Guan KL. 2014. The Hippo signaling pathway in stem cell biology and cancer. EMBO Rep. 15(6):642–656. doi: 10.15252/embr.201438638.
- Mohajan S, Jaiswal PK, Vatanmakarian M, Yousefi H, Sankaralingam S, Alahari SK, Koul S, Koul HK. 2021. Hippo pathway: regulation, deregulation and potential therapeutic targets in cancer. Cancer Lett. 507:112–123. doi: 10.1016/j.canlet.2021.03.006.
- Nan Y, Luo Q, Wu X, Liu S, Zhao P, Chang W, Zhou A, Liu Z. 2022. DLGAP1-AS2-Mediated phosphatidic acid synthesis activates YAP signaling and confers chemoresistance in squamous cell carcinoma. Cancer Res. 82(16):2887–2903. doi: 10.1158/0008-5472.CAN-22-0717.
- Ni W, Yao S, Zhou Y, Liu Y, Huang P, Zhou A, Liu J, Che L, Li J. 2019. Long noncoding RNA GAS5 inhibits progression of colorectal cancer by interacting with and triggering YAP phosphorylation and degradation and is negatively regulated by the m(6)A reader YTHDF3. Mol Cancer. 18(1):143. doi: 10.1186/s12943-019-1079-y.
- Ni W, Zhang Y, Zhan Z, Ye F, Liang Y, Huang J, Chen K, Chen L, Ding Y. 2017. A Novel lncRNA uc.134 represses hepatocellular carcinoma progression by inhibiting CUL4A-mediated ubiquitination of LATS1. J Hematol Oncol. 10(1):91.
- Papait R, Kunderfranco P, Stirparo GG, Latronico MV, Condorelli G. 2013. Long noncoding RNA: a new player of heart failure? J Cardiovasc Transl Res. 6(6):876–883. doi: 10.1007/s12265-013-9488-6.
- Park JH, Pyun WY, Park HW. 2020. Cancer metabolism: phenotype, signaling and therapeutic targets. Cells. 9:10.
- Piccolo S, Dupont S, Cordenonsi M. 2014. The biology of YAP/TAZ: hippo signaling and beyond. Physiol Rev. 94(4):1287–1312. doi: 10.1152/physrev.00005.2014.
- Plouffe SW, Hong AW, Guan KL. 2015. Disease implications of the Hippo/YAP pathway. Trends Mol Med. 21(4):212–222. doi: 10.1016/j.molmed.2015.01.003.
- Pocaterra A, Romani P, Dupont S. 2020. YAP/TAZ functions and their regulation at a glance. J Cell Sci. 133:2. doi: 10.1242/jcs.230425.
- Roh J, Im M, Chae Y, Kang J, Kim W. 2022. The involvement of long Non-coding RNAs in glutamine-metabolic reprogramming and therapeutic resistance in cancer. Int J Mol Sci. 23:23.
- Roh J, Im M, Kang J, Youn B, Kim W. 2023. Long non-coding RNA in glioma: novel genetic players in temozolomide resistance. Anim Cells Syst (Seoul. 27(1):19–28. doi: 10.1080/19768354.2023.2175497.
- Roh J, Kim B, Im M, Jang W, Chae Y, Kang J, Youn B, Kim W. 2023. MALAT1-regulated gene expression profiling in lung cancer cell lines. BMC Cancer. 23(1):818. doi: 10.1186/s12885-023-11347-7.
- Saito A, Nagase T. 2015. Hippo and TGF-beta interplay in the lung field. Am J Physiol Lung Cell Mol Physiol. 309(8):L756–L767. doi: 10.1152/ajplung.00238.2015.
- Sanchez-Vega F, Mina M, Armenia J, Chatila WK, Luna A, La KC, Dimitriadoy S, Liu DL, Kantheti HS, Saghafinia S, et al. 2018. Oncogenic signaling pathways in The cancer genome atlas. Cell. 173(2):321–337.e310. doi: 10.1016/j.cell.2018.03.035.
- Seo D, Kim D, Chae Y, Kim W. 2020. The ceRNA network of lncRNA and miRNA in lung cancer. Genomics Inform. 18(4):e36. doi: 10.5808/GI.2020.18.4.e36.
- Seo D, Kim D, Kim W. 2019. Long non-coding RNA linc00152 acting as a promising oncogene in cancer progression. Genomics Inform. 17(4):e36. doi: 10.5808/GI.2019.17.4.e36.
- Seo D, Roh J, Chae Y, Kim W. 2021. Gene expression profiling after LINC00472 overexpression in an NSCLC cell line1. Cancer Biomark. 32(2):175–188. doi: 10.3233/CBM-210242.
- Seo J, Kim J. 2018. Regulation of Hippo signaling by actin remodeling. BMB Rep. 51(3):151–156. doi: 10.5483/BMBRep.2018.51.3.012.
- Shen M, Su Y, Song S, Liu D, Liu Z, Chen D, Pan Y, Zhang L, Xu X. 2023. SNHG14 facilitates cell proliferation in colorectal cancer through targeting KRAS via Hippo-YAP signaling. Cell Mol Biol (Noisy-le-Grand). 69(3):64–68. doi: 10.14715/cmb/2023.69.3.8.
- Shen YW, Zhou YD, Chen HZ, Luan X, Zhang WD. 2021. Targeting CTGF in cancer: an emerging therapeutic opportunity. Trends Cancer. 7(6):511–524. doi: 10.1016/j.trecan.2020.12.001.
- Shih CH, Chuang LL, Tsai MH, Chen LH, Chuang EY, Lu TP, Lai LC. 2021. Hypoxia-Induced MALAT1 promotes the proliferation and migration of breast cancer cells by sponging MiR-3064-5p. Front Oncol. 11:658151. doi: 10.3389/fonc.2021.658151.
- Siegel RL, Miller KD, Fuchs HE, Jemal A. 2022. Cancer Statistics, 2022. CA Cancer J Clin. 72(1):7–33.
- Slack FJ, Chinnaiyan AM. 2019. The role of Non-coding RNAs in oncology. Cell. 179(5):1033–1055. doi: 10.1016/j.cell.2019.10.017.
- Soltani R, Amini M, Mazaheri Moghaddam M, Jebelli A, Ahmadiyan S, Bidar N, Baradaran B, MotieGhader H, Asadi M, Mokhtarzadeh A. 2022. LncRNA DLGAP1-AS2 overexpression associates with gastric tumorigenesis: a promising diagnostic and therapeutic target. Mol Biol Rep. 49(7):6817–6826. doi: 10.1007/s11033-021-07038-w.
- Son B, Lee S, Youn H, Kim E, Kim W, Youn B. 2017. The role of tumor microenvironment in therapeutic resistance. Oncotarget. 8(3):3933–3945. doi: 10.18632/oncotarget.13907.
- Sun W, Shi Q, Zhang H, Yang K, Ke Y, Wang Y, Qiao L. 2019. Advances in the techniques and methodologies of cancer gene therapy. Discov Med. 27(146):45–55.
- Torre LA, Siegel RL, Ward EM, Jemal A. 2016. Global cancer incidence and mortality rates and trends–An update. Cancer Epidemiol Biomarkers Prev. 25(1):16–27. doi: 10.1158/1055-9965.EPI-15-0578.
- Totaro A, Panciera T, Piccolo S. 2018. YAP/TAZ upstream signals and downstream responses. Nat Cell Biol. 20(8):888–899. doi: 10.1038/s41556-018-0142-z.
- Tu J, Tan X, Chen Y, Chen Y, Li Z, Zhang Y, Chen X, Yang H, Chen H, Yu Z. 2022. Growth arrest-specific transcript 5 represses endometrial cancer development by promoting antitumor function of tumor-associated macrophages. Cancer Sci. 113(8):2496–2512. doi: 10.1111/cas.15390.
- Vaghari-Tabari M, Ferns GA, Qujeq D, Andevari AN, Sabahi Z, Moein S. 2021. Signaling, metabolism, and cancer: an important relationship for therapeutic intervention. J Cell Physiol. 236(8):5512–5532. doi: 10.1002/jcp.30276.
- Varelas X. 2014. The Hippo pathway effectors TAZ and YAP in development, homeostasis and disease. Development. 141(8):1614–1626. doi: 10.1242/dev.102376.
- Wang H, Min J, Xu C, Liu Y, Yu Z, Gong A, Xu M. 2023. Hypoxia-elicited exosomes promote the chemoresistance of pancreatic cancer cells by transferring LncROR via hippo signaling. J Cancer. 14(6):1075–1087. doi: 10.7150/jca.81320.
- Wang J, Huang F, Shi Y, Zhang Q, Xu S, Yao Y, Jiang R. 2021. RP11-323N12.5 promotes the malignancy and immunosuppression of human gastric cancer by increasing YAP1 transcription. Gastric Cancer. 24(1):85–102. doi: 10.1007/s10120-020-01099-9.
- Wang P, Bai C, Shen S, Jiang C, Deng J, Han D. 2021. MALAT1 promotes malignant pleural mesothelioma by sponging miR-141-3p. Open Med (Wars). 16(1):1653–1667. doi: 10.1515/med-2021-0383.
- Wang Y, Jia A, Cao Y, Hu X, Wang Y, Yang Q, Bi Y, Liu G. 2020. Hippo kinases MST1/2 regulate immune cell functions in cancer, infection, and autoimmune diseases. Crit Rev Eukaryot Gene Expr. 30(5):427–442. doi: 10.1615/CritRevEukaryotGeneExpr.2020035775.
- Wang Y, Liu S. 2021. LncRNA GHET1 promotes hypoxia-induced glycolysis, proliferation, and invasion in triple-negative breast cancer through the Hippo/YAP signaling pathway. Front Cell Dev Biol. 9:643515. doi: 10.3389/fcell.2021.643515.
- Wang Y, Xin D, Zhou L. 2020. LncRNA LINC00152 increases the aggressiveness of human retinoblastoma and enhances carboplatin and Adriamycin resistance by regulating MiR-613/Yes-associated protein 1 (YAP1) axis. Med Sci Monit. 26.
- Wei Y, Hui VLZ, Chen Y, Han R, Han X, Guo Y. 2023. YAP/TAZ: molecular pathway and disease therapy. MedComm. 4(4):e340.
- Wennmann DO, Schmitz J, Wehr MC, Krahn MP, Koschmal N, Gromnitza S, Schulze U, Weide T, Chekuri A, Skryabin BV, et al. 2014. Evolutionary and molecular facts link the WWC protein family to Hippo signaling. Mol Biol Evol. 31(7):1710–1723. doi: 10.1093/molbev/msu115.
- Wolf GT, Fisher SG, Hong WK, Hillman R, Spaulding M, Laramore GE, Endicott JW, McClatchey K, Henderson WG. 1991. Induction chemotherapy plus radiation compared with surgery plus radiation in patients with advanced laryngeal cancer. N Engl J Med. 324(24):1685–1690. doi: 10.1056/NEJM199106133242402.
- Wu Q, Guo J, Liu Y, Zheng Q, Li X, Wu C, Fang D, Chen X, Ma L, Xu P, et al. 2021. YAP drives fate conversion and chemoresistance of small cell lung cancer. Sci Adv. 7(40):eabg1850.
- Wu X, Wang Y, Zhong W, Cheng H, Tian Z. 2020. The long Non-coding RNA MALAT1 enhances ovarian cancer cell stemness by inhibiting YAP translocation from nucleus to cytoplasm. Med Sci Monit. 26:e922012.
- Xiao L, Shi XY, Li ZL, Li M, Zhang MM, Yan SJ, Wei ZL. 2021. Downregulation of LINC01508 contributes to cisplatin resistance in ovarian cancer via the regulation of the Hippo-YAP pathway. J Gynecol Oncol. 32(5):e77. doi: 10.3802/jgo.2021.32.e77.
- Xie SC, Zhang JQ, Jiang XL, Hua YY, Xie SW, Qin YA, Yang YJ. 2020. LncRNA CRNDE facilitates epigenetic suppression of CELF2 and LATS2 to promote proliferation, migration and chemoresistance in hepatocellular carcinoma. Cell Death Dis. 11(8):676. doi: 10.1038/s41419-020-02853-8.
- Yang P, Zhang D, Wang T, Ji J, Jin C, Peng C, Tan Y, Zhou J, Wang L, Feng Y, et al. 2022. CAF-derived exosomal WEE2-AS1 facilitates colorectal cancer progression via promoting degradation of MOB1A to inhibit the Hippo pathway. Cell Death Dis. 13(9):796. doi: 10.1038/s41419-022-05240-7.
- Yang Y, Liu X, Zheng J, Xue Y, Liu L, Ma J, Wang P, Yang C, Wang D, Shao L, et al. 2020. Interaction of BACH2 with FUS promotes malignant progression of glioma cells via the TSLNC8-miR-10b-5p-WWC3 pathway. Mol Oncol. 14(11):2936–2959. doi: 10.1002/1878-0261.12795.
- Yao PA, Wu Y, Zhao K, Li Y, Cao J, Xing C. 2022. The feedback loop of ANKHD1/lncRNA MALAT1/YAP1 strengthens the radioresistance of CRC by activating YAP1/AKT signaling. Cell Death Dis. 13(2):103. doi: 10.1038/s41419-022-04554-w.
- Yao RW, Wang Y, Chen LL. 2019. Cellular functions of long noncoding RNAs. Nat Cell Biol. 21(5):542–551. doi: 10.1038/s41556-019-0311-8.
- Yeung KT, Yang J. 2017. Epithelial-mesenchymal transition in tumor metastasis. Mol Oncol. 11(1):28–39. doi: 10.1002/1878-0261.12017.
- Yu M, Shi C, Xu D, Lin X, Ji T, Shi Z, Zhuge X, Zhuo S, Yang Q. 2022. LncRNA ASB16-AS1 drives proliferation, migration, and invasion of colorectal cancer cells through regulating miR-185-5p/TEAD1 axis. Cell Cycle. 21(1):1–11. doi: 10.1080/15384101.2021.1973700.
- Yue X, Wu WY, Dong M, Guo M. 2021. LncRNA MALAT1 promotes breast cancer progression and doxorubicin resistance via regulating miR-570-3p. Biomed J. 44(6 Suppl 2):S296–S304.
- Zanconato F, Cordenonsi M, Piccolo S. 2016. YAP/TAZ at the roots of cancer. Cancer Cell. 29(6):783–803. doi: 10.1016/j.ccell.2016.05.005.
- Zhang Z, Qiu N, Yin J, Zhang J, Liu H, Guo W, Liu M, Liu T, Chen D, Luo K, et al. 2020. SRGN crosstalks with YAP to maintain chemoresistance and stemness in breast cancer cells by modulating HDAC2 expression. Theranostics. 10(10):4290–4307. doi: 10.7150/thno.41008.
- Zheng L, Luo C, Yang N, Pei H, Ji M, Shu Y, Zhang Z, Dong S, Wang X, Li X, et al. 2022. Ionizing radiation-induced long noncoding RNA CRYBG3 regulates YAP/TAZ through mechanotransduction. Cell Death Dis. 13(3):209. doi: 10.1038/s41419-022-04650-x.
- Zhou Y, Jin Q, Chang J, Zhao Z, Sun C. 2022. Correction to: long non-coding RNA ZMIZ1-AS1 promotes osteosarcoma progression by stabilization of ZMIZ1. Cell Biol Toxicol.
- Zhu B, Finch-Edmondson M, Leong KW, Zhang X VM, Lin QXX, Lee Y, Ng WT, Guo H, Wan Y, et al. 2021. LncRNA SFTA1P mediates positive feedback regulation of the Hippo-YAP/TAZ signaling pathway in non-small cell lung cancer. Cell Death Discov. 7:1.
- Zhu Y, Bo H, Chen Z, Li J, He D, Xiao M, Xiang L, Jin L, Zhou J, Gong L, et al. 2020. LINC00968 can inhibit the progression of lung adenocarcinoma through the miR-21-5p/SMAD7 signal axis. Aging. 12(21):21904–21922.
- Zhuang C, Liu Y, Fu S, Yuan C, Luo J, Huang X, Yang W, Xie W, Zhuang C. 2020. Silencing of lncRNA MIR497HG via CRISPR/Cas13d induces bladder cancer progression through promoting the crosstalk between Hippo/Yap and TGF-beta/smad signaling. Front Mol Biosci. 7:616768. doi: 10.3389/fmolb.2020.616768.
- Zhuang C, Liu Y, Fu S, Yuan C, Luo J, Huang X, Yang W, Xie W, Zhuang C. 2021. Corrigendum: silencing of lncRNA MIR497HG via CRISPR/Cas13d induces bladder cancer progression through promoting the crosstalk between Hippo/Yap and TGF-beta/smad signaling. Front Mol Biosci. 8:664616. doi: 10.3389/fmolb.2021.664616.
- Zhuo H, Wu C, Tang J, Zhang F, Xu Z, Sun D, Teng Y, Tan Z. 2023. RP11-40C6.2 inactivates Hippo signaling by attenuating YAP1 ubiquitylation in hepatitis B virus-associated hepatocellular carcinoma. J Clin Transl Hepatol. 11(2):323–333.
- Zinatizadeh MR, Miri SR, Zarandi PK, Chalbatani GM, Raposo C, Mirzaei HR, Akbari ME, Mahmoodzadeh H. 2021. The Hippo tumor suppressor pathway (YAP/TAZ/TEAD/MST/LATS) and EGFR-RAS-RAF-MEK in cancer metastasis. Genes Dis. 8(1):48–60. doi: 10.1016/j.gendis.2019.11.003.