ABSTRACT
Breast cancer is a frequently occurring malignant tumor that is one of the leading causes of cancer-related deaths in women worldwide. Monoclonal antibodies that block programed cell death 1 (PD-1)/programed cell death ligand 1 (PD-L1) – a typical immune checkpoint – are currently the recommended standard therapies for many advanced and metastatic tumors such as triple-negative breast cancer. However, some patients develop drug resistance, leading to unfavorable treatment outcomes. Therefore, other approaches are required for anticancer treatments, such as downregulation of PD-L1 expression and promotion of degradation of PD-L1. Scoparone (SCO) is a bioactive compound isolated from Artemisia capillaris that exhibits antitumor activity. However, the effect of SCO on PD-L1 expression in cancer has not been confirmed yet. This study aimed to evaluate the role of SCO in PD-L1 expression in breast cancer cells in vitro. Our results show that SCO downregulated PD-L1 expression in a dose-dependent manner, via AKT inhibition. Interestingly, SCO treatment did not alter PTEN expression, but increased the expression of mitogen-activated protein kinase phosphatase-3 (MKP-3). In addition, the SCO-induced decrease in PD-L1 expression was reversed by siRNA-mediated MKP-3 knockdown. Collectively, these findings suggest that SCO inhibited the expression of PD-L1 in breast cancer cells by upregulating MKP-3 expression. Therefore, SCO may serve as an innovative combinatorial agent for cancer immunotherapy.
KEYWORDS:
Introduction
Breast cancer is the most frequently reported malignancy and leading cause of cancer-related deaths in women worldwide (Harbeck et al. Citation2019; Giaquinto et al. Citation2022). Immunotherapy has recently become a crucial treatment approach for cancer, in addition to surgery, chemotherapy, radiotherapy, and hormone therapy (Schneble et al. Citation2015; Waks and Winer Citation2019; Debien et al. Citation2023). In particular, immune checkpoint inhibitors – which block key immunosuppressive receptors, such as programed cell death 1 (PD-1), programed death ligand 1 (PD-L1), and cytotoxic T-lymphocyte-associated protein 4 (CTLA-4), – have revolutionized the treatment of solid tumors (Ribas and Wolchok Citation2018; Jacob et al. Citation2023).
PD-L1 is one of the most important molecular pathways used by tumor cells to evade the immune checkpoints of T cells (Ricklefs et al. Citation2018; Kumagai et al. Citation2020). PD-L1 expressed on the surface of tumor cells bind to PD-1 expressed by activated T cells, resulting in the direct inhibition of T cell effector functions against tumor cells (Iwai et al. Citation2017; Han et al. Citation2020). PD-L1 is frequently overexpressed in most breast cancer tissues, particularly in triple-negative breast cancer (TNBC) (Gatalica et al. Citation2014; Mittendorf et al. Citation2014; Twyman-Saint Victor et al. Citation2015). It is associated with several clinicopathological parameters that indicate poor outcomes and may increase the risk of mortality (Ghebeh et al. Citation2006; Wang C et al. Citation2017).
Scoparone (SCO) is a biologically active compound isolated from Artemisia capillaris that possesses various biological properties, including anti-allergic (Choi YH and Yan Citation2009), antitumor (Kim JK et al. Citation2013; Wu X et al. Citation2023), antioxidant (Atmaca et al. Citation2011) and anti-inflammatory activities (Cho et al. Citation2016; Lu et al. Citation2018). However, the role of SCO in regulating PD-L1 expression or in cancer immunotherapy has not yet been elucidated.
Mitogen-activated protein kinase phosphatase-3 (MKP-3) is a cytoplasmic dual-specificity phosphatase that preferentially dephosphorylates ERK (Muda et al. Citation1996), which is a major trigger for the development of most types of cancers (Garcia-Gomez et al. Citation2018; Maik-Rachline et al. Citation2019). MKP-3 exerts a tumor-suppressive effect via the negative regulation of ERK in different types of cancers, including pancreatic (Furukawa et al. Citation2003), lung (Okudela et al. Citation2009), ovarian (Chan et al. Citation2008), colon (Kim HS et al. Citation2021), breast cancer (Luo et al. Citation2015), and melanoma (Warmka et al. Citation2004).
Therefore, in this study, we investigated the effects of SCO on PD-L1 expression in breast cancer cells and found that SCO reduced the expression of PD-L1, which was mediated by AKT inhibition through the induction of MKP-3. Furthermore, MKP-3 knockdown resulted in increased AKT phosphorylation and PD-L1 expression. Collectively, these data provide evidence for SCO-induced MKP-3 expression as a mechanism that regulates PD-L1 expression in breast cancer cells. This suggests the potential application of SCO in cancer immunotherapy.
Materials and methods
Materials
SCO was obtained from MedChem Express (Monmouth Junction, NJ, USA). Anti-PD-L1, anti-phospho-NF-κB (Thr172), anti-phospho-AKT (Ser473), anti-phospho-ERK1/2, anti-phospho-STAT1 (Tyr701), anti-phospho-STAT3 (Tyr705), and anti-PTEN antibodies were obtained from Cell Signaling Technology (Danvers, MA, USA); anti-MKP-3 antibody was purchased from Abcam (Waltham, MA, USA). The anti-β-actin antibody was obtained from Santa Cruz Biotechnology (Dallas, TX, USA).
Cell culture
MCF7 and MDA-MB-231 cells were obtained from the Korean Cell Line Bank (Seoul, Republic of Korea) and cultured in RPMI 1640 medium (WELGENE, Gyeongsan, Republic of Korea) supplemented with 10% fetal bovine serum (Thermo Fisher Scientific, Waltham, MA, USA) and 1% antibiotics (WELGENE). The cells were incubated at 37°C in a humidified atmosphere containing 5% CO2.
Cell viability assay
The MDA-MB-231 cells were seeded at a density of 1 × 104 cells/well in 96-well plates. The cell counting kit-8 (CCK-8; Dojindo Molecular Technologies, Rockville, MD, USA) was used for cell viability assessment 24 h after incubation with SCO at various concentrations (0–500 μM), following the manufacturer’s instructions. The absorbance of the plates was measured at 450 nm using a Multiskan GO microplate spectrophotometer (Thermo Fisher Scientific) (Kim DB et al. Citation2022).
Western blot analysis
The cells were washed with ice-cold phosphate buffer saline and lysed on ice in a lysis buffer (50 mM Tris-HCl [pH 7.5], 150 mM NaCl, 1% Nonidet P-40, 0.1% sodium dodecyl sulfate, and 0.5% sodium deoxycholate) supplemented with protease and phosphatase inhibitors (Son et al. Citation2022). Aliquots containing equal amounts of protein were loaded and separated using sodium dodecyl sulfate–polyacrylamide gel electrophoresis. Proteins were then transferred onto nitrocellulose membranes (Bio-Rad, Hercules, CA, USA) and probed with the indicated antibodies. Protein bands were detected by chemiluminescence using a ChemiDoc gel imaging system (Bio-Rad).
RNA extraction and real-time quantitative polymerase chain reaction
Total RNA was isolated from cells using the AccuPrep Universal RNA Extraction Kit (Bioneer, Daejeon, Republic of Korea), according to the manufacturer’s instructions. Reverse transcription was performed using the AccuPower CycleScript RT PreMix (Bioneer) after RNA isolation and quantitation. Real-time quantitative polymerase chain reaction (RT-qPCR) experiments were performed using AccuPower 2X GreenStar qPCR Master Mix (Bioneer) and analyzed using a CFX Connect Real-Time PCR detection system (Bio-Rad). The normalization of gene expression levels was performed relative to the 18S ribosomal RNA (rRNA) as a reference housekeeping gene. The primer sequences used for RT-qPCR are listed in Supplementary Data 1.
Small-interfering RNA (siRNA) transfection
Pre-designed siRNAs targeting human MKP-3 (#1848-1) and control siRNA (#SN-1001) were purchased from Bioneer. MDA-MB-231 cells were seeded in 60-mm dishes, grown to approximately 80% confluence, and transfected with siRNA duplexes using Lipofectamine RNAiMAX (Thermo Fisher Scientific), according to the manufacturer’s recommendations. To determine the extent of siRNA inhibition, the expression of MKP-3 in transfected cells was assessed using western blotting.
Statistical analysis
Data were evaluated using analysis of variance (Sigma Stat 12.0, Systat Software, San Jose, CA, USA) with parametric or nonparametric post hoc analysis, and multiple comparisons were made using the least significant difference method. All data are presented as the mean ± standard deviation of at least three independent experiments. Statistical comparisons of the results were made using one-way analysis of variance, and p < 0.05 was considered statistically significant.
Results
SCO decreases the expression of PD-L1 in human breast cancer cells
The chemical structure of SCO is shown in (A). First, we performed a CCK-8 assay to examine the cytotoxic effect of SCO on MDA-MB-231 cells. As shown in (B), SCO treatment with up to 500 μM for 24 h exhibited no cytotoxic effects on MDA-MB-231 cells. Wu et al. recently reported that high concentration (up to 1000 μM) and prolonged (up to 72 h) SCO treatment decreased viability and induced apoptosis in breast cancer cells. However, they did not observe any cytotoxicity below 500 μM for 24 h (Wu X et al. Citation2023), which is consistent with our results. Therefore, we used a relatively safe dose of 100 μM in subsequent experiments to explore the molecular mechanism underlying the inhibition of PD-L1 expression by SCO.
Figure 1. Chemical structure and effect of scoparone (SCO) on cell viability. (A) Chemical structure of SCO. (B) Effect of treatment with vehicle (DMSO) or SCO concentrations for 24 h on the viability of MDA-MB-231 cells, as evaluated by the cell counting kit-8 assay. The results are represented as the mean ± standard deviation of three independent experiments. N.S. indicates not significant (p > 0.05).
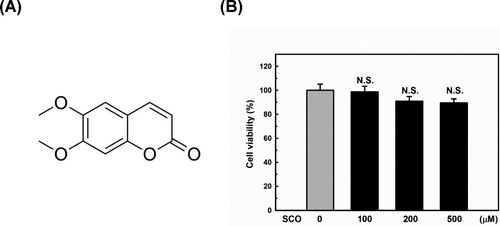
To examine the inhibitory effects of SCO, we used two breast cancer cell lines with differential PD-L1 expression under standard culture conditions. Consistent with the findings of previous studies (Rom-Jurek et al. Citation2018; Azadi et al. Citation2019), a high level of PD-L1 protein expression was observed in the MDA-MB-231 cells, whereas a low PD-L1 protein expression was observed in MCF-7 cells ((A)). The effects of SCO on PD-L1 protein and mRNA expression levels in human breast cancer cells were examined. As shown in (A and B), SCO treatment significantly downregulated PD-L1 expression by inhibiting transcription. SCO decreased PD-L1 expression in a dose-dependent manner ((C)).
Figure 2. Scoparone (SCO) downregulates the expression of programed cell death-ligand 1 (PD-L1) in human breast cancer cells. MDA-MB-231 (MB-231) cells were treated with vehicle (DMSO) or SCO (100 μM) for 24 h. MCF-7 was used as a negative control for PD-L1. (A) PD-L1 expression was measured by western blotting analysis. The β-actin protein level was considered as a loading control. Data are represented as mean ± standard deviation (n = 3). (B) The mRNA expression of PD-L1 was detected by real-time polymerase chain reaction. 18S ribosomal RNA was used as an internal control. Data are represented as mean ± standard deviation. (C) MDA-MB-231 (MB-231) cells were treated with various concentrations of SCO for 24 h, followed by a western blot analysis with the indicated antibodies.
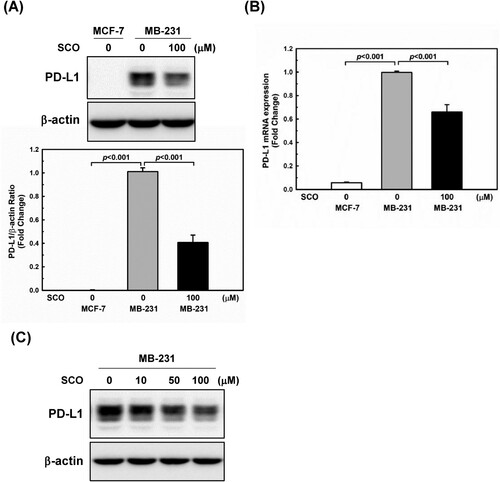
SCO inhibits activation of AKT in human breast cancer cells
The expression of PD-L1 in cancer cells is regulated by various oncogenic signaling pathways. MAPK (Wagner and Nebreda Citation2009; Guo et al. Citation2020) and PI3 K/AKT (Fresno Vara et al. Citation2004; Haddadi et al. Citation2018) are well-studied oncogenic pathways that play important roles in the pathogenesis of cancer by activating many downstream targets that regulate cell survival, proliferation, and motility. They are also vital for PD-L1 induction in cancer cells (Kim YB et al. Citation2019; Mansour et al. Citation2020; Zhang S et al. Citation2022). In addition, JAK-STAT pathway (Sasidharan Nair et al. Citation2018; Song et al. Citation2018) and NF-κB (Antonangeli et al. Citation2020) are related to the high levels of PD-L1 in several cancers. Thus, we examined the changes induced by SCO treatment in various signaling pathways. As shown in (A), among the signaling molecules that regulate PD-L1 expression, AKT phosphorylation decreased in a dose-dependent manner following SCO treatment. SCO treatment decreased AKT phosphorylation by 45% ((B)).
Figure 3. Scoparone (SCO) inhibits AKT phosphorylation in human breast cancer cells. (A) MDA-MB-231 (MB-231) cells were treated with various concentrations of SCO for 24 h, followed by using western blot analysis with the indicated antibodies. (B) Western blot was used to analyze the protein levels of p-AKT and AKT in MDA-MB-231 (MB-23) cells with or without SCO treatment for 24 h. The β-actin was used as a loading control. The data show the mean ± standard deviation for three independent experiments.
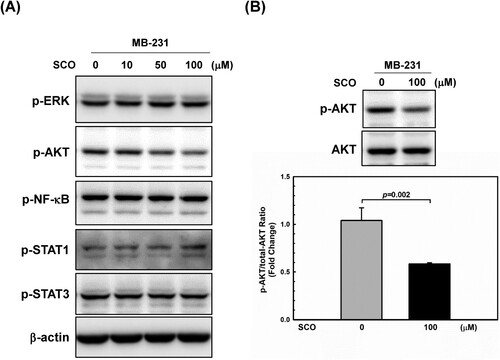
The gene encoding for phosphatase and tensin homolog deleted on chromosome ten (PTEN) is a major gatekeeper gene of the AKT signaling pathway. Additionally, it ranks among the most frequently deleted tumor suppressor genes in human cancer cells (Leslie and Downes Citation2004; Haddadi et al. Citation2018). MDA-MB-231 cells express wild-type PTEN (Hlobilkova et al. Citation2006; Wieland et al. Citation2021). In addition, Razmara et al. suggested that MKP-3 negatively modulates the PI3K-dependent AKT phosphorylation (Razmara et al. Citation2012). Therefore, we measured protein levels of PTEN and MKP-3 protein. PTEN expression remained unchanged, whereas MKP-3 expression increased with increasing SCO levels ((A)). SCO treatment resulted in a 46% increase in MKP-3 protein levels ((B)) and a 52% increase in MKP-3 mRNA levels ((C)). To examine whether MKP-3 affected AKT phosphorylation in MDA-MB-231 cells, we conducted MKP-3 knockdown experiments. As shown in (D), a 2.1-fold increase in phosphorylated AKT was observed in cells with MKP-3 knockdown. This result is consistent with the findings of Razmara et al (Razmara et al. Citation2012).
Figure 4. Scoparone (SCO)-induced expression of mitogen-activated protein kinase phosphatase-3 (MKP-3) inhibits AKT phosphorylation in human breast cancer cells. (A) MDA-MB-231 (MB-231) cells were treated with various concentrations of SCO for 24 h, followed by using western blot analysis with the indicated antibodies. (B) MDA-MB-231 (MB-231) cells were treated with vehicle (DMSO) or SCO (100 μM) for 24 h. MKP-3 expression was measured using western blotting analysis. The β-actin protein level was considered as a loading control. (C) The mRNA expression of MKP-3 was detected by real-time polymerase chain reaction. 18S ribosomal RNA was used as an internal control. (D) MDA-MB-231 (MB-231) cells were transfected with either non-targeting siRNA (siC) or siRNA directed against MKP-3 (siMKP-3) for 48 h. MKP-3, p-AKT, and β-actin levels were assessed using western blot analysis. All Data are represented as the mean ± standard deviation (n = 3).
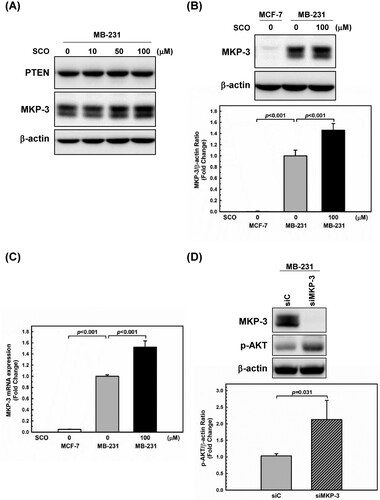
SCO-induced reduction of PD-L1 expression in human breast cancer cells is mediated by MKP-3
To further investigate whether MKP-3 is necessary for the SCO-mediated inhibition of PD-L1 expression, MDA-MB-231 cells were transfected with either control siRNA or MKP-3 siRNA and then treated with SCO. Even in untreated controls, MKP-3 knockdown led to a marked increase in the expression of PD-L1. In addition, PD-L1 inhibition by SCO was abolished when MKP-3 was knocked down ((A)). These results suggested that MKP-3 is involved in the reduction of SCO-induced PD-L1 expression in human breast cancer cells.
Figure 5. Mitogen-activated protein kinase phosphatase-3 (MKP-3) is necessary for scoparone (SCO)-mediated inhibition of programed cell death-ligand 1 (PD-L1) expression. (A) MDA-MB-231 (MB-231) cells were transfected with either non-targeting siRNA (siC) or siRNA directed against MKP-3 (siMKP-3) for 24 h and subsequently exposed to vehicle (DMSO) or SCO (100 μM) for 24 h. MKP-3, PD-L1, and β-actin levels were assessed using western blot analysis. (B). Possible mechanisms underlying the reduction in the activity of PD-L1 mediated by SCO. SCO-induced activation of MKP-3 reduced PD-L1 expression via inhibition of AKT.
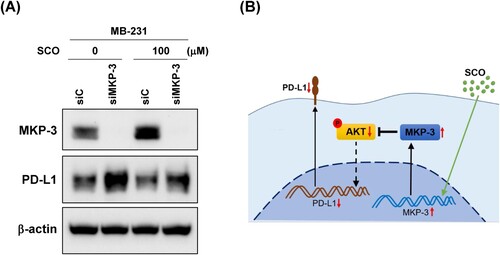
Discussion
PD-L1 is an immunoglobulin-like molecule that is widely expressed in most human cancers. The binding of PD-L1 to its receptor, PD-1, on T cells results in immunosuppression, leading to immune evasion in cancer (Chen Citation2004; Tsushima et al. Citation2007). Overexpression of PD-L1 is associated with poor prognosis (Ghebeh et al. Citation2006; Muenst et al. Citation2014; Wang C et al. Citation2017; Wu Z et al. Citation2019), larger tumors, higher tumor grade, estrogen receptor-negative, progesterone receptor-negative, HER-2-positive status, cell proliferation, and an increased abundance of tumor-infiltrating lymphocytes (TILs) in patients with breast cancer (Bertucci et al. Citation2015; Twyman-Saint Victor et al. Citation2015). Tumors overexpressing PD-L1 are often accompanied by the infiltration of PD-1-positive TILs, which are associated with shortened overall survival, indicating a poor prognosis for breast cancer (Muenst et al. Citation2013; Sun et al. Citation2014). PD-L1 affects not only T cells but also tumor-associated macrophages, inducing tumor-promoting M2 polarization (Meng et al. Citation2022; Zhang R et al. Citation2022).
Although inhibition of the PD-L1/PD-1 axis has been developed as an important approach to cancer immunotherapy, PD-L1 also exhibits tumor-intrinsic functions. For instance, PD-L1 signaling promotes epithelial-to-mesenchymal transition in several cancer subtypes (Alsuliman et al. Citation2015; Wang Y et al. Citation2015). PD-L1 also acts as an anti-apoptotic receptor in response to Fas ligation. Furthermore, it is associated with cancer stem cell proliferation (Azuma et al. Citation2008; Yang et al. Citation2015). PD-L1 can enhance the proliferation and survival of acute myeloid leukemia cells by upregulating the pentose phosphate pathway, which is crucial for cell proliferation and the β-oxidation of fatty acids, which promotes cell survival (Soltani et al. Citation2023). PD-L1 suppresses tumor autophagy by promoting basal mTORC1 signaling in melanoma and ovarian cancer models (Clark et al. Citation2016). Therefore, in addition to targeting the disruption of PD-L1/PD-1, it is important to identify agents that impede the expression of PD-L1.
SCO, also known as 6,7-dimethoxycoumarin, has been proposed to exert antitumor activity by regulating signaling pathways involving AKT (Li N et al. Citation2021; Huang S et al. Citation2023), NF-kB (Wu X et al. Citation2023), and STAT3 (Kim JK et al. Citation2013). Since PD-L1 expression in cancer cells is also regulated by signaling pathways, such as AKT (Fresno Vara et al. Citation2004; Haddadi et al. Citation2018), NF-κB (Antonangeli et al. Citation2020), and STAT3 (Marzec et al. Citation2008), we investigated whether SCO can regulate PD-L1 expression.
In this study, we identified SCO as a PD-L1 inhibitor in MDA-MB-231 cells – a highly aggressive, invasive, and poorly differentiated TNBC cell line (). SCO decreases AKT phosphorylation, a crucial signaling pathway for PD-L1 expression (Fresno Vara et al. Citation2004), but does not upregulate PTEN. Instead, this decrease was attributed to an increase in MKP-3 levels ( and ). Although Razmara et al. suggested that MKP-3 inhibits AKT activity (Razmara et al. Citation2012), Rodrigues et al. reported that MKP-3 overexpression does not affect AKT phosphorylation (Rodrigues et al. Citation2017). Therefore, MKP-3-mediated AKT dephosphorylation might be an indirect effect. Interestingly, SCO treatment increased MKP-3 protein levels by 46% ((B)) but did not affect the phosphorylation of ERK ((A)), which is the preferred substrate of MKP-3. Regulation of protein phosphorylation requires a balance between the activities of protein kinases and phosphatases. Although MKP-3 is an important phosphatase for ERK, several other phosphatases – such as PP1 (Li R et al. Citation2013), PP2A (Yu et al. Citation2004), DUSP5 (Rushworth et al. Citation2014), DUSP7 (Keyse Citation2008), MKP-1 (Zhao et al. Citation2021), MKP-4 (Emanuelli et al. Citation2008), MKP-5 (Nomura et al. Citation2012), and alkaline phosphatase (ALP) (Sharma et al. Citation2014) – have also been reported to dephosphorylate ERK. Other kinases such as PI3 K, conventional protein kinase C (Grammer and Blenis Citation1997), and TGF-β-activated kinase 1 (TAK1) (Simard et al. Citation2015) play major roles in MEK-independent ERK activation. Therefore, further research is necessary on the upstream kinases and phosphatases that may regulate ERK phosphorylation.
The knockdown experiments further confirmed that the SCO-induced increase in MKP-3 expression was required for the suppression of PD-L1 expression ((A)). MKP-3 knockdown alone led to a substantial increase in PD-L1 protein levels ((A)). This implied that MKP-3 plays an important role in regulating the expression of PD-L1. To our knowledge, this is the first report describing the role of MKP-3 in reducing PD-L1 expression in cancer cells via AKT regulation, which presents a new therapeutic target for PD-L1 regulation.
Kim et al. reported that SCO inhibited the phosphorylation and transcriptional activity of STAT3 in DU145 prostate cancer cells (Kim JK et al. Citation2013); however, no inhibition of STAT3 was observed in the present study. Several drugs exhibited varying effects on STAT3 in MDA-MB-231 and DU145 cells: SH003 inhibited STAT3 in MDA-MB-231 cells, but not in DU145 cells (Choi YJ et al. Citation2016); the methylene chloride fraction of Chrysanthemum indicum L. exhibited a clear inhibitory effect on constitutive STAT3 activation in DU145 cells, but not in MDA-MB-231 cells (Kim C et al. Citation2013). Thus, SCO may have different signaling effects on different types of cancer cells.
Collectively, the findings of the present study suggest that SCO attenuates PD-L1 expression in human breast cancer cells via MKP-3 upregulation ((B)).
Recently, curcumin was reported to promote PD-L1 degradation and sensitize breast cancer cells to anti-cytotoxic T-lymphocyte-associated antigen 4 therapy (Lim et al. Citation2016). Furthermore, extracellular release of PD-L1 with platycodin D increased interleukin-2 production in activated Jurkat T cells (Huang MY et al. Citation2019), suggesting that phytochemicals, including SCO, can selectively reduce PD-L1 expression on the cell membrane, leading to an immunotherapeutic effect. As mentioned previously, PD-L1 is involved in various intrinsic tumor functions. Therefore, further studies are required to investigate the effects of SCO on epithelial-to-mesenchymal transition, proliferation, survival, and autophagy.
Supplemental Material
Download MS Word (13.8 KB)Acknowledgments
This study was supported by grants from the Korea Health Technology R&D Project through the Korea Health Industry Development Institute (KHIDI), funded by the Ministry of Health & Welfare, Republic of Korea (grant number: HI22C1989), and by the Inha University Research Grant (INHA-67953). The authors thank Ok Hee Park, a research technician at the Department of Molecular Medicine, Inha University College of Medicine, for her technical assistance. The authors thank Siyoon Kim, Cheongna Dalton School, Incheon 22742, Republic of Korea, for her assistance with the graphics shown in (B).
Disclosure statement
No potential conflict of interest was reported by the author(s).
Additional information
Funding
References
- Alsuliman A, Colak D, Al-Harazi O, Fitwi H, Tulbah A, Al-Tweigeri T, Al-Alwan M, Ghebeh H. 2015. Bidirectional crosstalk between PD-L1 expression and epithelial to mesenchymal transition: significance in claudin-low breast cancer cells. Mol Cancer. 14:149. doi:10.1186/s12943-015-0421-2.
- Antonangeli F, Natalini A, Garassino MC, Sica A, Santoni A, Di Rosa F. 2020. Regulation of PD-L1 Expression by NF-kappaB in Cancer. Front Immunol. 11:584626. doi:10.3389/fimmu.2020.584626.
- Atmaca M, Bilgin HM, Obay BD, Diken H, Kelle M, Kale E. 2011. The hepatoprotective effect of coumarin and coumarin derivates on carbon tetrachloride-induced hepatic injury by antioxidative activities in rats. J Physiol Biochem. 67(4):569–576. doi:10.1007/s13105-011-0103-5.
- Azadi S, Aboulkheyr Es H, Razavi Bazaz S, Thiery JP, Asadnia M, Ebrahimi Warkiani M. 2019. Upregulation of PD-L1 expression in breast cancer cells through the formation of 3D multicellular cancer aggregates under different chemical and mechanical conditions. Biochim Biophys Acta Mol Cell Res. 1866(12):118526. doi:10.1016/j.bbamcr.2019.118526.
- Azuma T, Yao S, Zhu G, Flies AS, Flies SJ, Chen L. 2008. B7-H1 is a ubiquitous antiapoptotic receptor on cancer cells. Blood. 111(7):3635–3643. doi:10.1182/blood-2007-11-123141.
- Bertucci F, Finetti P, Colpaert C, Mamessier E, Parizel M, Dirix L, Viens P, Birnbaum D, van Laere S. 2015. PDL1 expression in inflammatory breast cancer is frequent and predicts for the pathological response to chemotherapy. Oncotarget. 6(15):13506–13519. doi:10.18632/oncotarget.3642.
- Chan DW, Liu VW, Tsao GS, Yao KM, Furukawa T, Chan KK, Ngan HY. 2008. Loss of MKP3 mediated by oxidative stress enhances tumorigenicity and chemoresistance of ovarian cancer cells. Carcinogenesis. 29(9):1742–1750. doi:10.1093/carcin/bgn167.
- Chen L. 2004. Co-inhibitory molecules of the B7-CD28 family in the control of T-cell immunity. Nat Rev Immunol. 4(5):336–347. doi:10.1038/nri1349.
- Cho DY, Ko HM, Kim J, Kim BW, Yun YS, Park JI, Ganesan P, Lee JT, Choi DK. 2016. Scoparone Inhibits LPS-Simulated Inflammatory Response by Suppressing IRF3 and ERK in BV-2 Microglial Cells. Molecules. 21(12):1718. doi:10.3390/molecules21121718.
- Choi YH, Yan GH. 2009. Anti-allergic effects of scoparone on mast cell-mediated allergy model. Phytomedicine. 16(12):1089–1094. doi:10.1016/j.phymed.2009.05.003.
- Choi YJ, Choi YK, Lee KM, Cho SG, Kang SY, Ko SG. 2016. SH003 induces apoptosis of DU145 prostate cancer cells by inhibiting ERK-involved pathway. BMC Complement Altern Med. 16(1):507. doi:10.1186/s12906-016-1490-5.
- Clark CA, Gupta HB, Sareddy G, Pandeswara S, Lao S, Yuan B, Drerup JM, Padron A, Conejo-Garcia J, Murthy K, et al. 2016. Tumor-intrinsic PD-L1 signals regulate cell growth, pathogenesis, and autophagy in ovarian cancer and melanoma. Cancer Res. 76(23):6964–6974. doi:10.1158/0008-5472.CAN-16-0258.
- Debien V, De Caluwe A, Wang X, Piccart-Gebhart M, Tuohy VK, Romano E, Buisseret L. 2023. Immunotherapy in breast cancer: an overview of current strategies and perspectives. NPJ Breast Cancer. 9(1):7. doi:10.1038/s41523-023-00508-3.
- Emanuelli B, Eberle D, Suzuki R, Kahn CR. 2008. Overexpression of the dual-specificity phosphatase MKP-4/DUSP-9 protects against stress-induced insulin resistance. Proc Natl Acad Sci USA. 105(9):3545–3550. doi:10.1073/pnas.0712275105.
- Fresno Vara JA, Casado E, de Castro J, Cejas P, Belda-Iniesta C, Gonzalez-Baron M. 2004. PI3 K/Akt signalling pathway and cancer. Cancer Treat Rev. 30(2):193–204. doi:10.1016/j.ctrv.2003.07.007.
- Furukawa T, Sunamura M, Motoi F, Matsuno S, Horii A. 2003. Potential tumor suppressive pathway involving DUSP6/MKP-3 in pancreatic cancer. Am J Pathol. 162(6):1807–1815. doi:10.1016/S0002-9440(10)64315-5.
- Garcia-Gomez R, Bustelo XR, Crespo P. 2018. Protein-protein interactions: emerging oncotargets in the RAS-ERK pathway. Trends Cancer. 4(9):616–633. doi:10.1016/j.trecan.2018.07.002.
- Gatalica Z, Snyder C, Maney T, Ghazalpour A, Holterman DA, Xiao N, Overberg P, Rose I, Basu GD, Vranic S, et al. 2014. Programmed cell death 1 (PD-1) and its ligand (PD-L1) in common cancers and their correlation with molecular cancer type. Cancer Epidemiol Biomarkers Prev. 23(12):2965–2970. doi:10.1158/1055-9965.EPI-14-0654.
- Ghebeh H, Mohammed S, Al-Omair A, Qattan A, Lehe C, Al-Qudaihi G, Elkum N, Alshabanah M, Bin Amer S, Tulbah A, et al. 2006. The B7-H1 (PD-L1) T lymphocyte-inhibitory molecule is expressed in breast cancer patients with infiltrating ductal carcinoma: correlation with important high-risk prognostic factors. Neoplasia. 8(3):190–198. doi:10.1593/neo.05733.
- Giaquinto AN, Sung H, Miller KD, Kramer JL, Newman LA, Minihan A, Jemal A, Siegel RL. 2022. Breast cancer statistics, 2022. CA Cancer J Clin. 72(6):524–541. doi:10.3322/caac.21754.
- Grammer TC, Blenis J. 1997. Evidence for MEK-independent pathways regulating the prolonged activation of the ERK-MAP kinases. Oncogene. 14(14):1635–1642. doi:10.1038/sj.onc.1201000.
- Guo YJ, Pan WW, Liu SB, Shen ZF, Xu Y, Hu LL. 2020. ERK/MAPK signalling pathway and tumorigenesis. Exp Ther Med. 19(3):1997–2007.
- Haddadi N, Lin Y, Travis G, Simpson AM, Nassif NT, McGowan EM. 2018. PTEN/PTENP1: ‘Regulating the regulator of RTK-dependent PI3 K/Akt signalling’, new targets for cancer therapy. Mol Cancer. 17(1):37. doi:10.1186/s12943-018-0803-3.
- Han Y, Liu D, Li L. 2020. PD-1/PD-L1 pathway: current researches in cancer. Am J Cancer Res. 10(3):727–742.
- Harbeck N, Penault-Llorca F, Cortes J, Gnant M, Houssami N, Poortmans P, Ruddy K, Tsang J, Cardoso F. 2019. Breast cancer. Nat Rev Dis Primers. 5(1):66. doi:10.1038/s41572-019-0111-2.
- Hlobilkova A, Knillova J, Svachova M, Skypalova P, Krystof V, Kolar Z. 2006. Tumour suppressor PTEN regulates cell cycle and protein kinase B/Akt pathway in breast cancer cells. Anticancer Res. 26(2A):1015–1022.
- Huang MY, Jiang XM, Xu YL, Yuan LW, Chen YC, Cui G, Huang RY, Liu B, Wang Y, Chen X, et al. 2019. Platycodin D triggers the extracellular release of programed death Ligand-1 in lung cancer cells. Food Chem Toxicol. 131:110537. doi:10.1016/j.fct.2019.05.045.
- Huang S, Lin L, Ma Y, Zhu Q, Weng N. 2023. Scoparone induces autophagic cell death via the PAK1/AKT axis in colorectal cancer. Eur J Pharmacol. 959:176091. doi:10.1016/j.ejphar.2023.176091.
- Iwai Y, Hamanishi J, Chamoto K, Honjo T. 2017. Cancer immunotherapies targeting the PD-1 signaling pathway. J Biomed Sci. 24(1):26. doi:10.1186/s12929-017-0329-9.
- Jacob SL, Huppert LA, Rugo HS. 2023. Role of immunotherapy in breast cancer. JCO Oncol Pract. 19(4):167–179. doi:10.1200/OP.22.00483.
- Keyse SM. 2008. Dual-specificity MAP kinase phosphatases (MKPs) and cancer. Cancer Metastasis Rev. 27(2):253–261. doi:10.1007/s10555-008-9123-1.
- Kim C, Kim MC, Kim SM, Nam D, Choi SH, Kim SH, Ahn KS, Lee EH, Jung SH, Ahn KS. 2013. Chrysanthemum indicum L. extract induces apoptosis through suppression of constitutive STAT3 activation in human prostate cancer DU145 cells. Phytother Res. 27(1):30–38. doi:10.1002/ptr.4689.
- Kim DB, Unenkhuu B, Kim GJ, Kim SW, Kim HS. 2022. Cynarin attenuates LPS-induced endothelial inflammation via upregulation of the negative regulator MKP-3. Anim Cells Syst (Seoul). 26(3):119–128. doi:10.1080/19768354.2022.2077438.
- Kim HS, Kang YH, Lee J, Han SR, Kim DB, Ko H, Park S, Lee MS. 2021. Biphasic regulation of mitogen-activated protein kinase phosphatase 3 in hypoxic colon cancer cells. Mol Cells. 44(10):710–722. doi:10.14348/molcells.2021.0093.
- Kim JK, Kim JY, Kim HJ, Park KG, Harris RA, Cho WJ, Lee JT, Lee IK. 2013. Scoparone exerts anti-tumor activity against DU145 prostate cancer cells via inhibition of STAT3 activity. PLoS One. 8(11):e80391. doi:10.1371/journal.pone.0080391.
- Kim YB, Ahn JM, Bae WJ, Sung CO, Lee D. 2019. Functional loss of ARID1A is tightly associated with high PD-L1 expression in gastric cancer. Int J Cancer. 145(4):916–926. doi:10.1002/ijc.32140.
- Kumagai S, Togashi Y, Kamada T, Sugiyama E, Nishinakamura H, Takeuchi Y, Vitaly K, Itahashi K, Maeda Y, Matsui S, et al. 2020. The PD-1 expression balance between effector and regulatory T cells predicts the clinical efficacy of PD-1 blockade therapies. Nat Immunol. 21(11):1346–1358. doi:10.1038/s41590-020-0769-3.
- Leslie NR, Downes CP. 2004. PTEN function: how normal cells control it and tumour cells lose it. Biochem J. 382(Pt 1):1–11. doi:10.1042/BJ20040825.
- Li N, Yang F, Liu DY, Guo JT, Ge N, Sun SY. 2021. Scoparone inhibits pancreatic cancer through PI3 K/Akt signaling pathway. World J Gastrointest Oncol. 13(9):1164–1183. doi:10.4251/wjgo.v13.i9.1164.
- Li R, Gong Z, Pan C, Xie DD, Tang JY, Cui M, Xu YF, Yao W, Pang Q, Xu ZG, et al. 2013. Metal-dependent protein phosphatase 1A functions as an extracellular signal-regulated kinase phosphatase. FEBS J. 280(11):2700–2711. doi:10.1111/febs.12275.
- Lim SO, Li CW, Xia W, Cha JH, Chan LC, Wu Y, Chang SS, Lin WC, Hsu JM, Hsu YH, et al. 2016. Deubiquitination and stabilization of PD-L1 by CSN5. Cancer Cell. 30(6):925–939. doi:10.1016/j.ccell.2016.10.010.
- Lu C, Li Y, Hu S, Cai Y, Yang Z, Peng K. 2018. Scoparone prevents IL-1beta-induced inflammatory response in human osteoarthritis chondrocytes through the PI3 K/Akt/NF-kappaB pathway. Biomed Pharmacother. 106:1169–1174. doi:10.1016/j.biopha.2018.07.062.
- Luo ML, Gong C, Chen CH, Hu H, Huang P, Zheng M, Yao Y, Wei S, Wulf G, Lieberman J, et al. 2015. The Rab2A GTPase promotes breast cancer stem cells and tumorigenesis via Erk signaling activation. Cell Rep. 11(1):111–124. doi:10.1016/j.celrep.2015.03.002.
- Maik-Rachline G, Hacohen-Lev-Ran A, Seger R. 2019. Nuclear ERK: mechanism of translocation, substrates, and role in cancer. Int J Mol Sci. 20(5):1194. doi:10.3390/ijms20051194.
- Mansour FA, Al-Mazrou A, Al-Mohanna F, Al-Alwan M, Ghebeh H. 2020. PD-L1 is overexpressed on breast cancer stem cells through notch3/mTOR axis. Oncoimmunology. 9(1):1729299. doi:10.1080/2162402X.2020.1729299.
- Marzec M, Zhang Q, Goradia A, Raghunath PN, Liu X, Paessler M, Wang HY, Wysocka M, Cheng M, Ruggeri BA, et al. 2008. Oncogenic kinase NPM/ALK induces through STAT3 expression of immunosuppressive protein CD274 (PD-L1, B7-H1). Proc Natl Acad Sci USA. 105(52):20852–20857. doi:10.1073/pnas.0810958105.
- Meng Z, Zhang R, Wu X, Zhang M, Jin T. 2022. PD-L1 mediates triple-negative breast cancer evolution via the regulation of TAM/M2 polarization. Int J Oncol. 61(6). doi:10.3892/ijo.2022.5440.
- Mittendorf EA, Philips AV, Meric-Bernstam F, Qiao N, Wu Y, Harrington S, Su X, Wang Y, Gonzalez-Angulo AM, Akcakanat A, et al. 2014. PD-L1 expression in triple-negative breast cancer. Cancer Immunol Res. 2(4):361–370. doi:10.1158/2326-6066.CIR-13-0127.
- Muda M, Boschert U, Dickinson R, Martinou JC, Martinou I, Camps M, Schlegel W, Arkinstall S. 1996. MKP-3, a novel cytosolic protein-tyrosine phosphatase that exemplifies a new class of mitogen-activated protein kinase phosphatase. J Biol Chem. 271(8):4319–4326. doi:10.1074/jbc.271.8.4319.
- Muenst S, Schaerli AR, Gao F, Daster S, Trella E, Droeser RA, Muraro MG, Zajac P, Zanetti R, Gillanders WE, et al. 2014. Expression of programmed death ligand 1 (PD-L1) is associated with poor prognosis in human breast cancer. Breast Cancer Res Treat. 146(1):15–24. doi:10.1007/s10549-014-2988-5.
- Muenst S, Soysal SD, Gao F, Obermann EC, Oertli D, Gillanders WE. 2013. The presence of programmed death 1 (PD-1)-positive tumor-infiltrating lymphocytes is associated with poor prognosis in human breast cancer. Breast Cancer Res Treat. 139(3):667–676. doi:10.1007/s10549-013-2581-3.
- Nomura M, Shiiba K, Katagiri C, Kasugai I, Masuda K, Sato I, Sato M, Kakugawa Y, Nomura E, Hayashi K, et al. 2012. Novel function of MKP-5/DUSP10, a phosphatase of stress-activated kinases, on ERK-dependent gene expression, and upregulation of its gene expression in colon carcinomas. Oncol Rep. 28(3):931–936.
- Okudela K, Yazawa T, Woo T, Sakaeda M, Ishii J, Mitsui H, Shimoyamada H, Sato H, Tajiri M, Ogawa N, et al. 2009. Down-regulation of DUSP6 expression in lung cancer: its mechanism and potential role in carcinogenesis. Am J Pathol. 175(2):867–881. doi:10.2353/ajpath.2009.080489.
- Razmara M, Eger G, Rorsman C, Heldin CH, Lennartsson J. 2012. MKP3 negatively modulates PDGF-induced Akt and Erk5 phosphorylation as well as chemotaxis. Cell Signal. 24(3):635–640. doi:10.1016/j.cellsig.2011.11.001.
- Ribas A, Wolchok JD. 2018. Cancer immunotherapy using checkpoint blockade. Science. 359(6382):1350–1355. doi:10.1126/science.aar4060.
- Ricklefs FL, Alayo Q, Krenzlin H, Mahmoud AB, Speranza MC, Nakashima H, Hayes JL, Lee K, Balaj L, Passaro C, et al. 2018. Immune evasion mediated by PD-L1 on glioblastoma-derived extracellular vesicles. Sci Adv. 4(3):eaar2766. doi:10.1126/sciadv.aar2766.
- Rodrigues BA, Kuga GK, Munoz VR, Gaspar RC, Tavares MR, Botezelli JD, da Silva ASR, Cintra DE, de Moura LP, Simabuco FM, et al. 2017. Overexpression of Mitogen-activated protein kinase phosphatase-3 (MKP-3) reduces FoxO1 phosphorylation in mice hypothalamus. Neurosci Lett. 659:14–17. doi:10.1016/j.neulet.2017.08.067.
- Rom-Jurek EM, Kirchhammer N, Ugocsai P, Ortmann O, Wege AK, Brockhoff G. 2018. Regulation of programmed death Ligand 1 (PD-L1) expression in breast cancer cell lines in vitro and in immunodeficient and humanized tumor mice. Int J Mol Sci. 19(2).
- Rushworth LK, Kidger AM, Delavaine L, Stewart G, van Schelven S, Davidson J, Bryant CJ, Caddye E, East P, Caunt CJ, et al. 2014. Dual-specificity phosphatase 5 regulates nuclear ERK activity and suppresses skin cancer by inhibiting mutant Harvey-Ras (HRasQ61L)-driven SerpinB2 expression. Proc Natl Acad Sci USA. 111(51):18267–18272. doi:10.1073/pnas.1420159112.
- Sasidharan Nair V, Toor SM, Ali BR, Elkord E. 2018. Dual inhibition of STAT1 and STAT3 activation downregulates expression of PD-L1 in human breast cancer cells. Expert Opin Ther Targets. 22(6):547–557. doi:10.1080/14728222.2018.1471137.
- Schneble E, Jinga DC, Peoples G. 2015. Breast cancer immunotherapy. Maedica (Bucur). 10(2):185–191.
- Sharma U, Pal D, Prasad R. 2014. A novel role of alkaline phosphatase in the ERK1/2 dephosphorylation in renal cell carcinoma cell lines: a new plausible therapeutic target. Biochimie. 107(Pt B):406–409. doi:10.1016/j.biochi.2014.09.016.
- Simard FA, Cloutier A, Ear T, Vardhan H, McDonald PP. 2015. MEK-independent ERK activation in human neutrophils and its impact on functional responses. J Leukoc Biol. 98(4):565–573. doi:10.1189/jlb.2MA1214-599R.
- Soltani M, Ghanadian M, Ghezelbash B, Shokouhi A, Zamyatnin AA Jr, Bazhin AV, Ganjalikhani-Hakemi M. 2023. PD-L1 stimulation can promote proliferation and survival of leukemic cells by influencing glucose and fatty acid metabolism in acute myeloid leukemia. BMC Cancer. 23(1):447. doi:10.1186/s12885-023-10947-7.
- Son S, Yoo SA, Nam K, Oh S, Lee KM, Yi JY, Shin I. 2022. Brain type of creatine kinase induces doxorubicin resistance via TGF-beta signaling in MDA-MB-231 breast cancer cells. Anim Cells Syst (Seoul). 26(5):203–213. doi:10.1080/19768354.2022.2107070.
- Song TL, Nairismagi ML, Laurensia Y, Lim JQ, Tan J, Li ZM, Pang WL, Kizhakeyil A, Wijaya GC, Huang DC, et al. 2018. Oncogenic activation of the STAT3 pathway drives PD-L1 expression in natural killer/T-cell lymphoma. Blood. 132(11):1146–1158. doi:10.1182/blood-2018-01-829424.
- Sun S, Fei X, Mao Y, Wang X, Garfield DH, Huang O, Wang J, Yuan F, Sun L, Yu Q, et al. 2014. PD-1(+) immune cell infiltration inversely correlates with survival of operable breast cancer patients. Cancer Immunol Immunother. 63(4):395–406. doi:10.1007/s00262-014-1519-x.
- Tsushima F, Yao S, Shin T, Flies A, Flies S, Xu H, Tamada K, Pardoll DM, Chen L. 2007. Interaction between B7-H1 and PD-1 determines initiation and reversal of T-cell anergy. Blood. 110(1):180–185. doi:10.1182/blood-2006-11-060087.
- Twyman-Saint Victor C, Rech AJ, Maity A, Rengan R, Pauken KE, Stelekati E, Benci JL, Xu B, Dada H, Odorizzi PM, et al. 2015. Radiation and dual checkpoint blockade activate non-redundant immune mechanisms in cancer. Nature. 520(7547):373–377. doi:10.1038/nature14292.
- Wagner EF, Nebreda AR. 2009. Signal integration by JNK and p38 MAPK pathways in cancer development. Nat Rev Cancer. 9(8):537–549. doi:10.1038/nrc2694.
- Waks AG, Winer EP. 2019. Breast cancer treatment: a review. JAMA. 321(3):288–300. doi:10.1001/jama.2018.19323.
- Wang C, Zhu H, Zhou Y, Mao F, Lin Y, Pan B, Zhang X, Xu Q, Huang X, Sun Q. 2017. Prognostic value of PD-L1 in breast cancer: a meta-analysis. Breast J. 23(4):436–443. doi:10.1111/tbj.12753.
- Wang Y, Wang H, Zhao Q, Xia Y, Hu X, Guo J. 2015. PD-L1 induces epithelial-to-mesenchymal transition via activating SREBP-1c in renal cell carcinoma. Med Oncol. 32(8):212. doi:10.1007/s12032-015-0655-2.
- Warmka JK, Mauro LJ, Wattenberg EV. 2004. Mitogen-activated protein kinase phosphatase-3 is a tumor promoter target in initiated cells that express oncogenic Ras. J Biol Chem. 279(32):33085–33092. doi:10.1074/jbc.M403120200.
- Wieland A, Strissel PL, Schorle H, Bakirci E, Janzen D, Beckmann MW, Eckstein M, Dalton PD, Strick R. 2021. Brain and breast cancer cells with PTEN loss of function reveal enhanced durotaxis and RHOB dependent amoeboid migration utilizing 3D scaffolds and aligned microfiber tracts. Cancers (Basel). 13(20):5144. doi:10.3390/cancers13205144.
- Wu X, Li X, Li J, Zhao X, Cui Y, Eerdun C. 2023. Scoparone inhibits breast cancer cell viability through the NF-kappaB signaling pathway. Exp Ther Med. 26(1):328. doi:10.3892/etm.2023.12027.
- Wu Z, Zhang L, Peng J, Xu S, Zhou L, Lin Y, Wang Y, Lu J, Yin W, Lu J. 2019. Predictive and prognostic value of PDL1 protein expression in breast cancer patients in neoadjuvant setting. Cancer Biol Ther. 20(6):941–947. doi:10.1080/15384047.2019.1583533.
- Yang Y, Wu KE, Zhao E, Li W, Shi L, Xie G, Jiang B, Wang Y, Li R, Zhang P, et al. 2015. B7-H1 enhances proliferation ability of gastric cancer stem-like cells as a receptor. Oncol Lett. 9(4):1833–1838. doi:10.3892/ol.2015.2949.
- Yu LG, Packman LC, Weldon M, Hamlett J, Rhodes JM. 2004. Protein phosphatase 2A, a negative regulator of the ERK signaling pathway, is activated by tyrosine phosphorylation of putative HLA class II-associated protein I (PHAPI)/pp32 in response to the antiproliferative lectin, jacalin. J Biol Chem. 279(40):41377–41383. doi:10.1074/jbc.M400017200.
- Zhang R, Meng Z, Wu X, Zhang M, Piao Z, Jin T. 2022. PD-L1/p-STAT3 promotes the progression of NSCLC cells by regulating TAM polarization. J Cell Mol Med. 26(23):5872–5886. doi:10.1111/jcmm.17610.
- Zhang S, You X, Xu T, Chen Q, Li H, Dou L, Sun Y, Xiong X, Meredith MA, Sun Y. 2022. PD-L1 induction via the MEK-JNK-AP1 axis by a neddylation inhibitor promotes cancer-associated immunosuppression. Cell Death Dis. 13(10):844. doi:10.1038/s41419-022-05292-9.
- Zhao L, Su J, Liu S, Li Y, Xi T, Ruan J, Liang KX, Huang R. 2021. MAP kinase phosphatase MKP-1 regulates p-ERK1/2 signaling pathway with fluoride treatment. Biochem Biophys Res Commun. 542:65–72. doi:10.1016/j.bbrc.2020.12.100.