ABSTRACT
Background
Male infertility is a worldwide population health concern, but its etiology remains poorly understood. Tortoise-shell glue (TSG) is widely utilized as a pharmaceutical or dietary supplement for various health ailments. This study aimed to evaluate the effects of TSG on male infertility in obese rats.
Methods
A male infertility with obesity rat model was established after a high-fat diet (HFD) and cyclophosphamide (CTX) treatment. The body weight, testis weight, and weight of adipose tissues around the epididymis were detected. We then evaluated spermatozoon motility and concentration and testis pathological changes. Next, we assessed the hypothalamic-pituitary-gonadal (HPG) axis. Moreover, serum metabolomics was used to identify differential metabolites. The Spearman correlation analysis was employed to assess the correlation between differential metabolites and sex hormone levels.
Results
Here, we demonstrated that TSG alleviated obesity and male infertility. Meanwhile, TSG restored the function of the HPG axis in obese infertility rats, including mRNA levels of GnRH, GnRHR, RFRP-3, and kisspeptin in the hypothalamus and pituitary and sex hormone levels in the serum. In addition, 16 potentially significant metabolites, such as Quinapril and Malabaricone C, were identified as significant in the therapeutic effects of TSG on male infertility. The majority of these differential metabolites exhibited recovery following TSG treatment. The further Spearman analysis demonstrated the close correlation between differential metabolites and sex hormones, especially Kamahine C.
Conclusion
TSG attenuated obesity and male infertility in rats through the HPG axis by regulating serum metabolites. It shows potential as a viable treatment option for male infertility.
1. Introduction
Obesity is associated with a range of comorbidities, including psychological, mechanical, and metabolic disorders. These comorbidities can result in dysfunction of the female and male gonads, as well as an increased risk for type 2 diabetes mellitus, dyslipidemia, hypertension, and cardiovascular disorders (Csige et al. Citation2018; Michelet et al. Citation2018; Quail and Dannenberg Citation2019; Genchi et al. Citation2022). Without exception, obesity is now affecting all countries, and this impact is projected to become even more significant in the current decade. Consequently, there will be an increase in the number of years lost due to poor health, disability, and mortality (World Obesity Federation Global Obesity Observatory. World obesity atlas Citation2022 Citation2022). Meanwhile, the prevalence of obesity among men of reproductive age has exhibited a consistent upward trend in recent decades, coinciding with a simultaneous decrease in male fertility (Ameratunga et al. Citation2023). There exists a significant body of evidence indicating a correlation between obesity and diminished male fertility (Liu and Ding Citation2017). Indeed, a significant proportion of male subfertility and infertility, approximately 20%, can be directly linked to the presence of overweight and obesity (Carrageta et al. Citation2019). Mechanisms through which obesity can potentially impact spermatogenesis encompass thermal effects, hyperestrogenism, hypogonadotropic hypogonadism, diabetes mellitus, sexual dysfunction, and disturbances in sperm epigenetics (Barbagallo et al. Citation2021; Keyhan et al. Citation2021; Maděrka Citation2023). However, there are no precise conclusions yet.
Traditional Chinese medicine (TCM) has a long-standing history of addressing obesity and male infertility by employing pharmacological agents sourced from natural origins, such as herbal or animal tissue-derived materials (Zhou et al. Citation2019; Zhang et al. Citation2023). Tortoise-shell glue (TSG), a traditional component of TCM, is widely utilized as a medicinal substance or dietary supplement for various health conditions. (Su et al. Citation2018; Sheng et al. Citation2022). From TCM theory, TSG can nourish the liver and kidneys, strengthen the muscles and bones, and nourish the essence and blood. A prior study has found that TSG could promote bone regeneration in rats and zebrafish (Su et al. Citation2018). In our previous study, a substantial dosage of TSG treatment exhibited encouraging therapeutic outcomes on the process of spermatogenesis in rats with oligoasthenospermia (OA), as evidenced by a notable increase in spermatozoon density, motility, and testosterone (T) production (Sheng et al. Citation2022). Nevertheless, whether TSG could perform therapeutic effects on male infertility in obese rats remains unclear.
Among the many factors by which obesity affects spermatogenesis, hypogonadotropic hypogonadism has been recognized as the primary contributor to male infertility, which results from abnormal regulation of the hypothalamic-pituitary-gonadal (HPG) axis (Manaserh et al. Citation2019; Spaziani et al. Citation2023). The HPG axis is an important neuroendocrine axis that regulates the function of the reproductive system. The main function of this axis is to regulate and coordinate the secretion of gonadal hormones and reproductive function. It is of paramount importance in many processes related to the development, maturation, and aging of the male (Corradi et al. Citation2016). A large number of studies have reported the therapeutic effects on male infertility by modulating the HPG axis, such as aromatase inhibitors (Yang et al. Citation2021), gonadotropin (Boeri et al. Citation2021), Tamoxifen (Dimakopoulou et al. Citation2021), and so on. However, it is still unclear whether TSG could exert therapeutic effects on male infertility through the HPG axis in obese rats.
In the present study, we established a male infertile rat model, and at the same time administered TSG intervention to explore its potential mechanism of therapeutic action, which provided new insights into the treatment of male infertility.
2. Materials and methods
2.1. Animal experiments
Male specific-pathogen-free Sprague Dawley rats, weighing between 230-250 g, were obtained from Hunan SJA Laboratory Animal Co., LTD (Changsha, China). The rats were housed in a pathogen-free facility with controlled environmental conditions, including a 12-h light/dark cycle, a temperature of 22 ± 1°C, and a humidity level of approximately 50%. All the rats were provided with unrestricted access to food and water and were allowed to acclimate for a duration of one week.
Rats were randomly assigned to four groups (n = 5 per group): Control group, HFD group, HFD + CTX group, and HFD + CTX + TSG group. The Control group and the other three groups were fed a standard-fat diet (1022, Beijing HFK Bioscience, Beijing, China; SFD) and a high-fat diet (XTHF60, Xietong Bio-engineering, Nanjing, China; HFD) for six weeks, respectively. Meanwhile, cyclophosphamide (CTX) (30 mg/kg/week, dissolved in saline, Meilunbio, Dalian, China) was administered to induce infertility by intraperitoneal injection once a week for six weeks in the HFD + CTX and HFD + CTS + TSG groups (Sheng et al. Citation2023). Subsequently, based on our previous studies (Su et al. Citation2018; Sheng et al. Citation2022; Sheng et al. Citation2023), TSG (1.25 g/kg/day, dissolved in saline, Hubei Kangyuan Pharmaceutical Co., LTD, Wuhan, China) was intragastrically administered once daily for six weeks. Body weight was detected every week. After six weeks, peripheral blood was collected for further assays. Subsequently, rats were injected intravenously with barbiturates (150 mg/kg) and then sacrificed. The weights of testis and adipose tissues around the epididymis were detected. The testis, epididymal, hypothalamus, and pituitary tissues were collected for subsequent molecular biology and morphological analysis. The animal experimental protocol was approved by the Ethics Committee of Hunan University of Chinese Medicine (LLBH-202304030005).
2.2. Spermatozoon motility and concentration analysis
Cauda epididymal spermatozoa of rats were collected and prepared according to the methodology outlined in a previous report (Wang et al. Citation2013). In brief, two caudal epididymis were placed in the HEPES medium. The epididymis cauda was partitioned into three segments and subsequently subjected to incubation at 37°C for 10 min in a 5% CO2 incubator. The spermatozoon underwent a gentle filtration process using nylon gauze, followed by centrifugation and subsequent resuspension in 1 mL of fresh M199 medium. Next, computer-assisted sperm analysis (CASA) was performed using the HST sperm quality analysis system Sperm Tracker (NatureGene Corp., NJ, USA). For each sample, three independent cameras were used and a total of 18 random fields (six fields/camera) were counted. The spermatozoon motility and concentration were measured automatically, including progressive motility rate (PR, %), non-progressive motility rate (NP, %), total motility rate (PR + NP, %), and concentration (106/mL).
2.3. Histological examination
Hematoxylin-eosin (HE) staining was employed to assess morphological changes in testicular tissues. Briefly, paraffin-embedded tissue sections were roasted and dried for 12 h at 60°C, followed by immersion in xylene for 20 min three times. Subsequently, the sections were immersed in ethanol solutions of varying concentrations (100%, 100%, 95%, 85%, and 75%) for 5 min, and then rinsed with distilled water for 5 min. Sequentially, the sections were successively stained with hematoxylin (AWI0020, Abiowell, Changsha, China) and eosin (AWI0020, Abiowell). The sections were then dehydrated by a gradient of alcohol (95-100%) for 5 min per stage, followed by immersion in xylene for 10 min, repeated twice. The sections were finally sealed with neutral gum and examined under the optical microscope (BA210 T, Motic, Xiamen, China).
2.4. Quantitative real-time PCR (qRT-PCR)
The mRNA levels of gonadotropin-releasing hormone (GnRH), GnRH receptor (GnRHR), RF amide-related peptide-3 (RFRP-3), and kisspeptin were assessed using qRT-PCR. Total RNA samples were extracted from the hypothalamus and pituitary tissues using Trizol reagent (15596026, Thermo Fisher Scientific) in accordance with the manufacturer's instructions. Correspondingly, the process of reverse transcription was employed to convert mRNA into cDNA, utilizing the mRNA reverse transcription kit (CW2569, CWBIO, Beijing, China). The qRT-PCR was conducted by the SYBR method using Ultra SYBR Mixture (CW2601, CWBIO). The experiments were conducted using a fluorescence quantitative PCR instrument (Thermo Fisher Scientific). Primers were designed utilizing Primer 5 software (PREMIER Biosoft, San Francisco, CA, USA) (). β-actin was used as a reference gene. Data analysis was conducted using the 2-ΔΔCt method.
Table 1. Sequences of the primers.
2.5. Enzyme-linked immunosorbent assay (ELISA) analysis
The serum was obtained from peripheral blood through a centrifugation process at 1000 × g for 15 min. The concentrations of luteinizing hormone (LH), follicle-stimulating hormone (FSH), prolactin (PRL), estradiol (E2), progesterone (PROG), T, GnRH, and leptin in the serum were detected using the rat LH kit (CSB-E12654r, CUSABIO, Wuhan, China), rat FSH kit (E-EL-R0391c, Elabscience, Wuhan, China), rat PRL kit (CSB-E06881r, CUSABIO), rat E2 kit (CSB-E05110r, CUSABIO), rat PROG kit (CSB-E07282r, CUSABIO), rat T kit (CSB-E05100r, CUSABIO), rat GnRH kit (CSB-E08037r, CUSABIO), and rat leptin kit (CSB-E07433r, CUSABIO) following the manufacturers’ instructions, respectively. The measurement of absorbance value at 450 nm was conducted using a Microplate Reader (Heales, Shenzhen, China).
2.6. Metabolomics analysis
Each sample was weighed about 50 mg and 400 μl of methanol solution (containing 5 μg/ml L-2-chloro-phenylalanine as an internal standard) was added. Next, the samples underwent homogenization for 3 min at 60 Hz, repeated twice. The samples were subsequently subjected to centrifugation at 13,000 rpm for 10 min at 4°C, resulting in the collection of the supernatant. An equal quantity of each sample to be tested was taken and mixed, and it was used as a Quality Control (QC) sample.
The Agilent 1290 Infinity UPLC system (Agilent Technologies, Santa Clara, CA, USA) in conjunction with the Agilent 6545 UHD and Accurate-Mass Q-TOF system (G6546AA, Agilent Technologies) was utilized for conducting the metabolomics analysis. The volume of the was 4 µL. Samples were separated through an XSelect HSS T3 column (2.1 × 100 mm with 2.5 μm particle size, 186006741, Waters, Milford, MA, USA). The column temperature was maintained at 40°C and the mobile phases used were 0.1% formate in H2O (mobile phase A) and 0.1% formate in acetonitrile (mobile phase B). The gradient commenced with 3 min of isocratic elution with 20% B (and 80% A). B was elevated to 95% within a span of 6 min and maintained at that level for 4 min. Rapidly, the mobile phase composition was adjusted back to 5% B within 0.1 min and maintained for 3 min to allow for column post-equilibration. The flow rate for the mobile phases was adjusted at 0.4 mL/min. The mass spectrometer was operated in either positive or negative information-dependent acquisition (IDA) modes. The instrument parameters used in the study were as follows: the gas temperature was 325°C; the nebulizer pressure was 20 psig; the capillary voltage was 4.5 kV in positive mode and −3.5 kV in negative mode; the drying gas flow was 8 I/min in positive mode and 10 I/min in negative mode; the fragmentor voltage was set to 120 V; the skimmer voltage was set to 45 V. The mass range was defined as m/z 50 to m/z 1,500.
Agilent Masshunter Qualitative Analysis B.08.00 software (Agilent Technologies) was utilized to extract the matched peak data from the LC-MS raw data. Additionally, the peak area data were normalized (Gong et al. Citation2019). Principal components analysis (PCA) of the normalized data was conducted using the R.vegan package. Based on variable importance (VIP) values (VIP ≥ 1) and t-test (P < 0.05), the selection of differential metabolites was conducted among four groups. The identification of these metabolites was performed using online databases: HMDB (http://www.hmdb.ca) and KEGG (http://www.kegg.jp).
2.7. Statistic analysis
All data were presented as the mean and standard deviation (SD) values of five independent experiments. Statistical analyses were conducted utilizing GraphPad Prism 8.0 (GraphPad Software Inc., San Diego, CA, USA). The one-way analysis of variance (ANOVA) was employed to compare multiple groups. The Spearman correlation analysis was employed to evaluate the correlation between differential metabolites and sex hormone levels. P < 0.05 was used to determine statistical significance.
3. Results
3.1. TSG ameliorated obesity and male infertility in HFD-induced obese rats
Initially, we investigated whether TSG regulated male infertility in HFD-induced obese rats. From our results, it was observed that the body weight, testis weight, and weight of adipose tissues around the epididymis in the HFD and HFD + CTX groups exhibited a significant increase compared with the Control group. However, TSG treatment significantly ameliorated those symptoms (A and B).
Figure 1. Effects of TSG treatment on obesity and male infertility. (A) Body weight changes of four groups were monitored once a week for six weeks. (B) The weights of testis and adipose tissues around the epididymis were detected after six weeks. (C) Representative spermatozoon tracks of four groups generated by CASA. Tracks color code: blue-curvilineal moving; green-linearly moving; red-immotile (excluded from analysis); Magnification 400 × . (D) The changes in spermatozoon concentration were evaluated using CASA. (E) The changes in progressive motility (PR, %) and total motility (PR + NP, %) of spermatozoa. (F) HE staining of rat testicular tissues to evaluate morphological changes. Data are expressed as mean ± SD (n = 5). *P < 0.05 vs. Control group, #P < 0.05 vs. HFD + CTX group. HFD, high-fat diet; CTX, Cyclophosphamide; TSG, tortoise-shell glue; PR, progressive motility rate; NP, non-progressive motility rate.
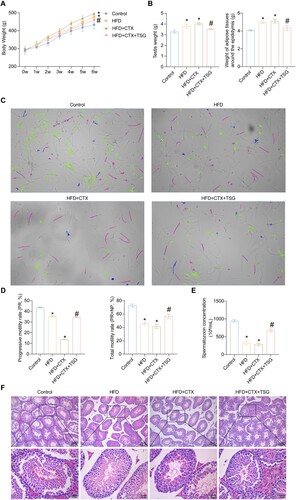
At present, the diagnostic assessment of male infertility is a topic of debate, as the semen analysis is considered the main but limited method for determining the extent of male infertility (Agarwal et al. Citation2021). Therefore, we performed spermatozoon motility trajectory analysis as well as motility and concentration assay using CASA technology. As shown in C, the majority of spermatozoa in the Control group displayed curvilineal moving (blue tracks) and linearly moving (green tracks), while there were scarce motile spermatozoa in the HFD + CTX group. After TSG treatment, the motile spermatozoa were increased. The spermatozoa movement movies exhibited consistent results (the videos are shown in Supplementary information). Additionally, the progressive motility, total motility, and concentration of spermatozoa in the cauda epididymis from the HFD group were remarkably reduced compared to the Control group, while they were further decreased in the HFD + CTX group. Nevertheless, they were remarkably restored after TSG treatment (D and E). Consistent with this, observation of the testicular tissues revealed that compared with the Control group, the HFD and HFD + CTX groups exhibited disorganized spermatid cells within the testicular seminiferous tubules. Additionally, nucleus rupture, cell detachment, and decreased spermatozoa were observed in the testicular cells. Nevertheless, compared with the HFD + CTX group, the germ cells at various stages of development in the HFD + CTX + TSG group exhibited a more organized and compact arrangement, and the seminiferous tubules contained a higher abundance of spermatozoa (F). These results revealed that TSG treatment mitigated the increased weights of body, testis, and adipose tissues around the epididymis induced by HFD and CTX and promoted the recovery and spermatogenesis of testicular tissues.
3.2. TSG restored the function of the HPG axis in HFD-induced obese rats with male infertility
Physiological changes caused by obesity, which have a negative impact on the male reproductive endocrine system, are primarily mediated through the HPG axis, the main endocrine regulator of reproductive functions (Leisegang et al. Citation2021). Therefore, gene transcriptions of the HPG axis were evaluated. GnRH from the hypothalamus is the final brain signal that regulates reproduction (Moenter Citation2018), while RFRP-3 and kisspeptin are widely recognized for their involvement in establishing a connection between metabolic and reproductive functions of hypothalamic neuroendocrine pathways (Wolfe and Hussain Citation2018; Singh et al. Citation2022). So we detected the expression of GnRH, RFRP-3, and kisspeptin in the hypothalamus and GnRHR, RFRP-3, and kisspeptin in the pituitary, the markers representing HPG axis activity. As depicted in A, mRNA levels of GnRH, RFRP-3, and kisspeptin in hypothalamus tissues significantly decreased in both the HFD and HFD + CTX groups when compared to the Control group. Notably, TSG treatment substantially augmented mRNA levels of these markers in the HFD + CTX + TSG group, as compared to the HFD + CTX group. Similarly, the combination of HFD and CTX significantly attenuated the mRNA levels of GnRHR, RFRP-3, and kisspeptin in pituitary tissues. The HFD + CTX-induced decrease of these molecules was also ameliorated to some extent in the presence of TSG (B). Moreover, we detected sex hormone levels in the serum to evaluate the function of gonadal, including LH, FSH, PRL, E2, PROG, and T. The findings of our study revealed that compared with the Control group, LH and FSH levels were increased in both the HFD and HFD + CTX groups while E2, PROG, and T levels were decreased. As expected, TSG treatment substantially mitigated these changes in the HFD + CTX + TSG group compared to the HFD + CTX group. Besides, there was no statistically significant variation observed in the PRL levels among the four groups (C). Furthermore, leptin is a kind of hormone that plays a crucial role in the regulation of body weight, and it also has significant implications for reproductive function (Khodamoradi et al. Citation2020). Excessive production of leptin plays a crucial role in the onset of androgen insufficiency and diminished reproductive function among men with obesity (Childs et al. Citation2021). Thus, expression levels of GnRH and leptin in the serum were detected to further explore the function of the HPG axis. We observed a significant increase in GnRH levels and a significant decrease in leptin levels in the presence of TSG, as compared to the HFD + CTX group (D). These data indicated from various aspects that TSG significantly restored the function of the HPG axis in obese rats with infertility.
Figure 2. Effects of TSG treatment on HPG axis in obese rats with male infertility. (A) The mRNA levels of GnRH, RFRP-3, and kisspeptin in the hypothalamus tissues of the four groups using qRT-PCR. (B) The mRNA levels of GnRHR, RFRP-3, and kisspeptin in the pituitary tissues of the four groups using qRT-PCR. (C) Expression levels of LH, FSH, PRL, E2, PROG, and T in the serum of the four groups using ELISA. (D) Expression levels of GnRH and leptin in the serum of the four groups using ELISA. Data are expressed as mean ± SD (n = 5). *P < 0.05 vs. Control group, #P < 0.05 vs. HFD + CTX group. HFD, high-fat diet; CTX, Cyclophosphamide; TSG, tortoise-shell glue; GnRH, gonadotropin-releasing hormone; RFRP-3, RF amide-related peptide-3; GnRHR, GnRH receptor; LH, luteinizing hormone; FSH, follicle-stimulating hormone; PRL, prolactin; E2, estradiol; PROG, progesterone; T, testosterone.
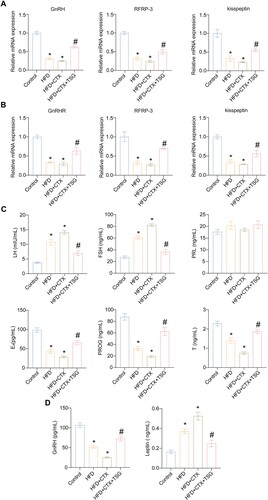
3.3. TSG mediated serum metabolism in obese rats with infertility
Previous studies have reported that serum metabolism reflects the activity of the HPG axis (da Costa et al. Citation2021; Luo et al. Citation2022). To understand the metabolic effects of TSG against male infertility through the HPG axis, we detected and analyzed differential metabolites in serum samples from rats. To investigate the separation among the Control, HFD, HFD + CTX, and HFD + CTX + TSG groups, we performed PCA. As depicted in A, PCA demonstrated that samples belonging to the same group exhibited a tendency to cluster together, whereas samples from distinct groups were clearly discernible from one another. Based on the HMDB database, a total of 993 different metabolites were identified between the Control and HFD + CTX groups, with 580 metabolites up-regulated and 413 metabolites down-regulated. Additionally, we identified 225 different metabolites between the HFD + CTX and HFD + CTX + TSG groups, with 142 metabolites up-regulated and 83 metabolites down-regulated (B). To visually represent the differences in metabolite levels among the four groups, we generated a heat map. As depicted in C, levels of large amounts of candidate metabolites were altered in the HFD and HFD + CTX groups compared with the Control group. However, in the HFD + CTX + TSG group, most of these alterations were reversed, such as 2-Piperidinone, Kamahine C, and (S)-Spinacine, indicating that TSG treatment alleviated metabolic perturbations.
Figure 3. Changes in the serum metabolomics profiles of four groups. (A) PCA model was used to distinguish the difference between groups. (B) Volcano plots showing trends in metabolite expression differences. (C) The heat map of potential metabolites from four groups. HFD, high-fat diet; CTX, Cyclophosphamide; TSG, tortoise-shell glue.
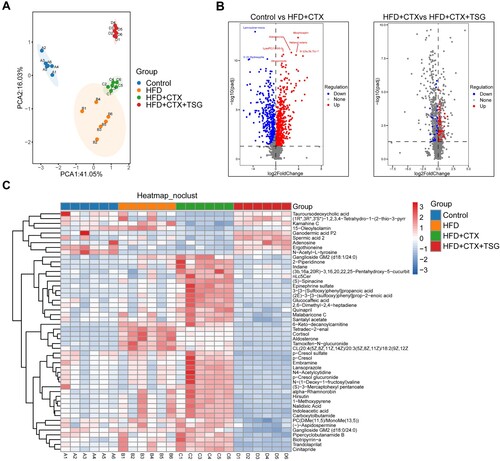
3.4. TSG regulated serum metabolites in obese rats with infertility
Next, according to VIP values from four groups, the top 16 key differential metabolites were identified (). It showed that the combination of HFD and CTX regulated the biomarkers, including 2-Piperidinone, Kamahine C, (S)-Spinacine, Trandolaprilat, Cinitapride, 3-[3-(Sulfooxy)phenyl]propanoic acid, (2E)−3-[3-(sulfooxy)phenyl]prop-2-enoic acid, Quinapril, Malabaricone C, 1-Methoxypyrene, Nalidixic Acid, Hirsutin, (S)−3-Mercaptohexyl pentanoate, Indoleacetic acid, Pipercyclobutanamide B, and Biotripyrrin-a. Compared with the HFD + CTX group, the addition of TSG significantly restored most of these metabolites. The data indicated that TSG may regulate infertility by the HPG axis in obese rats through these differential metabolites.
Figure 4. The relative content changes of serum metabolites. Bar graph of intergroup abundance variation of 16 differential metabolites. Data are expressed as mean ± SD (n = 6). *P < 0.05 vs. Control group, #P < 0.05 vs. HFD + CTX group. HFD, high-fat diet; CTX, Cyclophosphamide; TSG, tortoise-shell glue.
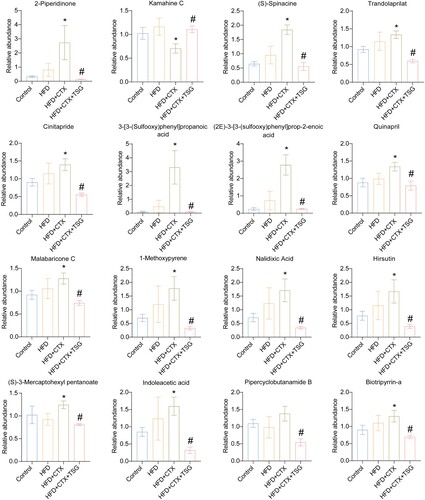
3.5. The Spearman correlation between the differential serum metabolites and sex hormones levels
To explore the relationship between the differential metabolites and sex hormones levels in obese rats with infertility, based on the previous assay and metabolomics data, we subsequently performed a Spearman correlation analysis. As shown in , 2-Piperidinone, (S)-Spinacine, Trandolaprilat, Cinitapride, 3-[3-(Sulfooxy)phenyl]propanoic acid, (2E)−3-[3-(sulfooxy)phenyl]prop-2-enoic acid, Quinapril, Malabaricone C, 1-Methoxypyrene, Nalidixic Acid, Hirsutin, Indoleacetic acid, and Biotripyrrin-a exhibited positive correlations with levels of LH and FSH, while displaying negative correlations with levels of E2, PROG, and T. Kamahine C demonstrated positive correlations with levels of PRL, E2, PROG, and T and negative correlations with levels of LH and FSH. (S)−3-Mercaptohexyl pentanoate demonstrated a negative correlation with the level of PRL. Pipercyclobutanamide B was found to have positive correlations with levels of LH and FSH while showing negative correlations with PRL, PROG, and T. These findings showed that the modulatory effect of TSG on the HPG axis is linked to a specifically altered serum-metabolic axis, especially Kamahine C.
Figure 5. The Spearman correlation between the differential metabolites and levels of sex hormones. The color blue is used to represent a positive correlation, while the color pink is used to represent a negative correlation. The intensity of the color is directly proportional to the magnitude of the Spearman correlation. *P < 0.05, **P < 0.01.
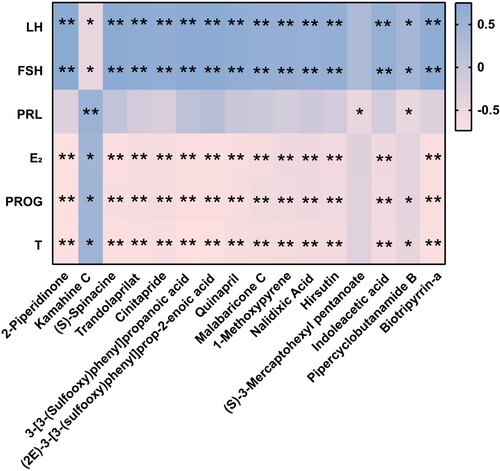
4. Discussion
TCM has been employed as a therapeutic approach for addressing infertility over an extended period of time. Sun et al. conducted a clinical study aimed at evaluating the efficacy of Yikang decoction, a TCM formula, in the treatment of male immune infertility. The results from Sun et al. showed that Yikang decoction markedly ameliorated spermatozoon density and semen liquidation, as compared to the baseline. The effect of Yikang decoction was found to be more pronounced in all parameters assessed, when compared to the treatment with prednisone, a commonly used clinical drug for infertility (Sun and Bao Citation2006). In addition, Berberine (Wu et al. Citation2016) and Trigonella foenum-graecum seed extract (Swaroop et al. Citation2015) have been found to exert therapeutic effects on obese polycystic ovary syndrome (PCOS) patients with infertility to some extent. Furthermore, Dong et al. revealed that Huatan Shengjing decoction reduced body weight and blood lipid levels and improved spermatozoon quality in HFD-fed rats by regulating PI3 K/Akt and MAPK signaling pathways (Dong et al. Citation2022). Our previous study has revealed that the treatment of TSG, which is one of the classic TCMs, promoted the spermatogenesis process in OA rats (Sheng et al. Citation2022). Therefore, we wondered that weather TSG has therapeutic effects on obese rats with male infertility. As expected, our data showed that TSG enhanced spermatozoon motility and concentration, leading to the promotion of the recovery and spermatogenesis of testicular tissues in this study. Consequently, it could be inferred that TSG may provide a good therapeutic effect on male infertility clinically.
The normal functioning of the HPG axis is crucial for maintaining the health of the reproductive system. There is a study that reported that the up-regulated activation of the central HPG axis can be epigenetically altered, which can lead to PCOS with obesity and infertility (Walters et al. Citation2018). Therefore, we surmised that TSG may exert therapeutic effects on male infertility in obese rats through the HPG axis. In the current study, it was observed that the activity of the HPG axis was diminished, resulting in a significant decrease in the levels of LH, FSH, and leptin and a significant increase in the levels of E2, PROG, T, and GnRH induced by HFD and CTX. Additionally, TSG restored the levels of these sex hormones by activating the HPG axis, thereby promoting reproductive function. Consistent with our data, Tekin et al. have found reduced levels of GnRH, LH, FSH, and T in male infertility rats induced by irisin administration (Tekin et al. Citation2019). Furthermore, other reports have confirmed that the reproductive function relies on the proper functioning of the HPG axis (Maggi et al. Citation2016; Barber et al. Citation2021). Obese males exhibit elevated levels of estrogen in comparison to T levels. Reduced circulating T levels inhibit the HPG axis, consequently causing erectile dysfunction and decreased libido, which contributes to infertility commonly observed in males with obesity (Rao et al. Citation2013). Collectively, TSG could activate the HPG axis, regulate the levels of sex hormones, and thereby restore male infertility in obese rats.
Previous study has indicated that the analysis of serum metabolomics has the potential to identify distinct metabolic patterns associated with non-obstructive azoospermia (Zhang et al. Citation2017). Additionally, it could provide specific differences in metabolic characteristics of the HPG axis and reproductive system (Wu et al. Citation2023). Nevertheless, TSG-induced metabolic alterations remain largely unknown. Therefore, in the present study, the serum metabolomics analysis was performed to further investigate the therapeutic effects of TSG on male infertility in obese rats. Our results demonstrated that TSG could increase the activity of the HPG axis through multiple significant metabolites. Among them, Quinapril, a robust angiotensin-converting enzyme (ACE) inhibitor, has been shown to induce positive haemodynamic changes and enhance ventricular and endothelial function across various cardiovascular disorders (Wilson et al. Citation2023). Malabaricone C, a natural inhibitor of sphingomyelin synthase, has the potential as a protective agent against diet-induced obesity and its impact on lipid metabolism in mice (Othman et al. Citation2019). In addition, Hodges et al. have found that Nalidixic Acid improved glucose tolerance in HFD-induced obese mice (Hodges et al. Citation2022). From our metabolic results, we observed robust associations between sex hormone levels and several significant metabolites. For instance, Quinapril, Malabaricone C, and Nalidixic Acid exhibited a positive correlation with levels of LH and FSH, while displaying a negative correlation with levels of E2, PROG, and T. These data indicated that TSG may regulate the HPG axis as well as male infertility through these key metabolites.
There are certain limitations to the present study. Firstly, additional experiments are required to validate the precise mechanisms by which TSG regulates the HPG axis. Secondly, global metabolic changes in other organizations, such as the testis, should be determined in future studies, in order to provide a more comprehensive understanding of the mechanism by which TSG influences anti-infertility. In addition, enhanced metabolomics technologies, such as targeted metabolomics, are necessary to purposefully identify metabolite biomarkers. Furthermore, according to our findings, HFD caused infertility in rats to some extent. Our future research directions may be to extend the duration of HFD, in order to explore whether prolonged HFD could cause male infertility and whether TSG has a therapeutic effect on obesity-related infertility in rats so that regulatory relations between them will be more clear.
5. Conclusion
In conclusion, TSG was able to ameliorate obesity and male infertility induced by HFD and CTX. These biological effects of TSG on obese rats with male infertility were associated with HPG axis function. Moreover, we identified the serum metabolic responses toward TSG therapy in male infertility rats and revealed strong correlations between levels of sex hormones and significant metabolites, such as Quinapril, Malabaricone C, and Nalidixic Acid. These results indicated that TSG possesses anti-male infertility potential, which makes it an attractive candidate for future development.
Authors’ contributions
Conceptualization: WS; Methodology: YL; Data curation, Formal analysis, and Visualization: WS, YL, LL, BL, TS, and CZ; Project administration: QH; Writing-original draft: WS and YL; Writing-review & editing: QH. All authors agree to be accountable for the content of the work.
Availability of data and materials
The data supporting the conclusion are included in the article.
Ethics approval
The animal experimental protocol was approved by the Ethics Committee of Hunan University of Chinese Medicine (LLBH-202304030005).
Supplemental Material
Download Zip (22.5 MB)Disclosure statement
No potential conflict of interest was reported by the author(s).
Additional information
Funding
References
- Agarwal A, Baskaran S, Parekh N, Cho CL, Henkel R, Vij S, Arafa M, Panner Selvam MK, Shah R. 2021. Male infertility. Lancet. 397(10271):319–333. doi:10.1016/S0140-6736(20)32667-2.
- Ameratunga D, Gebeh A, Amoako A. 2023. Obesity and male infertility. Best Pract Res Clin Obstet Gynaecol. 90:102393. doi:10.1016/j.bpobgyn.2023.102393.
- Barbagallo F, Condorelli RA, Mongioì LM, Cannarella R, Cimino L, Magagnini MC, Crafa A, La Vignera S, Calogero AE. 2021. Molecular mechanisms underlying the relationship between obesity and male infertility. Metabolites. 11(12):840. doi:10.3390/metabo11120840.
- Barber TM, Kyrou I, Kaltsas G, Grossman AB, Randeva HS, Weickert MO. 2021. Mechanisms of central hypogonadism. Int J Mol Sci. 22(15):8217. doi:10.3390/ijms22158217.
- Boeri L, Capogrosso P, Salonia A. 2021. Gonadotropin treatment for the male hypogonadotropic hypogonadism. Curr Pharm Des. 27(24):2775–2783. doi:10.2174/1381612826666200523175806.
- Carrageta DF, Oliveira PF, Alves MG, Monteiro MP. 2019. Obesity and male hypogonadism: Tales of a vicious cycle. Obes Rev. 20(8):1148–1158. doi:10.1111/obr.12863.
- Childs GV, Odle AK, MacNicol MC, MacNicol AM. 2021. The importance of leptin to reproduction. Endocrinology. 162(2):bqaa204. doi:10.1210/endocr/bqaa204.
- Corradi PF, Corradi RB, Greene LW. 2016. Physiology of the hypothalamic pituitary gonadal axis in the male. Urol Clin North Am. 43(2):151–162. doi:10.1016/j.ucl.2016.01.001.
- Csige I, Ujvárosy D, Szabó Z, Lőrincz I, Paragh G, Harangi M, Somodi S. 2018. The Impact of Obesity on the Cardiovascular System. J Diabetes Res. 2018:3407306.
- da Costa CS, Oliveira TF, Freitas-Lima LC, Padilha AS, Krause M, Carneiro M, Salgado BS, Graceli JB. 2021. Subacute cadmium exposure disrupts the hypothalamic-pituitary-gonadal axis, leading to polycystic ovarian syndrome and premature ovarian failure features in female rats. Environ Pollut. 269:116154. doi:10.1016/j.envpol.2020.116154.
- Dimakopoulou A, Foran D, Jayasena CN, Minhas S. 2021. Stimulation of leydig and sertoli cellular secretory function by anti-oestrogens: tamoxifen. Curr Pharm Des. 27(23):2682–2691. doi:10.2174/1381612826666200213095228.
- Dong Y, Zheng Y, Zhu L, Li T, Guan Y, Zhao S, Wang Q, Wang J, Li L. 2022. Hua-Tan-Sheng-Jing decoction treats obesity With oligoasthenozoospermia by Up-regulating the PI3K-AKT and down-regulating the JNK MAPK signaling pathways: At the crossroad of obesity and oligoasthenozoospermia. Front Pharmacol. 13:896434. doi:10.3389/fphar.2022.896434.
- Genchi VA, Rossi E, Lauriola C, D'Oria R, Palma G, Borrelli A, Caccioppoli C, Giorgino F, Cignarelli A. 2022. Adipose tissue dysfunction and obesity-related male hypogonadism. Int J Mol Sci. 23(15):8194. doi:10.3390/ijms23158194.
- Gong W, Zhu S, Chen C, Yin Q, Li X, Du G, Zhou Y, Qin X. 2019. The anti-depression effect of angelicae sinensis radix Is related to the pharmacological activity of modulating the hematological anomalies. Front Pharmacol. 10:192. doi:10.3389/fphar.2019.00192.
- Hodges WT, Jarasvaraparn C, Ferguson D, Griffett K, Gill LE, Chen Y, Ilagan MXG, Hegazy L, Elgendy B, Cho K, et al. 2022. Mitochondrial pyruvate carrier inhibitors improve metabolic parameters in diet-induced obese mice. J Biol Chem. 298(2):101554. doi:10.1016/j.jbc.2021.101554.
- Keyhan S, Burke E, Schrott R, Huang Z, Grenier C, Price T, Raburn D, Corcoran DL, Soubry A, Hoyo C, et al. 2021. Male obesity impacts DNA methylation reprogramming in sperm. Clin Epigenetics. 13(1):17. doi:10.1186/s13148-020-00997-0.
- Khodamoradi K, Parmar M, Khosravizadeh Z, Kuchakulla M, Manoharan M, Arora H. 2020. The role of leptin and obesity on male infertility. Curr Opin Urol. 30(3):334–339. doi:10.1097/MOU.0000000000000762.
- Leisegang K, Sengupta P, Agarwal A, Henkel R. 2021. Obesity and male infertility: Mechanisms and management. Andrologia. 53(1):e13617.
- Liu Y, Ding Z. 2017. Obesity, a serious etiologic factor for male subfertility in modern society. Reproduction. 154(4):R123–R131. doi:10.1530/REP-17-0161.
- Luo Y, Qiao X, Xu L, Huang G. 2022. Irisin: circulating levels in serum and its relation to gonadal axis. Endocrine. 75(3):663–671. doi:10.1007/s12020-022-02981-5.
- Maděrka M. 2023. Obesity and assisted reproduction. Ceska Gynekol. 88(3):200–208. doi:10.48095/cccg2023200.
- Maggi R, Cariboni AM, Marelli MM, Moretti RM, Andrè V, Marzagalli M, Limonta P. 2016. GnRH and GnRH receptors in the pathophysiology of the human female reproductive system. Hum Reprod Update. 22(3):358–381. doi:10.1093/humupd/dmv059.
- Manaserh IH, Chikkamenahalli L, Ravi S, Dube PR, Park JJ, Hill JW. 2019. Ablating astrocyte insulin receptors leads to delayed puberty and hypogonadism in mice. PLoS Biol. 17(3):e3000189. doi:10.1371/journal.pbio.3000189.
- Michelet X, Dyck L, Hogan A, Loftus RM, Duquette D, Wei K, Beyaz S, Tavakkoli A, Foley C, Donnelly R, et al. 2018. Metabolic reprogramming of natural killer cells in obesity limits antitumor responses. Nat Immunol. 19(12):1330–1340. doi:10.1038/s41590-018-0251-7.
- Moenter SM. 2018. Gnrh neurons on LSD: a year of rejecting hypotheses that May have made karl popper proud. Endocrinology. 159(1):199–205. doi:10.1210/en.2017-03040.
- Othman MA, Yuyama K, Murai Y, Igarashi Y, Mikami D, Sivasothy Y, Awang K, Monde K. 2019. Malabaricone C as natural sphingomyelin synthase inhibitor against diet-induced obesity and Its lipid metabolism in mice. ACS Med Chem Lett. 10(8):1154–1158. doi:10.1021/acsmedchemlett.9b00171.
- Quail DF, Dannenberg AJ. 2019. The obese adipose tissue microenvironment in cancer development and progression. Nat Rev Endocrinol. 15(3):139–154. doi:10.1038/s41574-018-0126-x.
- Rao PM, Kelly DM, Jones TH. 2013. Testosterone and insulin resistance in the metabolic syndrome and T2DM in men. Nat Rev Endocrinol. 9(8):479–493. doi:10.1038/nrendo.2013.122.
- Sheng W, Xu W, Ding J, Lu B, Liu L, He Q, Zhou Q. 2023. Guijiajiao (Colla Carapacis et Plastri, CCP) prevents male infertility via gut microbiota modulation. Chin J Nat Med. 21(6):403–410.
- Sheng W, Xu W, Hu H, Xiao D, He Q. 2022. Effects of tortoise-shell glue on rat oligoasthenospermia model. Chin Med J (Engl). 135(6):732–734. doi:10.1097/CM9.0000000000001658.
- Singh P, Anjum S, Srivastava RK, Tsutsui K, Krishna A. 2022. Central and peripheral neuropeptide RFRP-3: a bridge linking reproduction, nutrition, and stress response. Front Neuroendocrinol. 65:100979. doi:10.1016/j.yfrne.2022.100979.
- Spaziani M, Carlomagno F, Tarantino C, Angelini F, Vincenzi L, Gianfrilli D. 2023. New perspectives in functional hypogonadotropic hypogonadism: beyond late onset hypogonadism. Front Endocrinol (Lausanne). 14::1184530.
- Su W, Feng C, Tseng C, Hung H, Chen Z, Lee H, Jean Y, Wu SS, Wen Z. 2018. Therapeutic effect of Guijiajiao (Colla Carapacis et Plastri) on bone regeneration in rats and zebrafish. J Tradit Chin Med. 38(2):197–210. doi:10.1016/j.jtcm.2018.04.006.
- Sun Z, Bao Y. 2006. TCM treatment of male immune infertility–a report of 100 cases. J Tradit Chin Med. 26(1):36–38.
- Swaroop A, Jaipuriar AS, Gupta SK, Bagchi M, Kumar P, Preuss HG, Bagchi D. 2015. Efficacy of a novel Fenugreek seed extract (Trigonella foenum-graecum, Furocyst) in polycystic ovary syndrome (PCOS). Int J Med Sci. 12(10):825–831. doi:10.7150/ijms.13024.
- Tekin S, Beytur A, Erden Y, Beytur A, Cigremis Y, Vardi N, Turkoz Y, Tekedereli I, Sandal S. 2019. Effects of intracerebroventricular administration of irisin on the hypothalamus-pituitary-gonadal axis in male rats. J Cell Physiol. 234(6):8815–8824. doi:10.1002/jcp.27541.
- Walters KA, Gilchrist RB, Ledger WL, Teede HJ, Handelsman DJ, Campbell RE. 2018. New perspectives on the pathogenesis of PCOS: neuroendocrine origins. Trends Endocrinol Metab. 29(12):841–852. doi:10.1016/j.tem.2018.08.005.
- Wang YN, Wang B, Liang M, Han CY, Zhang B, Cai J, Sun W, Xing GG. 2013. Down-regulation of CatSper1 channel in epididymal spermatozoa contributes to the pathogenesis of asthenozoospermia, whereas up-regulation of the channel by Sheng-Jing-San treatment improves the sperm motility of asthenozoospermia in rats. Fertil Steril. 99(2):579–587. doi:10.1016/j.fertnstert.2012.10.030.
- Wilson AJ, Sands GB, Wang VY, Pontre B, Ennis DB, Young AA, LeGrice IJ, Nash MP. 2023. Quinapril treatment curtails decline of global longitudinal strain and mechanical function in hypertensive rats. J Hypertens. 41(10):1606–1614. doi:10.1097/HJH.0000000000003512.
- Wolfe A, Hussain MA. 2018. The emerging role(s) for kisspeptin in metabolism in mammals. Front Endocrinol (Lausanne). 9:184. doi:10.3389/fendo.2018.00184.
- World Obesity Federation Global Obesity Observatory. 2022. World Obesity Atlas. 2022. London: World Obesity Federation. [accessed]. https://data.worldobesity.org/publications/?cat=15.
- Wu J, Wen L, Chen J, Chang Y, Huang R, Lin Y, Shen G, Feng J. 2023. Discover boy specific-biomarkers and reveal gender-related metabolic differences in central precocious puberty. J Steroid Biochem Mol Biol. 231:106305. doi:10.1016/j.jsbmb.2023.106305.
- Wu XK, Wang YY, Liu JP, Liang RN, Xue HY, Ma HX, Shao XG, Ng EH. 2016. Randomized controlled trial of letrozole, berberine, or a combination for infertility in the polycystic ovary syndrome. Fertil Steril. 106(3):757–765.e1.e751. doi:10.1016/j.fertnstert.2016.05.022.
- Yang C, Li P, Li Z. 2021. Clinical application of aromatase inhibitors to treat male infertility. Hum Reprod Update. 28(1):30–50. doi:10.1093/humupd/dmab036.
- Zhang Q, Bai Y, Wang W, Li J, Zhang L, Tang Y, Yue S. 2023. Role of herbal medicine and gut microbiota in the prevention and treatment of obesity. J Ethnopharmacol. 305:116127. doi:10.1016/j.jep.2022.116127.
- Zhang Z, Zhang Y, Liu C, Zhao M, Yang Y, Wu H, Zhang H, Lin H, Zheng L, Jiang H. 2017. Serum metabolomic profiling identifies characterization of Non-obstructive azoospermic Men. Int J Mol Sci. 18(2):238. doi:10.3390/ijms18020238.
- Zhou SH, Deng YF, Weng ZW, Weng HW, Liu ZD. 2019. Traditional Chinese medicine as a remedy for male infertility: a review. World J Mens Health. 37(2):175–185. doi:10.5534/wjmh.180069.