ABSTRACT
The system forming ovarian follicles is developed to investigate in vitro folliculogenesis in a confined environment to obtain functional oocytes. Several studies have reported the successful generation of fully functional oocytes using mouse-induced pluripotent stem cells (iPSCs) and mouse female germline stem cells (fGSCs) as sources of stem cells for in vitro gametogenesis models. In addition, human oogonia have been generated through heterologous co-culture of differentiated human primordial germ cell-like cells (hPGCLCs) with mouse germline somatic cells, although oocyte formation remains challenging. Thus, studies on in vitro ovarian formation in other species are utilized as an introductory approach for in vitro mammalian gametogenesis by understanding the differences in culture systems between species and underlying mechanisms. In this study, we optimized the method of the entire oogenesis process from rat embryonic gonads. We identified well-maturated MII oocytes from rat gonads using our constructed method. Moreover, we generated the first successful in vitro reconstitution of xenogeneic follicles from mouse primordial germ cells (PGCs) and rat somatic cells. We also established an appropriate culture medium and incubation period for xenogeneic follicles. This method will be helpful in studies of xenogeneic follicular development and oocyte generation.
Introduction
Reconstitute the entire gametogenesis process in culture is one of the major goals in developmental and reproductive biology. Many researchers have done a lot of research to generate oocytes from mouse pluripotent stem cells and female germline stem cells (fGSCs) in vitro from the 2000s to the present (Hübner et al. Citation2003; Hayashi et al. Citation2011; Hayashi et al. Citation2012; Nakaki et al. Citation2013; Kurimoto et al. Citation2015; Morohaku et al. Citation2016; Hayashi et al. Citation2017). Hubner et al. reported a culture method for producing oocytes using mouse embryonic stem cells (ESCs) in 2003 (Hübner et al. Citation2003). Obata et al. reported the construction of oocytes using the gonads of 12.5dpc (day post-coitum) mouse embryo (Morohaku et al. Citation2016), and Hayashi et al. reported the generation of oocytes through the aggregation of mouse Primordial germ cell-like cells (mPGCLCs) and mouse germline somatic cells (Hikabe et al. Citation2016). The advance of the in vitro follicle development system through the co-culture of mouse primordial germ cells (mPGCs) and mouse germline somatic cells enables the formation of imitated ovaries in vivo, allowing the formation of oocytes and embryonic development. Moreover, in vitro studies of oogenesis from follicles have been conducted in bovine (Gupta et al. Citation2008; Gupta and Nandi Citation2012), porcine (Wu et al. Citation2001), caprine (Magalhães et al. Citation2011), ovine (Barboni et al. Citation2011; Luz et al. Citation2012) and monkey (Nakamura et al. Citation2016; Sakai et al. Citation2020; Gyobu-Motani et al. Citation2023). Furthermore, research on xenogeneic ovarian follicle formation between different species is also being conducted in avians (Liu et al. Citation2007).
In humans, as the number of infertility treatment patients has increased rapidly, research on in vitro egg production is necessary to overcome the era of low birth rate and treat infertile patients (Kee et al. Citation2006; Chen et al. Citation2007; Park et al. Citation2009; West et al. Citation2010; Leng et al. Citation2015; Jung et al. Citation2017; Yang et al. Citation2019). However, humans are limited in the number of eggs they can produce in a lifetime, and obtaining many eggs from a woman carries many risks. There are ethical issues with using human-derived germ cells, including harm to human life in the embryonic state. Thus, using germ cells derived from human embryos to treat human infertility and study maternally inherited diseases is challenging. Therefore, research has been actively conducted to generate human oocytes from xenogeneic ovaries to solve the above problems. Recently, research has been actively conducted to generate human oocytes from xenogeneic ovaries. The xenogeneic formation of ovarian follicles was performed by mixing human primordial germ cells with mouse germline somatic cells (Yamashiro et al. Citation2018a). However, due to the lack of research on in vitro folliculogenesis, only oogonia formation has been successful. There have been no reports on generating mammalian xenogeneic follicles in vitro.
Regarding similarity to humans, the evolutionary differences between mice, rats, and humans are minimal, but in some respects, rats are closer to humans than mice. For example, both rat and human genomes encode a similar number of genes. (Gibbs and Pachter Citation2004). Moreover, rats are physiologically and genetically closer to humans than mice (Do Carmo and Cuello Citation2013). These make them ideal models for biomedical and clinical research (Jacob Citation2010; Baker et al. Citation2013; Smith et al. Citation2019). Hence, rat models are widely used in toxicology, teratology, endocrinology, oncology, neurology, experimental geriatrics, cardiovascular research, dental research, and experimental parasitology. Therefore, in vitro xenogeneic ovary formation using rats will be utilized in basic research for human oocyte reproductive bioengineering by understanding the differences in culture medium between species and investigating mechanisms related to in vitro rat ovary formation and folliculogenesis. To date, most studies on rat oocyte production have been conducted only for the IVM process using in vivo oocytes (Vanderhyden and Armstrong Citation1990; Jiao et al. Citation2016; Taketsuru and Kaneko Citation2016) and the method of forming in vitro ovaries from rat gonads is not properly optimized.
This study demonstrated a system for the formation of in vitro rat ovaries from gonads. First, the culture conditions for follicle formation from rat gonads were optimized, and the number of follicles formed and the ovarian size were compared according to the culture medium. In vitro oocytes generated under optimized culture conditions can mature into MII oocytes. Finally, based on the above experiment, we established an optimal protocol for in vitro xenogeneic follicle formation between mouse and rat species and generated oocytes.
Materials and methods
Reagents
The following reagents and chemicals were purchased from respective suppliers: Dulbecco’s modified Eagle’s medium (1×) (DMEM; Gibco, cat. no. 11965-092), phosphate-buffered saline (PBS; Gibco, cat. no. 10010-023), Minimum Essential Medium Eagle, alpha modification (1×) (α-MEM; Gibco, cat. no. 12571–063 and 32571-036), 2-mercaptoethanol (55 mM; Gibco, cat. no. 21985-023), fetal bovine serum (FBS; Gibco, cat. no. 16000-044), GMEM (1×) (Thermo, cat. no. 11710-035), KSR (15%) (Thermo, cat. no. 10828-028), retinoic acid (100 mM; Sigma, cat. no. R2625), GlutaMax (100×) (Thermo, cat. no. 32571-036), non-essential amino acids (100×) (Thermo, cat. no. 11140-050), sodium pyruvate (Gibco, cat. no. 11360-070), Y27632 (Peprotech, cat. no. 1293823), penicillin/streptomycin/L-glutamine (100×) (Gibco, cat. no. 10378-016), Insulin-Transferrin-Selenium (ITS; Thermo, cat. no. 41400-045), ascorbic acid (150 mM; TCI, cat. no. G0394), ICI182,780 (Tocris, cat. no. 1047), follicle-stimulating hormone (FSH; Sigma, cat. no. F4021), epidermal growth factor (EGF; R&D Systems, cat. no. 2028-EG-200), polyvinylpyrrolidone (PVP; Sigma, cat. no. PVP360), human chorionic gonadotropin (1,200 IU mL−1) (hCG; Sigma, cat. no. C8554), collagenase type IV (1 g mL−1; Gibco, cat. no. 17104-019), trypsin-EDTA, 0.25% (Gibco, cat. no. 25200-072), TCM 199(Gibco, cat. no. 11150-059), StemPro-34 SFM (1×), liquid (Gibco, cat. no. 10639-011), and hyaluronidase (500 µg ml−1, TCI, cat. no. H0164), anti-Ddx4 (polyclonal, 1:500; Abcam), anti-GDF9 (polyclonal, 1:500; Invitrogen), anti-α-tubulin (monoclonal, 1:20, Thermo, cat no. 53-4502-82) fluorescently labeled (Alexa Fluor 488 or 568; Molecular Probes, Eugene, OR, USA), DAPI (molecular probes, Thermo).
Animal and ethics approval
All animal procedures were approved by the Animal Ethics Committee of Jeonbuk National University (JBNU, NON2023-137), Republic of Korea. All experiments and procedures were performed in accordance with the ethical standards, guidelines, and regulations of Jeonbuk National University. Pregnant female Sprague–Dawley rats and pregnant female outbred albino ICR mice were purchased from Samtako (Republic of Korea). Rats and mice were sacrificed by CO2 gas chamber to obtain E12.5, E13.5, E14.5, and E15.5 embryos, and gonads were isolated from female viviparous for ovary formation.
Magnetic-activating cell sorting (MACS)
The SSEA1-positive mPGCs were obtained using MACS according to the manufacturer's instructions. Briefly, 0.25% trypsin-EDTA was used to prepare a single suspension of 13.5dpc mouse gonads. Single cells were incubated with anti-SSEA1 antibody conjugated with microbeads (Miltenyi Biotech) at a 1:4 dilution for 15 min on ice. After washing with MACS buffer (PBS supplemented with 0.5% BSA and 2 mM EDTA) followed by centrifugation 350xg for 10 min, SSEA1-positive mPGCs were purified using an MS column (Miltenyi Biotech). For a collection of rat gonadal somatic cells, c-kit-positive cells were depleted. As mentioned above, a single suspension of 15.5dpc rat gonads was incubated with anti-rabbit c-kit antibody (Bioss) at 1:500 dilution for 15 min on ice, washed with MACS buffer and centrifuged at 350xg for 10 min. The washed cells were incubated with anti-rabbit antibody-conjugated microbeads at a 1:4 dilution for 15 min on ice. After washing with MACS buffer followed by centrifugation 350xg for 10 min, c-kit-positive rPGCs were depleted. Cells in the flow-through were used as a c-kit-depleted cell population.
FACS analysis
The 15.5dpc rat gonads were dissociated into single cells by treatment with 0.25% trypsin-EDTA. The cell pellets were then resuspended in PBS supplemented with 1% BSA and stained with anti-rabbit c-kit-FITC antibody (1:100, Bioss) for 30 min on ice. After antibody incubation, the cells were washed twice with cold PBS + 1% BSA and analyzed using FACS Symphony A3 (Becton Dickinson) installed at the Center for University Research Facility (CURF) at Jeonbuk National University.
Quantitative real-time PCR (qRT-PCR)
RNA was extracted from the cells using the AccuPrep® Universal RNA Extraction kit (Bioneer, Seoul, Korea) following the manufacturer's protocol. 1μg of total RNA was reverse-transcribed using Accupower® CycleScript RT Premix (Bioneer, Seoul, Korea) according to the manufacturer's instructions. Relative gene expression was measured in triplicated using Powerup SYBR Green master mix (Applied Biosystems, CA, USA). Relative changes in gene expression were analyzed using the 2−ΔΔCt method, and the results were normalized against the expression level of the housekeeping gene. The experiment was independently replicated three times. The following primers were used: rat GapDH sense, 5' – TCACAATTCCATCCCAGACCCCA – 3’; rat GapDH antisense, 5’ – ATGGTATTCGAGAGAAGGGAGGGC – 3’; rat Nr5a1 sense, 5’ – TCCTGGTATCTGAGGTCCCA – 3’; rat Nr5a1 antisense, 5’ – GGATGACCTCTGACCCAACA – 3’; rat Gata4 sense, 5’ – GATCTGACTCCAAAGCGCTG – 3’; rat Gata4 antisense, 5’ – TGATGGGTGATGTGCCTTCT – 3’; rat Lhx9 sense, 5’ – TGCTCCACTTGCAACAAGAC – 3’; rat Lhx9 antisense, 5’ – GGCTGTCCTTCATACCGAAA – 3’; mouse GapDH sense, 5’ – ATGAATACGGCTACAGCAACAGG – 3’; mouse GapDH antisense, 5’ – CTCTTGCTCAGTGTCCTTGCTG – 3’; mouse Blimp1 sense, 5’ – AGCATGACCTGACATTGACACC – 3’; mouse Blimp1 antisense, 5’ – CTCAACACTCTCATGTAAGAGGC – 3’; mouse Prdm14 sense, 5’ – ACAGCCAAGCAATTTGCACTAC – 3’; mouse Prdm14 antisense, 5’ – TTACCTGGCATTTTCATTGCTC – 3’.
Making xenogeneic reconstituted ovaries (XrOvaries)
Mouse PGCs and rat gonadal somatic cells obtained by MACS were resuspended in an appropriate volume of GK15 + RA medium (GMEM supplemented with 15% KSR, 100× NEAA, 100× GlutaMax, 25 µg ml−1 sodium pyruvate, 1 µM retinoic acid and 10 µM Y27632). Next, 5,000 mPGCs were aggregated with 50,000 rat gonadal somatic cells in a low-binding U-bottom 96well plate in GK15 + RA medium. Aggregation was performed for 2 days at 37°C under 5% CO2 and 95% air.
In vitro differentiation (IVDi) culture
Female fetal gonads without mesonephros were cultured in Transwell-COL membranes (3.0-µm pore size, 24-mm diameter, Corning, Inc.) soaked in α-MEM-based IVDi medium (α-MEM supplemented with 2% FBS, 150 µM ascorbic acid, 1× penicillin/streptomycin/L-glutamine, and 55 µM 2-mercaptoethanol). From days 5–11 of culture, 10 µM ICI182,780 was added to α-MEM-based IVDi medium (hereafter referred to as α-MEM). On day 4 of culture, the culture medium was changed to StemPro-based IVDi medium (StemPro34 SFM supplemented with 10% FBS, 150 µM ascorbic acid, 1× penicillin/streptomycin/L-glutamine, and 55 µM 2-mercaptoethanol). From days 7–10 of culture, 500 nM ICI182,780 was added to StemPro34-based IVDi medium (hereafter referred to as StemPro34). On day 29 of culture, individual secondary follicles (2FLs) were manually dissociated using sharpened tungsten needles. Gonads were cultured for 29 days at 37°C under 5% CO2 and 95% air. Approximately half of the medium in each well was replaced with fresh medium every other day.
Isolation of secondary follicles (2FLs)
Electrically sharpened tungsten needles were used to isolate individual 2FLs. The interstitial cells between the 2FLs were carefully removed. Then, 2FLs were separated from the ovaries and placed at regular intervals.
In vitro growth (IVG) culture
Single 2FLs on Transwell-COL membranes were soaked in IVG-ITS medium (α-MEM supplemented with 3 mg ml−1 BSA, 5 µg ml−1 insulin, 5 µg ml−1 transferrin, 5 ng ml−1 selenium, and 0.1 IU ml−1 FSH) for 10 days. Approximately half of the medium in each well was replaced with fresh medium every other day.
In vitro maturation (IVM) culture
Cumulus-oocyte complexes were transferred to IVM medium (TCM199 supplemented with 10% FBS, 0.1 IU ml−1 FSH, 5 ng ml−1 EGF, 1.5 IU ml−1 hCG, and LH). After 16 h of culture, swollen cumulus cells were stripped from oocytes by treatment with 1% hyaluronidase, and then MII oocytes were determined by the first polar body extrusion.
Immunofluorescence staining
In brief, oocytes were fixed for 40 min at room temperature in 4% paraformaldehyde. The cells were washed with PBS and treated with PBS containing 10% normal goat serum and 0.03% Triton X-100 for 45 min at room temperature. The primary antibodies used were anti-Ddx4, GDF9, and α-tubulin. Fluorescently labeled secondary antibodies were used to detect primary antibodies according to the manufacturer's specifications. All immunofluorescence samples were counterstained with DAPI, after which they were observed by a Leica DMi8 microscope and confocal laser-scanning microscope (LSM 880 with Airyscan, Carl Zeiss, Germany) installed at the Center for University-wide Research Facilities (CURF) at Jeonbuk National University.
Simple sequence length polymorphism (SSLP) Analysis
The rat-specific satellite DNA D1Rat122 and the mouse-specific satellite DNA D7Mit44 were used as species-specific DNA sequences for SSLP analysis of XrOocytes. The SSLP experiment was performed according to a standard procedure (Li et al. Citation2014). Primers are shown as follows (5’ to 3’): D1Rat122-F: TCATTTTCCTTCCTCTCTCTCAGTATG; D1Rat122-R: AAGACAAAATGCTGGAGGGAGTAA; D7Mit44-F: GTGAAACCATGGTGCAGATGACT; D7Mit44-R: TTCTGGCCTCTGTGAAGTAGTGAAC.
Statistical analysis
All experiments were performed in triplicate, and the generation of miniaturized ovaries using each method; the mean number of intact follicles per ovary was calculated by an arithmetical calculation. The data was collated and expressed as means ± standard error (SE) of the mean. Statistical tests were conducted using SAS version 9.4 (SAS Institute Inc., Cary, NC, USA), and statistical differences were performed with Student's t-test. A p-value < 0.05 (* p < 0.05, ** p < 0.01, and *** p < 0.001 in Figure) was regarded as significant.
Results
Generation of ovarian follicles using female rat gonads
To examine whether in vitro formation of ovarian follicles was induced from rat gonads, we isolated the gonads without mesonephros from E15.5 female rats (A). The rat gonads were cultured with α-MEM supplemented with 10% (vol/vol) FBS on transwell previously reported as mouse gonad culture (Morohaku et al. Citation2016). After 4 weeks, we identified ovary-like tissues (in vitro ovaries) containing follicles in rats as well as mice (B, white arrow). However, the efficiency of ovarian follicle formation was lower than that of mice. In addition, ovarian follicle formation was barely observed in α-MEM medium. The ovarian follicles located in the center of the ovary-like tissues were poorly formed. Therefore, in order to supply nutrients and signals to the inner follicles, the gonads were manually divided into several parts and cultured as previously described (C) (Jang and Choi Citation2021). As a result, the ovarian formation efficiency was somewhat improved, but as the culture progressed, the follicle formation rate was low, as in the previous results (D and E). Taken together, the partial culture of gonads helped to form in vitro ovaries efficiently. Still, it could not increase the efficiency of follicle formation in that ovaries, and α-MEM medium was not suitable for ovarian formation.
Figure 1. Optimized of follicular assembly in cultured rat gonads. (A) Gonads derived from rat embryos at 15.5dpc (down). (Scale bar, 500 μm). (B) Representative images of mouse and rat ovaries at 1∼4weeks of culture in α-MEM medium. (Scale bars 200 μm). (C) Image of the ovaries from gonadal tissues dissociated by three parts mechanical cutting. (Scale bar 500 μm). (D) Representative images of ovaries from three parts gonadal tissues at 1∼4weeks of culture in α – MEM medium. (Scale bars 200 μm). (E) Number of follicles generated from α-MEM medium at 4weeks. (n = 33, Experiments were performed in triplicate)
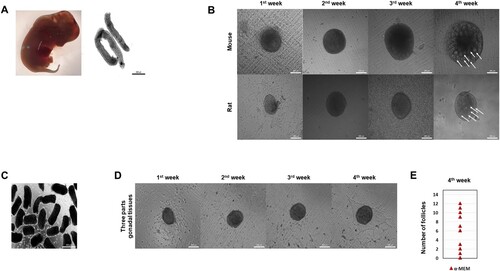
Optimized culture conditions for the formation of rat follicles
Next, to optimize the efficiency of ovarian follicle formation, we compared the two culture media by measuring the size, number, and morphological changes of the formed follicles. As a result, compared to the previously used α-MEM complete medium (α-MEM), it was confirmed that follicle development was much better induced with StemPro34-based IVDi medium (StemPro34) (Hikabe et al. Citation2016). Interestingly, the difference in follicle morphology in the cultured ovaries of each group showed a significant change from 3rd week (A). Then, we compared the differences in the number of ovarian follicles. The follicles formed from α-MEM did not differ in size, and an average of 2 follicles were formed, whereas the follicles cultured from StemPro34 dramatically increased in size and formed an average of 20 follicles (B). Moreover, the size of the ovaries cultured in α-MEM in the 4th week showed a significant difference for each ovary, whereas the ovaries cultured in StemPro34 showed a uniform size (C). Our results show that optimized culture condition gonad-derived ovaries can induce well-developed ovary-like tissues, including ovarian follicles.
Figure 2. Evaluation of the culture basal medium conditions. (A) Representative images of ovaries at 1∼4weeks of culture in the medium indicated on the left are shown. (Scale bars 200 μm) (B) Number of follicles in ovaries generated from different basal medium. (n = 33, Experiments were performed in triplicate) (* p < 0.05, ** p < 0.01, *** p < 0.001) (C) Ovary growth in different basal medium on 1∼4weeks culture. (The box plot shows the diameters of ovary on the 1∼4weeks) (* p < 0.05, ** p < 0.01, *** p < 0.001)
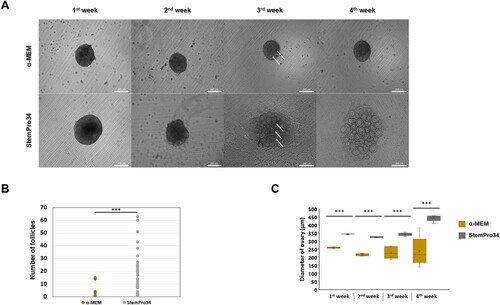
Maturation of rat oocytes in vitro
After 4 weeks of ovarian development, physical isolation of follicles was performed on mature ovarian follicles, including oocytes, before entering the in vitro growth (IVG) of oocytes. Isolation is a necessary process that resolves that the proliferation of the granulosa cell layer is limited in secondary follicle-like structures located at the border and internal of the in vitro ovary (Hikabe et al. Citation2016). The follicles isolated from the in vitro-developed ovaries formed cumulus-oocyte complexes and proliferated granulosa cells. Hence, there was little loss or damage to the oocytes during the isolation process, and eventually, oocytes could be obtained in large quantities (A). After 10 days of IVG culture with granulosa proliferation, clearly outlined germinal vesicles (GVs) with a characteristic nucleolus of oocytes were observed in most of the follicular structures (B). To confirm that the generated oocytes were indeed oocytes, the Ddx4 gene expressed in the cytoplasm of the oocytes and the oocyte-specific gene, GDF9, were immunostained (Noce et al. Citation2001; Pisarska et al. Citation2011; Sabour et al. Citation2011). The in vitro oocytes expressed Ddx4, as well as GDF9 (C). These data demonstrated that the culture of StemPro34 is applicable to the generation of normal oocytes from the gonads of rats as well as mice. GV oocytes were cultured with in vitro maturation (IVM) medium containing epidermal growth factor (EGF), follicle-stimulating hormone (FSH), and human chorionic gonadotropin (hCG) for 16 h at 37°C. This medium induced to resume meiosis. After 16 h, the oocytes were released from the first polar body and became MII oocytes. MII oocytes showed well-aligned chromosomes and typical barrel-shaped spindles. (D and E). However, unlike in mice, rat follicles showed a lower maturation efficiency of 0.9% (S1 Table).
Figure 3. Isolation of individual rat follicles for IVG culture and MII oocyte from IVM culture. (A) Representative images of isolation of individual follicles. (Scale bars 50 μm) (B) Representative follicle cultured on a Transwell-COL membrane on days 30–39 of culture. (Scale bars 200 μm) (C) Oocyte phase and expression of the Ddx4 and oocyte-specific marker GDF9 in vitro. (Scale bars 50 μm) (D) Image of the first polar body in MII oocyte. (Scale bar 50 μm) (E) Expression of α – tubulin in MII oocyte in vitro. (Scale bars 50 μm)
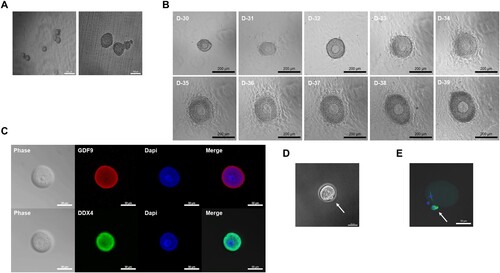
Reconstitution of xenogeneic ovaries between mouse and rat in vitro
The culture period was divided into two parts in this study to reconstruct the in vitro oocyte development process. The first is the process of aggregation of mouse gonadal primordial germ cells (PGCs) and rat gonadal somatic cells for two days, and the second is the in vitro differentiation (IVDi) process for four weeks. We isolated rat somatic cells that were negative for c-kit antibody, which is widely used to isolate PGCs from rats, and we used a specific surface embryonic antigen 1 (SSEA1) antibody to isolate PGCs from mouse gonads (A and S1 Fig) (Oikawa et al. Citation2022). We analyzed through quantitative real-time PCR (qRT-PCR) to confirm that somatic cells were well isolated from rat gonads. The somatic cells highly expressed the somatic cell markers LHX9, Gata4, and Nr5a1 (S2 Fig). The aggregation was performed for two days on U-bottom 96well with E13.5 female mouse gonadal primordial germ cells from the albino ICR strain with E15.5 female rat gonadal somatic cells from the albino Sprague Dawley (SD) strain (B). In in vitro differentiation (IVDi) of the aggregates, after this called xenogeneic reconstituted ovaries (XrOvaries), were placed on Transwell-COL, a culture insert coated with collagen, and filled with α-MEM medium. After 4 days of culture, the medium was replaced with StemPro34 medium. XrOvaries were confirmed at the 2nd week to form follicular structures containing one oocyte each. After that, progressively developing follicles could be observed every week, and well-developed XrSecondary follicles and oocytes were observed at 4 weeks (B, C and D). In this approach, Simple Sequence Length Polymorphism (SSLP) experiments are traditionally performed by analyzing PCR amplified SSLP markers between recipient and donor strains (Gurumurthy et al. Citation2015). To confirm whether oocytes were derived from mouse PGCs and cumulus cells were derived from rat gonadal somatic cells, we performed SSLP experiments. We confirmed the presence of parental species-specific DNA sequences in the generated oocytes and cumulus cells surrounding the oocyte (D). We detected mouse species-specific DNA sequence (D7Mit44) from oocytes and confirmed the rat species-specific DNA sequence (D1Rat122) from cumulus cells. Unexpectedly, we also detected D7Mit44 bands in the cumulus cells. Collectively, these data demonstrated that the co-culture of mouse PGCs and rat somatic cells successfully formed XrOvaries and generated XrFollicles and mouse oocytes in vitro. Finally, the XrFollicles generated using the aforementioned method were subjected to IVG for 10 days and then IVM for 16 hours, but unfortunately, no MII oocytes were produced (S2 Table).
Figure 4. The XrOocyte production from xenogeneic ovary. (A) A schematic of XrOocyte production in vitro. (B) Representative images of XrOvaries at 1∼4weeks of culture in StemPro34 medium. (Scale bars 200 μm). (C) Representative images of isolation of individual XrFollicles. (Scale bars 200 μm). (D) Representative images of isolation of individual XrOocytes. (Scale bars 200 μm). (E) The SSLP analysis of XrOocyte lines #1∼#6 and cumulus cell lines #1∼#2 is shown.
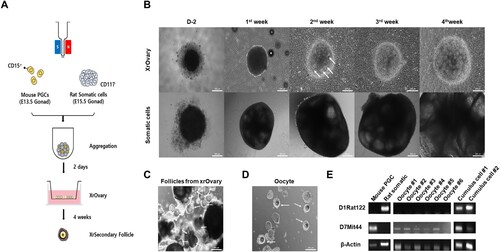
Discussion
The folliculogenesis and oogenesis are essential steps to overcome the socially emerging infertility problem. One of the major diseases that causes female infertility, which affects about 1% of women aged 30–40 years old, is primary ovarian insufficiency (POI) or premature ovarian insufficiency (POF) (Sadeghi Citation2013; Torrealday et al. Citation2017). In the reality that there are few effective methods of treating women with POI, hormone replacement therapy (a combination of estrogen, progestin, and gonadotropin) is the only standard treatment that replaces premenopausal levels of ovarian sex steroids (Luisi et al. Citation2013; Park et al. Citation2015; Kim et al. Citation2022; Ha et al. Citation2023). However, a fundamental way to overcome infertility is to uncover the mechanism of in vitro mammalian folliculogenesis and generate large numbers of in vitro oocytes.
Since the late twentieth century, advancements have been made in ovarian follicle culture. Up to now, various culture systems have been established for different follicular stages across multiple species, as reported by Hartshorne (Hartshorne Citation1997) and Simon's colleagues (Simon et al. Citation2020). Additionally, there's been progress in developing culture systems enabling the maturation of porcine primordial and primary follicles into meiotic oocytes capable of fertilization (Telfer et al. Citation2000; Kaneko et al. Citation2003). Later, in 2016, E12.5 female mouse gonadal somatic cells co-culture system with Primordial germ cell-like cells (PGCLCs) derived from mouse embryonic pluripotent stem cells (mESCs) was established by Hayashi and colleagues (Hikabe et al. Citation2016). Based on this, Hayashi and colleagues optimized the differentiation of fetal ovarian somatic cell-like cells (FOSLCs) and PGCLCs derived from mESCs and succeeded in follicle development and oocyte growth through co-culture (Yoshino et al. Citation2021). Recently, Ji Wu and colleagues reported that they generated offspring using Matrigel (3D ovarian organoids) through co-culture of mouse female germline stem cells (FGSCs) and mouse female gonadal somatic cells (Li et al. Citation2021). The generation and development of in vitro oocyte research has been conducted in mice and various species, but a protocol for generating oocytes by forming in vitro ovaries from rat gonads has not been established yet. In addition, there is no progress at the oogonia stage in human oocyte generation using xenogeneic ovaries in vitro. In this study, we established an optimal system for ovarian formation and oocyte production from rat gonads in vitro. And further succeeded in xenogeneic ovarian formation and oocyte generation from mouse and rat in vitro.
In mice, PGC becomes identifiable as a cluster of approximately 40 cells at the base of the early allantois at 7.25 days around the embryonic day (Chiquoine Citation1954; Ginsburg et al. Citation1990). They migrate to the hindgut endoderm, developing at ∼E7.75, move into the mesentery at ∼E9.5, and colonize the genital ridge at ∼E10.5 (Tam and Snow Citation1981; Molyneaux et al. Citation2001). Then, PGCs proliferated until ∼E13.5 (Hilscher et al. Citation1974; Speed Citation1982; Saitou and Yamaji Citation2012). However, in rats, PGCs were identified at the visceral yolk sac endoderm and base of the allantois at E10.5. They migrate to the ventral and lateral gut walls at E11.5, into the mesenchyme at ∼E12.5, and colonized the genital ridge at ∼E14 (Kemper and Peters Citation1987; Bandyopadhyay et al. Citation2004). According to previous reports, migration of rat PGC is later than that of mice in vivo. Therefore, mice mainly use 13.5dpc gonads, and rats mainly use 15.5dpc gonads.
In mice, studies of follicle and oocyte production from gonads or PGCs with germline somatic cell aggregation have mainly used α-MEM and StemPro34 (Ishikura et al. Citation2021; Ishikura et al. Citation2022; Aizawa et al. Citation2023; Murakami et al. Citation2023). We confirmed whether it could be applied to in vitro ovary formation from gonads in rats as well as mice. When gonads were cultured in α-MEM, in vitro ovaries were formed, and they generated secondary follicles. However, the α-MEM showed low follicle formation efficiency. We assumed that the fundamental reason for this low follicle generation efficiency was developmental difficulties in oocytes due to a lack of signal, nutrient factors, and space for cell growth in the center (Hikabe et al. Citation2016). To solve this problem, we tried a method that could produce fewer follicles in several ovaries by chopping the gonads, which showed efficiency in a previous study (Jang and Choi Citation2021). Still, it was ineffective regarding follicle development and oocyte generation efficiency.
Nevertheless, the follicle's inner part of the ovary developed well. Similar to the follicle's outer part of the ovary, signals and nutrients seemed to be well transferred to the inside of the ovary. However, it was found that 29 of 33 ovaries did not form follicles, except for 4 ovaries (12% ovary and follicle formation efficiency). These data showed that α-MEM can form ovaries from rat gonads, but it is unsuitable for obtaining many oocytes.
The efficiency of follicle formation and ovarian size changes according to α-MEM and StemPro34 were compared weekly. Most ovaries in the α-MEM medium did not produce follicles, but all of the ovaries in the StemPro34 medium produced follicles in the second week. The difference was noticeable in the fourth week; the ovarian size and number of follicles in α-MEM were still not significantly different, but StemPro34 showed a sharp increase. This is because the follicles did not develop well in α-MEM, so there was no change in the size of the ovary either. Collectively, these results indicated that similar to mice, StemPro34 is much more effective and suitable for in vitro ovary formation from rat gonads than α-MEM. Therefore, we used StemPro34 medium to perform all further experiments.
The oocytes made in this way expressed Ddx4 (germ cell marker) and GDF9 (oocyte-specific marker). It functions as an RNA binding protein (Linder and Lasko Citation2006), which was revealed by immunochemical staining with Ddx4 antibody in permeable, fully grown oocytes of the antral follicles to reveal cytoplasmic distribution (Zarate-Garcia et al. Citation2016; Gassei et al. Citation2017). GDF9 is also known to be indispensable for the oocyte-dependent development of ovarian follicles beyond the primary follicular stage (Dong et al. Citation1996). The maturation of the oocyte showed MII oocyte characteristics in the first polar body and the oocyte. Meiotic maturation has two critical processes: spindle assembly and chromosome migration, and errors in these processes can result in abnormal oocytes, such as aneuploid eggs (Chiang et al. Citation2012). These data showed that StemPro34 culture medium was able to generate oocytes from in vitro rat gonads.
Saitou and colleagues reported that they differentiated the human PGCLCs only into oogonia-like cells for four months in xenogeneic reconstituted ovaries with mouse embryonic ovarian somatic cells in vitro culture (Yamashiro et al. Citation2018b). Oocyte growth relies on interactions mediated by gap junctions that metabolically link two cell types (Eppig Citation1979; Herlands and Schultz Citation1984) with companion somatic cells and paracrine factors, such as KIT ligand (KL) (Packer et al. Citation1994) and other uncharacterized molecules (Cecconi et al. Citation1996). It seems that development after oogonia is complex due to differences in the egg development period and signal interactions between human PGCLCs and mouse somatic cells. In contrast, based on the established protocol above, we found that the culture medium and period of in vitro ovarian culture in rats was similar to that in mouse. Therefore, we successfully generated oocytes by aggregating mouse PGCLCs with rat somatic cells to generate xenogeneic ovaries in vitro. The oocytes were confirmed to be mouse-derived through SSLP, and the cumulus cells were confirmed to be rat – and mouse-derived. These results suggested that the DNA of mouse PGCs, which did not develop into an oocyte. Taken together, this system means that mouse-derived oocytes can be generated by rat cumulus cells. Nevertheless, under previously known mouse IVM culture conditions or rat IVM culture conditions, xenogeneic oocytes did not develop into M2 oocytes. This means that xenogeneic maturation culture requires specific culture conditions. Therefore, further research is required to explore the culture conditions and environment for xenogeneic oocyte in the IVM process.
In conclusion, xenogeneic ovarian and oogenesis research is making significant advances in life sciences and medicine and has the potential to be applied in a variety of fields. Our in vitro xenogeneic follicle formation system has established an optimized culture system to generate oocytes from mice and rats, which allows visualization of all processes in vitro. This method will be helpful in developing xenogeneic ovarian models applicable to other species and be used as a basis for mechanistic studies, such as developing germ cells, disease models, and new treatments. This culture condition would also help solve the infertility problem in humans and may contribute to preserving endangered mammalian species for future generations. However, due to the low efficiency of MII oocyte production, further research is needed to investigate the mechanisms and optimal conditions to enhance the production efficiency of MII oocytes. Moreover, additional research is necessary to determine whether the generated maturated oocytes can be functionally modified to produce offspring for the next generation.
Supplemental Material
Download Zip (206.7 KB)Disclosure statement
No potential conflict of interest was reported by the author(s).
Additional information
Funding
References
- Aizawa E, Ozonov EA, Kawamura YK, Dumeau CE, Nagaoka S, Kitajima TS, Saitou M, Peters AH, Wutz A. 2023. Epigenetic regulation limits competence of pluripotent stem cell-derived oocytes. EMBO J. 42:e113955.
- Baker HJ, Lindsey JR, Wesibroth SH. 2013. The laboratory rat: biology and diseases. Vol. 1984. Elsevier; p. 91–122. doi:10.1016/B978-0-12-263620-2.50010-0.
- Bandyopadhyay S, Banerjee S, Pal AK, Goswami SK, Chakravarty B, Kabir SN. 2004. Primordial germ cell migration in the Rat: preliminary evidence for a role of Galactosyltransferase1. Biol Reprod. Dec. 71:1822–1827. Epub 2004/08/03. doi:10.1095/biolreprod.104.028555.
- Barboni B, Russo V, Cecconi S, Curini V, Colosimo A, Garofalo MLA, Capacchietti G, Di Giacinto O, Mattioli M. 2011. In vitro grown sheep preantral follicles yield oocytes with normal nuclear-epigenetic maturation. PLoS One. 6:e27550.
- Cecconi S, Rossi G, De Felici M, Colonna RJMR, Research DIG. 1996. Mammalian oocyte growth in vitro is stimulated by soluble factor (s) produced by preantral granulosa cells and by Sertoli cells. Molecular Reproduction and Development: Incorporating Gamete Research. 44:540–546.
- Chen HF, Kuo HC, Chien CL, Shun CT, Yao YL, Ip PL, Chuang CY, Wang CC, Yang YS, Ho HN. 2007. Derivation, characterization and differentiation of human embryonic stem cells: comparing serum-containing versus serum-free media and evidence of germ cell differentiation. Hum Reprod. 22:567–577.
- Chiang T, Schultz RM, Lampson MA. 2012. Meiotic origins of maternal age-related aneuploidy. Biol Reprod. 86(3):1–7.
- Chiquoine AD. 1954. The identification, origin, and migration of the primordial germ cells in the mouse embryo. Anat Rec. 118:135–146.
- Do Carmo S, Cuello AC. 2013. Modeling Alzheimer's disease in transgenic rats. Mol Neurodegener. 8:37. Epub 2013/10/29. doi:10.1186/1750-1326-8-37.
- Dong J, Albertini DF, Nishimori K, Kumar TR, Lu N, Matzuk MM. 1996. Growth differentiation factor-9 is required during early ovarian folliculogenesis. Nature. 383:531–535. Epub 1996/10/10. doi:10.1038/383531a0.
- Eppig JJ. 1979. A comparison between oocyte growth in coculture with granulosa cells and oocytes with granulosa cell-oocyte junctional contact maintained in vitro. J Exp Zool. 209:345–353.
- Gassei K, Sheng Y, Fayomi A, Mital P, Sukhwani M, Lin C-C, Peters KA, Althouse A, Valli H, Orwig KE. 2017. DDX4-EGFP transgenic rat model for the study of germline development and spermatogenesis. Biol Reprod. 96:707–719.
- Gibbs RA, Pachter LJ. 2004. Genome sequence of the Brown Norway rat yields insights into mammalian evolution. Nature. 428:493–521.
- Ginsburg M, Snow MH, McLaren A. 1990. Primordial germ cells in the mouse embryo during gastrulation. Development. 110:521–528.
- Gupta P, Nandi S. 2012. Isolation and culture of preantral follicles for retrieving oocytes for the embryo production: Present status in domestic animals. Reprod Domest Anim. 47:513–519.
- Gupta P, Ramesh H, Manjunatha B, Nandi S, Ravindra J. 2008. Production of buffalo embryos using oocytes from in vitro grown preantral follicles. Zygote. 16:57–63.
- Gurumurthy CB, Joshi PS, Kurz SG, Ohtsuka M, Quadros RM, Harms DW, Lloyd KCK. 2015. Validation of simple sequence length polymorphism regions of commonly used mouse strains for marker assisted speed congenics screening. Int J Genomics. 2015:735845.
- Gyobu-Motani S, Yabuta Y, Mizuta K, Katou Y, Okamoto I, Kawasaki M, Kitamura A, Tsukiyama T, Iwatani C, Tsuchiya H, et al. 2023. Induction of fetal meiotic oocytes from embryonic stem cells in cynomolgus monkeys. EMBO J. 42:e112962.
- Ha J, Shin J, Seok E, Kim S, Sun S, Yang H. 2023. Estradiol and progesterone regulate NUCB2/nesfatin-1 expression and function in GH3 pituitary cells and THESC endometrial cells. Animal Cells Syst (Seoul). 27:129–137. doi:10.1080/19768354.2023.2226735.
- Hartshorne GM. 1997. In vitro culture of ovarian follicles. Rev Reprod. 2:94–104.
- Hayashi K, Hikabe O, Obata Y, Hirao Y. 2017. Reconstitution of mouse oogenesis in a dish from pluripotent stem cells. Nat Protoc. 12:1733–1744. Epub 2017/08/11. doi:10.1038/nprot.2017.070.
- Hayashi K, Ogushi S, Kurimoto K, Shimamoto S, Ohta H, Saitou M. 2012. Offspring from oocytes derived from in vitro primordial germ cell-like cells in mice. Science. 338:971–975. Epub 2012/10/09.
- Hayashi K, Ohta H, Kurimoto K, Aramaki S, Saitou M. 2011. Reconstitution of the mouse germ cell specification pathway in culture by pluripotent stem cells. Cell. 146:519–532. Epub 2011/08/09. doi:10.1016/j.cell.2011.06.052.
- Herlands RL, Schultz RM. 1984. Regulation of mouse oocyte growth: probable nutritional role for intercellular communication between follicle cells and oocytes in oocyte growth. J Exp Zool. 229:317–325.
- Hikabe O, Hamazaki N, Nagamatsu G, Obata Y, Hirao Y, Hamada N, Shimamoto S, Imamura T, Nakashima K, Saitou M, et al. 2016. Reconstitution in vitro of the entire cycle of the mouse female germ line. Nature. 539:299–303. Epub 2016/11/04. doi:10.1038/nature20104.
- Hilscher B, Hilscher W, Bülthoff-Ohnolz B, Krämer U, Birke A, Pelzer H, Gauss G. 1974. Kinetics of gametogenesis: I. Comparative histological and autoradiographic studies of oocytes and transitional prospermatogonia during oogenesis and prespermatogenesis. Cell Tissue Res. 154:443–470.
- Hübner K, Fuhrmann G, Christenson LK, Kehler J, Reinbold R, De La Fuente R, Wood J, Strauss JF, Boiani M, Schöler HR. 2003. Derivation of oocytes from mouse embryonic stem cells. Science.. 300:1251–1256. Epub 2003/05/06.
- Ishikura Y, Ohta H, Nagano M, Saitou M. 2022. Optimized protocol to derive germline stem-cell-like cells from mouse pluripotent stem cells. STAR Protocols. 3:101544. Epub 2022/07/18.
- Ishikura Y, Ohta H, Sato T, Murase Y, Yabuta Y, Kojima Y, Yamashiro C, Nakamura T, Yamamoto T, Ogawa T, et al. 2021. In vitro reconstitution of the whole male germ-cell development from mouse pluripotent stem cells. Cell Stem Cell. 28:2167–2179. doi:10.1016/j.stem.2021.08.005.
- Jacob HJ. 2010. Methods in molecular biology. Methods in Molecular Biology (Clifton, NJ). 597:1–11. Epub 2009/12/17. doi:10.1007/978-1-60327-389-3_1.
- Jang SW, Choi HW. 2021. Generation of miniaturized ovaries by In VitroCulture from mouse gonads. Development & Reproduction. 25:173–183. Epub 2021/12/25. doi:10.12717/DR.2020.25.3.173.
- Jiao GZ, Cui W, Yang R, Lin J, Gong S, Lian HY, Sun MJ, Tan JH. 2016. Optimized protocols for In vitro maturation of Rat oocytes dramatically improve their developmental competence to a level similar to that of ovulated oocytes. Cell Reprogram. 18:17–29. Epub 2015/12/19. doi:10.1089/cell.2015.0055.
- Jung D, Xiong J, Ye M, Qin X, Li L, Cheng S, Luo M, Peng J, Dong J, Tang F, et al. 2017. In vitro differentiation of human embryonic stem cells into ovarian follicle-like cells. Nat Commun. 8:15680. doi:10.1038/ncomms15680.
- Kaneko H, Kikuchi K, Noguchi J, Hosoe M, Akita T. 2003. Maturation and fertilization of porcine oocytes from primordial follicles by a combination of xenografting and in vitro culture. Biol Reprod. 69:1488–1493.
- Kee K, Gonsalves JM, Clark AT, Pera RA. 2006. Bone morphogenetic proteins induce germ cell differentiation from human embryonic stem cells. Stem Cells Dev. 15:831–837. Epub 2007/01/27. doi:10.1089/scd.2006.15.831.
- Kemper CH, Peters PW. 1987. Migration and proliferation of primordial germ cells in the rat. Teratology. 36:117–124. Epub 1987/08/01. doi:10.1002/tera.1420360115.
- Kim K-d, Choe JM, Myoung S, Lee SH, Kim M, Choi J-H, Park HT. 2022. Estradiol treatment increases M2-like visceral adipose tissue macrophages in obese ovariectomized mice regardless of its anorectic action. Animal Cells Syst (Seoul). 26:243–253. doi:10.1080/19768354.2022.2128871.
- Kurimoto K, Yabuta Y, Hayashi K, Ohta H, Kiyonari H, Mitani T, Moritoki Y, Kohri K, Kimura H, Yamamoto T, et al. 2015. Quantitative dynamics of chromatin remodeling during germ cell specification from mouse embryonic stem cells. Cell Stem Cell. 16:517–532. doi:10.1016/j.stem.2015.03.002.
- Leng L, Tan Y, Gong F, Hu L, Ouyang Q, Zhao Y, Lu G, Lin G. 2015. Differentiation of primordial germ cells from induced pluripotent stem cells of primary ovarian insufficiency. Hum Reprod. 30:737–748.
- Li W, Li X, Li T, Jiang MG, Wan H, Luo GZ, Feng C, Cui X, Teng F, Yuan Y, et al. 2014. Genetic modification and screening in rat using haploid embryonic stem cells. Cell Stem Cell. 14:404–414. Epub 2013/12/24. doi:10.1016/j.stem.2013.11.016.
- Li X, Zheng M, Xu B, Li D, Shen Y, Nie Y, Ma L, Wu J. 2021. Generation of offspring-producing 3D ovarian organoids derived from female germline stem cells and their application in toxicological detection. Biomaterials. 279:121213. doi:10.1016/j.biomaterials.2021.121213.
- Linder P, Lasko P. 2006. Bent out of shape: RNA unwinding by the DEAD-box helicase Vasa. Cell. 125:219–221. Epub 2006/04/25. doi:10.1016/j.cell.2006.03.030.
- Liu CH, Chang IK, Sasse J, Dumatol CJ, Basker JV, Wernery U. 2007. Xenogenic oogenesis of chicken (Gallus domesticus) female primordial germ cells in germline chimeric quail (Coturnix japonica) ovary. Anim Reprod Sci. 101:344–350. doi:10.1016/j.anireprosci.2006.12.021.
- Luisi S, Orlandini C, Biliotti G, Scolaro V, De Felice G, Regini C, Petraglia F. 2013. Hormone replacement therapy in menopause and in premature ovarian insufficiency. Minerva Ginecologica. Dec. 65:607–620. Epub 2013/12/19.
- Luz V, Araujo V, Duarte A, Celestino J, Silva T, Magalhaes-Padilha D, Chaves R, Brito I, Almeida A, Campello C. 2012. Eight-cell parthenotes originated from in vitro grown sheep preantral follicles. Reprod Sci. 19:1219–1225.
- Magalhães D, Duarte A, Araújo V, Brito I, Soares T, Lima I, Lopes C, Campello C, Rodrigues A, Figueiredo J. 2011. In vitro production of a caprine embryo from a preantral follicle cultured in media supplemented with growth hormone. Theriogenology. 75:182–188.
- Molyneaux KA, Stallock J, Schaible K, Wylie C. 2001. Time-lapse analysis of living mouse germ cell migration. Dev Biol. 240:488–498.
- Morohaku K, Tanimoto R, Sasaki K, Kawahara-Miki R, Kono T, Hayashi K, Hirao Y, Obata Y. 2016. Complete in vitro generation of fertile oocytes from mouse primordial germ cells. Proc Natl Acad Sci U S A. 113:9021–9026. Epub 2016/07/28. doi:10.1073/pnas.1603817113.
- Murakami K, Hamazaki N, Hamada N, Nagamatsu G, Okamoto I, Ohta H, Nosaka Y, Ishikura Y, Kitajima TS, Semba Y, et al. 2023. Generation of functional oocytes from male mice in vitro. Nature. 615:900–906. doi:10.1038/s41586-023-05834-x.
- Nakaki F, Hayashi K, Ohta H, Kurimoto K, Yabuta Y, Saitou M. 2013. Induction of mouse germ-cell fate by transcription factors in vitro. Nature. 501:222–226. doi:10.1038/nature12417.
- Nakamura T, Okamoto I, Sasaki K, Yabuta Y, Iwatani C, Tsuchiya H, Seita Y, Nakamura S, Yamamoto T, Saitou M. 2016. A developmental coordinate of pluripotency among mice, monkeys and humans. Nature. 537:57–62.
- Noce T, Okamoto-Ito S, Tsunekawa N. 2001. Vasa homolog genes in mammalian germ cell development. Cell Struct Funct. 26:131–136.
- Oikawa M, Kobayashi H, Sanbo M, Mizuno N, Iwatsuki K, Takashima T, Yamauchi K, Yoshida F, Yamamoto T, Shinohara T, et al. 2022. Functional primordial germ cell–like cells from pluripotent stem cells in rats. Science. 376:176–179.
- Packer AI, Hsu YC, Besmer P, Bachvarova RF. 1994. The ligand of the c-kit receptor promotes oocyte growth. Dev Biol. 161:194–205.
- Park S-J, Kim T-S, Kim J-M, Chang K-T, Lee H-S, Lee D-S. 2015. Repeated superovulation via PMSG/hCG administration induces 2-Cys peroxiredoxins expression and overoxidation in the reproductive tracts of female mice. Mol Cells. 38:1071–1078. doi:10.14348/molcells.2015.0173.
- Park TS, Galic Z, Conway AE, Lindgren A, van Handel BJ, Magnusson M, Richter L, Teitell MA, Mikkola HK, Lowry WE, et al. 2009. Derivation of primordial germ cells from human embryonic and induced pluripotent stem cells is significantly improved by coculture with human fetal gonadal cells. Stem Cells. 27:783–795. Epub 2009/04/08.
- Pisarska MD, Barlow G, Kuo FT. 2011. Minireview: roles of the forkhead transcription factor FOXL2 in granulosa cell biology and pathology. Endocrinology. 152:1199–1208. Epub 2011/01/21. doi:10.1210/en.2010-1041.
- Sabour D, Araúzo-Bravo MJ, Hübner K, Ko K, Greber B, Gentile L, Stehling M, Schöler HR. 2011. Identification of genes specific to mouse primordial germ cells through dynamic global gene expression. Hum Mol Genet. 20:115–125. Epub 2010/10/14. doi:10.1093/hmg/ddq450.
- Sadeghi MR. 2013. New hopes for the treatment of primary ovarian insufficiency/premature ovarian failure. J Reprod Infertil. 1–2. Epub 2013/08/09.
- Saitou M, Yamaji M. 2012. Primordial germ cells in mice. Cold Spring Harbor Perspect Biol. 4. Epub 2012/11/06.
- Sakai Y, Nakamura T, Okamoto I, Gyobu-Motani S, Ohta H, Yabuta Y, Tsukiyama T, Iwatani C, Tsuchiya H, Ema M. 2020. Induction of the germ cell fate from pluripotent stem cells in cynomolgus monkeys. Biol Reprod. 102:620–638.
- Simon LE, Kumar TR, Duncan FE. 2020. In vitro ovarian follicle growth: a comprehensive analysis of key protocol variables. Biol Reprod. 103:455–470.
- Smith JR, Bolton ER, Dwinell MR. 2019. The Rat: A Model Used in Biomedical Research. Methods in Molecular Biology (Clifton, NJ). 2018:1–41. Epub 2019/06/23. doi:10.1007/978-1-4939-9581-3_1.
- Speed R. 1982. Meiosis in the foetal mouse ovary: I. An analysis at the light microscope level using surface-spreading. Chromosoma. 85(3):427–437.
- Taketsuru H, Kaneko T. 2016. In vitro maturation of immature rat oocytes under simple culture conditions and subsequent developmental ability. J Reprod Dev. 62:521–526. Epub 2016/07/02.
- Tam P, Snow M. 1981. Proliferation and migration of primordial germ cells during compensatory growth in mouse embryos. Development. 64:133–147.
- Telfer E, Binnie J, McCaffery F, Campbell B. 2000. In vitro development of oocytes from porcine and bovine primary follicles. Mol Cell Endocrinol. 163:117–123.
- Torrealday S, Kodaman P, Pal L. 2017. Premature Ovarian Insufficiency - an update on recent advances in understanding and management [version 1; peer review: 3 approved].6.
- Vanderhyden BC, Armstrong DT. 1990. Effects of gonadotropins and granulosa cell secretions on the maturation and fertilization of rat oocytes in vitro. Mol Reprod Dev. 26:337–346. Epub 1990/08/01. doi:10.1002/mrd.1080260408.
- West FD, Roche-Rios MI, Abraham S, Rao RR, Natrajan MS, Bacanamwo M, Stice SL. 2010. KIT ligand and bone morphogenetic protein signaling enhances human embryonic stem cell to germ-like cell differentiation. Hum Reprod. 25:168–178.
- Wu J, Emery BR, Carrell DT. 2001. In vitro growth, maturation, fertilization, and embryonic development of oocytes from porcine preantral follicles. Biol Reprod. 64:375–381.
- Yamashiro C, Sasaki K, Yabuta Y, Kojima Y, Nakamura T, Okamoto I, Yokobayashi S, Murase Y, Ishikura Y, Shirane K, et al. 2018a. Generation of human oogonia from induced pluripotent stem cells in vitro. Science. 362:356–360. Epub 2018/09/22. doi:10.1126/science.aat1674.
- Yamashiro C, Sasaki K, Yabuta Y, Kojima Y, Nakamura T, Okamoto I, Yokobayashi S, Murase Y, Ishikura Y, Shirane K, et al. 2018b. Generation of human oogonia from induced pluripotent stem cells in vitro. Science. 362:356–360.
- Yang S, Ding S, He S, He L, Gao K, Peng S, Shuai C. 2019. Differentiation of primordial germ cells from premature ovarian insufficiency-derived induced pluripotent stem cells. Stem Cell Res Ther. 10:156. doi:10.1186/s13287-019-1261-6.
- Yoshino T, Suzuki T, Nagamatsu G, Yabukami H, Ikegaya M, Kishima M, Kita H, Imamura T, Nakashima K, Nishinakamura R, et al. 2021. Generation of ovarian follicles from mouse pluripotent stem cells. Science. 373:eabe0237.
- Zarate-Garcia L, Lane SIR, Merriman JA, Jones KT. 2016. FACS-sorted putative oogonial stem cells from the ovary are neither DDX4-positive nor germ cells. Sci Rep. 6:27991–27991.