ABSTRACT
Allergic asthma, a type of chronic airway inflammation, is a global health concern because of its increasing incidence and recurrence rates. Camellia sinensis L. yields a variety type of teas, which are also used as medicinal plants in East Asia and are known to have antioxidant, anti-inflammatory, and immune-potentiating properties. Here, we examined the constituents of C. sinensis L. extract (CSE) and evaluated the protective effects of CSE on allergic asthma by elucidating the underlying mechanism. To induce allergic asthma, we injected the sensitization solution (mixture of ovalbumin (OVA) and aluminum hydroxide) into mice intraperitoneally on days 0 and 14. Then, the mice were exposed to 1% OVA by a nebulizer on days 21 to 23, while intragastric administration of CSE (30 and 100 mg/kg) was performed each day on days 18 to 23. We detected five compounds in CSE, including (-)-epigallocatechin, caffeine, (-)-epicatechin, (-)-epigallocatechin gallate, and (-)-epicatechin gallate. Treatment with CSE remarkably decreased the airway hyperresponsiveness, OVA-specific immunoglobulin E level, and inflammatory cell and cytokine levels of mice, with a decrease in inflammatory cell infiltration and mucus production in lung tissue. Treatment with CSE also decreased the phosphorylation of nuclear factor-κB (NF-κB) and the expression of matrix-metalloproteinase (MMP)-9 in asthmatic mice. Our results demonstrated that CSE reduced allergic airway inflammation caused by OVA through inhibition of phosphorylated NF-κB and MMP-9 expression.
Abbreviations
8-OHdG, 8-hydroxy-2-deoxyguanosine; AHR, airway hyperresponsiveness; BALF, bronchoalveolar lavage fluid; CSE, Camellia sinensis L. extract; ELISA, enzyme-linked immunosorbent assay; ECM, extracellular matrix; HPLC, high performance liquid chromatograph; IHC, immunohistochemistry; Ig, immunoglobulin; IL, interleukin; MMP-9, matrix metallopeptidase-9; NF-κB, nuclear factor kappa B; OVA, ovalbumin; PBS, phosphate-buffered saline.
1. Introduction
Asthma, a type of chronic airway inflammation, involves distinct respiratory symptoms, including coughing, wheezing, and shortness of breath with varying degrees (Asher et al. Citation2020). Asthma is related to interactions between airway structural cells and immune cells through several cytokines, which are accompanied by activation of eosinophilic inflammation, airway hyperresponsiveness (AHR), production of immunoglobulin (Ig)E, airway remodeling, and mucus overproduction (Luo et al. Citation2022). Corticosteroids and beta-agonists are the key therapeutics used in asthma because of their remarkable anti-inflammatory and bronchodilation effects (Miller et al. Citation2021). However, despite their efficacy, corticosteroids have limitations regarding their dose and duration due to the adverse impacts such as a reduction in growth velocity, osteoporosis, and diabetes (Heffler e lea. 2018). Therefore, identifying therapeutic agents treating asthma with high efficacy and low risk remains essential. Specific chemical compounds from natural products can be used for drug discovery due to their numerous biological and pharmacological properties (Kim et al. Citation2022a).
Numerous signaling pathways, including matrix metallopeptidase (MMP)-9 and nuclear factor kappa B (NF-κB) signaling, are involved in the development of asthma. The NF-κB is a critical player in several inflammatory diseases by regulating the expression of many proinflammatory genes (Edwards et al. Citation2009). According to the external stimuli, the free p65/p50 of NF-κB translocates into the nucleus, binding to target gene promoters (Motolani et al. Citation2020). Additionally, MMP-9, which can be synthesized and released by several lung cells, is associated with airway remodeling by degrading the extracellular matrix (ECM) of the lung in asthma. Because MMP-9 has many substrates, not only the ECM proteins but also cytokines and anti-proteases, it modulates cell migration and the activity of some proteases and cytokines (Atkinson and Senior Citation2003). Therefore, regulating the NF-κB and MMP-9 expression may be a treatment strategy for asthma.
The leaves of Camellia sinensis L. yield a variety of teas based on the degree of fermentation, and tea comes second after water as the most popular beverage worldwide (Dou Citation2018). C. sinensis was originally cultivated in China before spreading to India, Europe, and America in the seventeenth century. In traditional oriental medicine, tea is used for fever, wound healing, indigestion, and diuretic effects (Chopade et al. Citation2008). Since then, tea's chemical components and health benefits have been investigated. According to previous studies, the main components of C. sinensis L. are polyphenols (catechins and flavonoids), alkaloids (caffeine and theobromine), and amino acids, which are associated with anti-inflammatory, antioxidant, hypoglycemic, anti-cancer, metabolic regulatory, and immune-boosting effects (Sharangi Citation2009; Hayat et al. Citation2015; Li et al. Citation2022; Zhao et al. Citation2022). Considering this evidence, we hypothesized that C. sinensis L. would alleviate the ovalbumin-induced allergic airway inflammation (OVA).
Here, we evaluated the pharmacological effects of C. sinensis L. extracts (CSE) and elucidated the mechanism of action by estimating the expression of proteins in asthmatic mice.
2. Materials and methods
2.1. Analytical sample preparation
C. sinensis leaves (500 g) were extracted for 3 h at 90 °C using 5L of 70% ethanol by a heat-reflux extractor (Misung Scientific, Republic of Korea). A rotary evaporator (50 °C; SciLab, Republic of Korea) was used to filter and evaporate the extract solution. The dried extract was suspended in distilled water and freeze dried using a freeze dryer (Ilshin Biobase, Republic of Korea) for 120 h at -80 °C to give an extract powder of C. sinensis leaves (135.6 g, yield 27.1%). Then, dissolve this extract (20 mg) in 10 mL of methanol. Five reference standards (2 mg/mL, purity of >98%, ChemFaces, Wuhan, China), including (-)-epigallocatechin (EGC), caffeine (CAF), (-)-epicatechin (EC), (-)-epicatechin gallate (ECG), and (-)-epigallocatechin gallate (EGCG), were prepared as standard stock solutions in methanol (HPLC grade).
2.2. Chromatography conditions
An Agilent 1200 HPLC instrument (Santa Clara, CA, USA) was used to perform HPLC analysis. The column was Zorbax Eclipse Plus C18 (150 × 4.6 mm, 3 µm; Agilent Technologies) and thermostated at 35 °C. The mobile phase was acetonitrile (A) and 0.1% formic acid in water (B). The gradient solvent system was optimized as follows: 95–75% B (0–30 min) and 75% B (30–35 min). The flow rate was 0.8 mL/min, and the injection volume of each sample was 3 μL. Detection was conducted at 240 nm. Identification of chromatographic peak was conducted using an Agilent 1200 LC/MSD system with an electrospray ionization (ESI) source. The separation conditions for ESI/MS analyses were identical to those used for the HPLC/DAD analyses. Mass spectra were acquired in positive ion mode, and the operating parameters were as follows: nitrogen (330 °C; flow rate, 9 L/min) was used as the drying gas, and the nebulizer was set at 40 psi; the mass analyzer was scanned from 100 m/z to 1000 m/z; the capillary voltage was 3200 V; and the fragmentation amplitude was set at 1.0 V. Data were analyzed using Agilent ChemStation software. To validate the developed analytical HPLC method, its linearity, limit of detection (LOD), limit of quantification (LOQ), precision, and accuracy were evaluated. Its procedure was identical to that described in our previous study (Pak et al. Citation2024). For precision, the standard solution was analyzed 5 times over a single day for intra-day variation, and on 3 consecutive days for inter-day variation.
2.3. Animal experimental procedure
All experiments with mice were performed under protocols approved by the Institutional Animal Care and Use Committee of Chonnam National University (CNU IACUC-YB-2021-100). Adult female BALB/c mice (6 weeks old, Samtako, Osan, Republic of Korea) were acclimated a week and were randomized into groups. After intraperitoneal injection of sensitization solution (mixture of 20 µg of OVA and 2 mg of aluminum hydroxide) or phosphate-buffered saline (PBS; normal control) on days 0 and 14, the mice were exposed to 1% OVA solution or PBS (normal control) for 1 h via a nebulizer (Omron, Tokyo, Japan) on days 21 to 23. On day 18, the intragastric administration of CSE (30 and 100 mg/kg) and DEX (2 mg/kg, positive drug) was started until day 23 once a day.
2.4. Measurement of airway resistance
On day 24, the airway responsiveness of the mice was assessed at single frequency forced oscillation of Flexivent (SCIREQ, Montreal, Canada). Alfaxalone (Jurox Pty Ltd., Hunter Valley, Australia) was used to anesthetize. A tracheostomy to insert a cannula connected to a nebulizer containing PBS or methacholine (0, 10, 20, and 40 mg/mL) was performed. After inflation of the lung to open closed areas and standardization of the lung volume, the baseline values were measured in triplicate. A sequence of measurements, nebulizing methacholine solution for 10 s and recording airway resistance for 1 min and refreshed for 2 min, was automatically repeated.
2.5. Analysis of serum and bronchoalveolar lavage fluids (BALF)
Blood samples were collected on day 25, following anesthesia with alfaxalone. To obtain BALF samples, a tracheostomy was performed to insert an endotracheal tube, and the lungs were lavaged with ice-cold PBS. The collected blood samples were centrifuged to obtain serum for measuring OVA-specific IgE by an enzyme-linked immunosorbent assay (ELISA) kit (BioLegend Inc., San Diego, CA, USA). The collected BALF samples were centrifuged, and the supernatants were used to measure the levels of inflammatory cytokines using an ELISA kit (R&D Systems, Minneapolis, MN, USA). After the pellets were reconstituted in PBS, Cytospin (Hanil Electric, Wonju, Republic of Korea) was used to adhere them to a slide glass, and then Diff-Quik staining (Sysmax, Kobe, Japan) to count the differential inflammatory cells was performed. The cell counter (Countess II, Thermo Fisher Scientific, San Diego, CA, USA) was used to determine the number of total inflammatory cells in BALF. The ratio of the differential inflammatory cells was applied to the total cell count.
2.6. Histological analysis
The left lobe of the lungs fixed with 10% neutral buffered formalin was embedded in paraffin. The sectioned lung tissues were stained with hematoxylin and eosin (Sigma-Aldrich) and periodic acid–Schiff (IMEB Inc., San Marcos, CA, USA) to assess inflammation and mucus secretion indices, obtained using an image analyzer (IMT i-Solution software, IMT i-Solution Inc., Vancouver, BC, Canada). Immunohistochemical studies were conducted using a commercial kit (Vector Laboratories, Burlingame, CA, USA) to evaluate the expression of 8-hydroxy-2-deoxyguanosine (8-OHdG), phosphorylated p65 and MMP-9 with primary antibodies (diluted 1:200).
2.7. Western blot
Total lung proteins were lysed with tissue lysis reagent (Sigma-Aldrich), and 30 µg of protein were separated by SDS-PAGE and then diverted to PVDF membranes (Millipore, Burlington, MA, USA). The membranes were incubated with primary antibodies (1:1000) overnight at 4 °C and conjugated secondary antibodies (1:10,000) for 2 h at 24 °C, and finally developed with detection reagents (Thermo Fisher Scientific). The membranes were visualized using Chemi-Doc (Bio-Rad Laboratories). Used primary antibodies β-actin, p65, p-p65, IκBα, p-IκBα, and MMP-9 were acquired from Cell Signaling (Boston, MA, USA) or Abcam (Cambridge, MA, USA).
2.8. Statistical analysis
The data are expressed as the mean ± standard deviation (SD). Statistical significance was evaluated using an analysis of variance followed by a multiple comparison test with Dunnett’s adjustment. Differences were considered statistically significant at P < 0.05.
3. Results
3.1. Identification of chromatographic peaks
The wavelength of 240 nm yielded the highest S/N ratio among the five reference standards. Through the optimized chromatography conditions, five reference standards were successfully resolved and eluted within 30 min. HPLC chromatograms of the CSE and five standard mixtures are shown in (a,b), and the chemical structures of the five standards are demonstrated in (c). Comparison of the retention times, UV spectra, and m/z of molecular ions (Supplementary Figure S1) of five major peaks in CSE five reference standards confirmed the identification of peaks 1–5 as (-)-epigallocatechin, caffeine, (-)-epicatechin, (-)-epigallocatechin gallate, and (-)-epicatechin gallate, respectively ().
Figure 1. HPLC chromatograms of CSE (A) and five reference standard mixtures (B). Chemical structures of the five standards (C). Peak identification: 1, EGC [(-)-epigallocatechin]; 2, CAF (caffeine); 3, EC [(-)-epicatechin]; 4, EGCG [(-)-epigallocatechin gallate]; and 5, ECG [(-)-epicatechin gallate]. Detection was performed at 240 nm.
![Figure 1. HPLC chromatograms of CSE (A) and five reference standard mixtures (B). Chemical structures of the five standards (C). Peak identification: 1, EGC [(-)-epigallocatechin]; 2, CAF (caffeine); 3, EC [(-)-epicatechin]; 4, EGCG [(-)-epigallocatechin gallate]; and 5, ECG [(-)-epicatechin gallate]. Detection was performed at 240 nm.](/cms/asset/ea907da4-b334-4f4f-8c07-f4cb72e50597/tacs_a_2383254_f0001_oc.jpg)
Table 1. Identification of HPLC chromatographic peaks of CSE by HPLC-DAD-ESI/MS
3.2. Validation of the method and sample analysis
The regression equations, linear ranges, correlation coefficients, LOD, and LOQ values for the five reference standards are listed in Supplementary Table S1. All calibration curves displayed good linearity (r2 ≥ 0.9998) within the given concentration ranges. The RSD values of the intra- and inter-day precision for the five reference standards were in the ranges of 0.06–0.85% and 0.11–0.57%, respectively (Supplementary Table S2), indicating that the current method exhibits high precision. The recovery test was conducted by spiking three known amounts (20%, 40%, and 60%) of five reference standards into the CSE; the recovery rate of each reference standard was in the range of 87.59–96.12%, and their RSD values were less than 4% (Supplementary Table S3), indicating the high accuracy of the proposed method. The developed HPLC method was applied to the simultaneous quantitative analysis of five marker compounds in the CSE thrice. The contents of the five markers in the CSE were in the range 21.81–127.41 mg/g (); the most abundant compound in the extract was (-)-epigallocatechin gallate (127.41 ± 0.78 mg/g), followed by (-)-epigallocatechin (73.08 ± 1.49 mg/g).
Table 2. Contents of five marker compounds in the CSE
3.3. CSE inhibited AHR and OVA-specific igE in asthmatic mice
The AHR of the OVA group increased prominently as the concentration of methacholine increased compared to the NC group. The administration of CSE prevented OVA-induced increases in AHR. In particular, the CSE100 group showed an adequate deterrent effect at 40 mg/ml of methacholine ((a)). Sensitization and challenge of OVA increased the OVA-specific IgE level in the OVA group compared to that of the NC group. Compared to the OVA group, the level of OVA-specific IgE significantly decreased in both the CSE30 and CSE100 groups. CSE inhibited the synthesis of OVA-specific IgE dose-dependently ((b)).
Figure 2. CSE inhibited AHR and OVA-specific IgE in asthmatic mice. (A) Airway resistance. (B) OVA-specific IgE level in serum. NC: normal control; OVA: asthma group; DEX: asthma with dexamethasone-treated group; CSE30 and CSE100: asthma with CSE-treated group (30 and 100 mg/kg). Data presented as means ± SD (n = 7). ##p < 0.01 compared to the NC group, *p < 0.05 and **p < 0.01 compared to the OVA group.
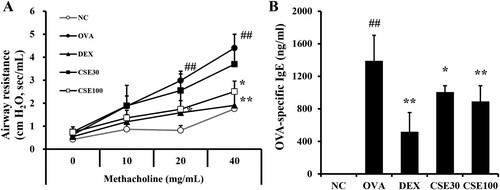
3.4. CSE reduced the number of inflammatory cells in the BALF of asthmatic mice
The number of total cells in the BALF of the OVA group was prominently increased compared to that in the NC group, and eosinophils accounted for most of the total cells ((a)). Administration of CSE reduced the number of total cells, eosinophils, and macrophages compared to the OVA group ((b–d)). In particular, the counts of eosinophils showed significant decreases in both the CSE30 and CSE100 groups, while there was no significant difference in the counts of neutrophils and lymphocytes among all groups.
Figure 3. CSE reduced the number of inflammatory cells in the BALF of asthmatic mice. (A) Representative image of BALF cells. The number of (B) total inflammatory cells, (C) eosinophils, and (D) macrophages in BALF. NC: normal control; OVA: asthma group; DEX: asthma with dexamethasone-treated group; CSE30 and CSE100: asthma with CSE-treated group (30 and 100 mg/kg). Data presented as means ± SD (n = 7). ##p < 0.01 compared to the NC group, *p < 0.05 and **p < 0.01 compared to the OVA group. Scale bar: 50 μm.
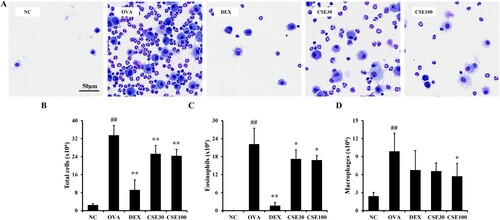
3.5. CSE prevented the production of inflammatory cytokines in the BALF of asthmatic mice
The levels of IL-4, -5, and -13 in the BALF of the OVA group were distinctly elevated compared to those of the NC group ((a–c)). The levels of all three inflammatory cytokines decreased in the CSE-treated groups compared to the OVA group. Notably, the IL-5 and -13 levels were effectively reduced in both the CSE100 and CSE30 groups.
Figure 4. CSE prevented the production of inflammatory cytokines in the BALF of asthmatic mice. The level of (A) IL-4, (B) IL-5, and (C) IL-13 in BALF. NC: normal control; OVA: asthma group; DEX: asthma with dexamethasone-treated group; CSE30 and CSE100: asthma with CSE-treated group (30 and 100 mg/kg). Data presented as means ± SD (n = 7). ##p < 0.01 compared to the NC group, *p < 0.05 and **p < 0.01 compared to the OVA group.
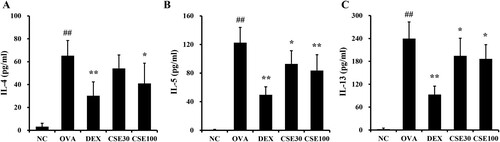
3.6. CSE suppressed airway inflammation and mucus production in asthmatic mice
Inflammatory cell recruitment was prominently increased in the lung of the OVA group compared to that of the NC group ((A, B)). The CSE-treated groups exhibited suppressed airway inflammation, which was evident in the CSE100 group. Additionally, the mucus secretion in the OVA group was significantly elevated compared to that in the NC group ((A, C)). CSE100 effectively reduced mucus production compared to that of the OVA group.
Figure 5. CSE suppressed airway inflammation and mucus secretion in lung of asthmatic mice. (a) Hematoxylin and eosin (H&E) and periodic acid-Schiff (PAS) stained lung tissue. (b) Inflammatory index. (c) Mucus secretion index. NC: normal control; OVA: asthma group; DEX: asthma with dexamethasone-treated group; CSE30 and CSE100: asthma with CSE-treated group (30 and 100 mg/kg). Data presented as means ± SD (n = 7). ##p < 0.01 compared to the NC group, *p < 0.05 and **p < 0.01 compared to the OVA group. Scale bar: 100 μm.
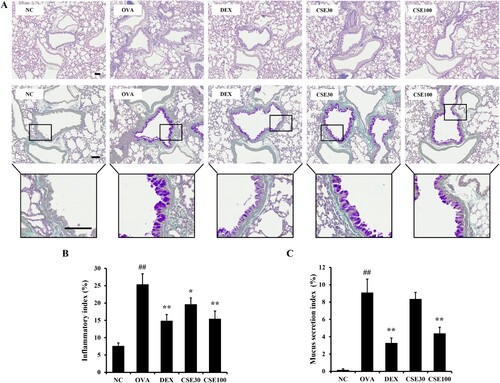
3.7. CSE suppressed oxidative damage and phosphorylated NF-κB in asthmatic mice
Compared to the control group, increased phosphorylation and nuclear translocation of NF-κB and 8-OHdG expression were observed in OVA-induced asthmatic mice by histological analysis ((A, B)). Compared to the observations in the OVA group, administration of CSE inhibited the phosphorylation and translocation of NF-κB and decreased the expression of 8-OHdG. The CSE30 and CSE100 groups showed effective decreases, which was apparent in the CSE100 group. Additionally, the phosphorylated p65 and IκBα level in the lung homogenates of the OVA group was increased compared to that of the NC group ((C, D)). CSE100 inhibited the phosphorylation of p65 and IκBα compared to that observed in the OVA group.
Figure 6. CSE suppressed the oxidative stress and phosphorylation of NF-κB in asthmatic mice. (a) 8-OHdG and phosphorylated NF-κB expression was determined by IHC. (b) 8-OHdG expression and NF-κB phosphorylation. (c) Phosphorylation of NF-κB on gel. (d) Relative protein expression value. NC: normal control; OVA: asthma group; DEX: asthma with dexamethasone-treated group; CSE30 and CSE100: asthma with CSE-treated group (30 and 100 mg/kg). Data presented as means ± SD (n = 7). ##p < 0.01 compared to the NC group, *p < 0.05 and **p < 0.01 compared to the OVA group. Scale bar: 100 μm.
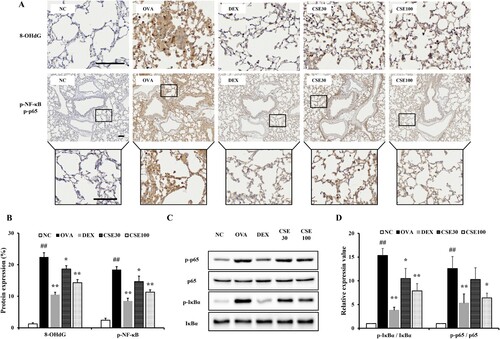
3.8. CSE inhibited MMP-9 in asthmatic mice
The MMP-9 level was elevated in asthma-induced lung tissues compared to the NC group ((A, B)). The CSE-treated groups showed distinct decreases in MMP-9 level compared to the OVA group. Similar to the result of histological analysis, MMP-9 expression in lung homogenates was increased in the OVA group compared to that in the NC group, which decreased significantly in the CSE100 group ((C, D)).
Figure 7. CSE inhibited MMP-9 expression in asthmatic mice. (a) MMP-9 expression was determined by IHC. (b) MMP-9 expression. (c) MMP-9 expression on gel. (d) Relative protein expression value. NC: normal control; OVA: asthma group; DEX: asthma with dexamethasone-treated group; CSE30 and CSE100: asthma with CSE-treated group (30 and 100 mg/kg). Data presented as means ± SD (n = 7). ##p < 0.01 compared to the NC group, *p < 0.05 and **p < 0.01 compared to the OVA group. Scale bar: 100 μm.
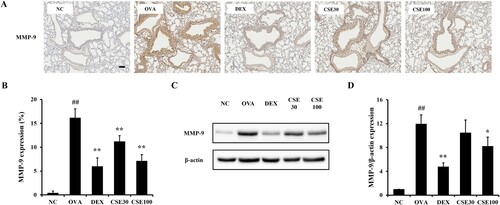
4. Discussion
Allergic asthma is steadily attracting attention not only because of its increasing incidence and recurrence rates but also due to its impact on patients’ health-related life quality, which encompasses both physical and mental aspects (Baiardini et al. Citation2006). Here, we evaluated the pharmacological effects of CSE on allergic asthma induced by OVA and revealed the underlying mechanism through a protein expression assay. Our results showed that administration of CSE decreased AHR and allergic inflammation by OVA challenge, which was demonstrated by a decrease in eosinophil counts and the levels of IL-4, -5, and -13 in BALF, as well as reduced inflammatory cell infiltration and mucus production in the lung. CSE administration suppressed the phosphorylated NF-κB and MMP-9, both associated with the inflammatory response and lung remodeling.
Allergic asthma, an eosinophilic airway inflammation, is associated with inflammatory cytokines released by CD4 Th2 cells (Hammad and Lambrecht Citation2021). Increased IL-4 promotes B cell isotype switch to produce allergen-specific IgE, and increased IL-5 results in airway eosinophilia and epithelial changes (King et al. Citation2018). Additionally, elevated IL-13 levels are involved in airway hyperreactivity, survival and activation of eosinophils, and goblet cell metaplasia, which results in hyper mucus secretion (Doran et al. Citation2017). Excessive inflammation and mucus production cause airway smooth muscle spasms and result in airway narrowing. In the present study, CSE-treated groups showed a remarkable reduction in AHR, serum IgE levels, and the number of eosinophils and Th2 cytokine levels in BALF. Additionally, treatment with CSE resulted in decreased inflammatory cell infiltration and secretion of mucus based on the histological analysis. These findings support the protective effects of CSE on the OVA-induced Th2 immune response.
Reactive oxygen species produced during asthma development often act as a stimulant of the NF-κB pathway (Lim et al. Citation2021). In resting status, NF-κB is inhibited by IκBα binding and exists in the cytoplasm of almost all cells. In perpetuating inflammatory responses to allergen exposure, IκBα is phosphorylated and then free activated NF-κB translocates into nucleus and binds to a specific region of the promoter to increase expression of inflammatory genes. Surprisingly, in asthma, NF-κB not only regulates the expression of several proinflammatory mediators but also adhesion molecules, mucin production, and angiogenic factors (Edwards et al. Citation2009; Mishra et al. Citation2018). NF-κB also induces the expression of MMP-9 from inflammatory cells (Tacon et al. Citation2010; Shin et al. Citation2020; Al-Sadi et al. Citation2021), which is regarded as a marker for monitoring airway remodeling in asthma (Barbaro et al. Citation2014). The actions of MMP on the ECM, including degradation and repair, lead to the production of bioactive pro-inflammatory molecules (Atkinson and Senior Citation2003; Manicone and McGuire Citation2008). Therefore, the downregulation of NF-κB and MMP-9 may represent an essential approach to controlling asthma. In the present study, CSE treatment significantly suppressed the oxidative stress and phosphorylated NF-κB induced by OVA challenge, which was followed by a reduction in the expression of MMP-9. IHC in the histological analysis also demonstrated decreased expression of 8-OHdG, phosphorylated NF-κB, and MMP-9. These findings indicate that the therapeutic effects of CSE on OVA-induced allergic asthma are associated with the suppression of NF-κB.
The leaves of Camellia sinensis L. are processed to make tea, a fragrant herbal beverage consumed worldwide for refreshment and health. Indeed, drinking 2–3 cups of tea per day has been shown to be associated with lower morbidity of chronic respiratory diseases (Chen et al. Citation2022b). Moreover, the main active compounds in tea, including polyphenols, saponins, polysaccharides, and theanine, have anti-allergic properties (Li et al. Citation2021). The five compounds isolated from CSE included (-)-epigallocatechin (EGC), (-)-epigallocatechin gallate (EGCG), caffeine (CAF), (-)-epicatechin (EC), and (-)-epicatechin gallate (ECG). EGC, the second most abundant catechin in CSE, exhibits radical scavenging activities (Ambigaipalan et al. Citation2020), while CAF improves airway function through bronchodilation in asthma (Welsh et al. Citation2010; Loube et al. Citation2023). EC has also been shown to have anti-inflammatory and anti-obesity benefits in mice (Bettaieb et al. Citation2016; Cremonini et al. Citation2019). EGCG, which is the most abundant component of CSE, has anti-allergic, antioxidant, anti-inflammatory, and anti-fibrotic benefits, which have been reported to benefit acute and chronic respiratory diseases including acute lung injury, bacterial pneumonia and asthma (Kim et al. Citation2006; Mokra et al. Citation2022). EGCG prevented cigarette smoke-induced inflammation and apoptosis by attenuating oxidative stress via inhibition of NF- κB activation, similar to our results (Liang et al. Citation2019). Additionally, EGCG and ECG have been shown to inhibit inflammation in the intestine, microglia, and macrophages by suppressing NF-κB (Bing et al. Citation2017; Chen et al. Citation2022a; Kim et al. Citation2022b). Furthermore, four catechins of CSE (EGC, EC, EGCG, and ECG) inhibit pro-/active MMP-9 activities in pulmonary smooth muscle cells (Sarkar et al. Citation2016). Thus, our findings are strongly supported by these earlier studies.
Our study showed that C. sinensis L. effectively alleviated allergic airway inflammation induced by OVA, partly by reducing the expression of phosphorylated NF-κB and MMP-9. These findings suggest that C. sinensis L. has potential value as a therapeutic agent for allergic asthma.
CRediT authorship contribution statement
So-Won Pak: contributed to the Methodology, Investigation, Formal analysis, Data curation, Writing – original draft. Ik Soo Lee: contributed to the Methodology, Resources, Formal analysis and Writing – original draft. Se-Jin Lee: contributed to the Formal analysis. Woong-Il Kim: contributed to the Formal analysis. Jong-Choon Kim: contributed to Formal analysis. In-Sik Shin: contributed to the, Conceptualization, Supervision, Reviewing – original draft. Taesoo Kim: contributed to Conceptualization, Funding acquisition and Reviewing original draft. All authors have read and agreed to the published version of the manuscript.
Declaration of competing interest
The authors declare that they have no competing interests.
Supplementary table.docx
Download MS Word (26.1 KB)Supplementary Material
Download MS Word (87.1 KB)Disclosure statement
No potential conflict of interest was reported by the author(s).
Data availability
Data supporting the results of this study are included in the article. If any other data may be needed, please contact the corresponding author upon request.
Additional information
Funding
References
- Al-Sadi R, Engers J, Haque M, King S, Al-Omari D, Ma TY. 2021. Matrix metalloproteinase-9 (MMP-9) induced disruption of intestinal epithelial tight junction barrier is mediated by NF-κB activation. PLoS One. 16(4):e0249544. doi:10.1371/journal.pone.0249544.
- Ambigaipalan P, Oh WY, Shahidi F. 2020. Epigallocatechin (EGC) esters as potential sources of antioxidants. Food Chem. 309:125609. doi:10.1016/j.foodchem.2019.125609.
- Asher MI, García-Marcos L, Pearce NE, Strachan DP. 2020. Trends in worldwide asthma prevalence. Eur Respir J. 56(6):2002094. doi:10.1183/13993003.02094-2020.
- Atkinson JJ, Senior RM. 2003. Matrix metalloproteinase-9 in lung remodeling. Am J Respir Cell Mol Biol. 28(1):12–24. doi:10.1165/rcmb.2002-0166TR.
- Baiardini I, Braido F, Brandi S, Canonica GW. 2006. Allergic diseases and their impact on quality of life. Ann Allergy Asthma Immunol. 97(4):419–428. doi:10.1016/S1081-1206(10)60928-3.
- Barbaro MP, Spanevello A, Palladino GP, Salerno FG, Lacedonia D, Carpagnano GE. 2014. Exhaled matrix metalloproteinase-9 (MMP-9) in different biological phenotypes of asthma. Eur J Intern Med. 25(1):92–96. doi:10.1016/j.ejim.2013.08.705.
- Bettaieb A, Cremonini E, Kang H, Kang J, Haj FG, Oteiza PI. 2016. Anti-inflammatory actions of (-)-epicatechin in the adipose tissue of obese mice. Int J Biochem Cell Biol. 81(Pt B):383–392. doi:10.1016/j.biocel.2016.08.044.
- Bing X, Xuelei L, Wanwei D, Linlang L, Keyan C. 2017. EGCG maintains Th1/Th2 balance and mitigates ulcerative colitis induced by dextran sulfate sodium through TLR4/MyD88/NF-κB signaling pathway in rats. Can J Gastroenterol Hepatol. 2017:3057268. doi:10.1155/2017/3057268.
- Chen G, Cheng K, Niu Y, Zhu L, Wang X. 2022a. (-)-Epicatechin gallate prevents inflammatory response in hypoxia-activated microglia and cerebral edema by inhibiting NF-κB signaling. Arch Biochem Biophys. 729:109393. doi:10.1016/j.abb.2022.109393.
- Chen Y, Zhang Y, Yang H, Ma Y, Zhou L, Lin J, Hou Y, Yu B, Wang Y. 2022b. Association of coffee and Tea consumption with cardiovascular disease, chronic respiratory disease, and their comorbidity. Mol Nutr Food Res. 66(24):e2200419. doi:10.1002/mnfr.202200419.
- Chopade V, Phatak A, Upaganlawar A, Tankar A. 2008. Green tea (camellia sinensis): chemistry, traditional, medicinal uses and its pharmacological activities-a review. Pharmacogn Rev. 2(3):157.
- Cremonini E, Fraga CG, Oteiza PI. 2019. (-)-Epicatechin in the control of glucose homeostasis: involvement of redox-regulated mechanisms. Free Radic Biol Med. 130:478–488. doi:10.1016/j.freeradbiomed.2018.11.010.
- Doran E, Cai F, Holweg CTJ, Wong K, Brumm J, Arron JR. 2017. Interleukin-13 in asthma and other eosinophilic disorders. Front Med (Lausanne). 4:139. doi:10.3389/fmed.2017.00139.
- Dou QP. 2018. Tea in health and disease. Nutrients. 11(4):929. doi:10.3390/nu11040929.
- Edwards MR, Bartlett NW, Clarke D, Birrell M, Belvisi M, Johnston SL. 2009. Targeting the NF-kappaB pathway in asthma and chronic obstructive pulmonary disease. Pharmacol Ther. 121(1):1–13. doi:10.1016/j.pharmthera.2008.09.003.
- Hammad H, Lambrecht BN. 2021. The basic immunology of asthma. Cell. 184(6):1469–1485. doi:10.1016/j.cell.2021.02.016.
- Hayat K, Iqbal H, Malik U, Bilal U, Mushtaq S. 2015. Tea and its consumption: benefits and risks. Crit Rev Food Sci Nutr. 55(7):939–954. doi:10.1080/10408398.2012.678949.
- Heffler E, Madeira LNG, Ferrando M, Puggioni F, Racca F, Malvezzi L, Passalacqua G, Canonica GW. 2018. Inhaled corticosteroids safety and adverse effects in patients with asthma. J Allergy Clin Immunol Pract. 6(3):776–781. doi:10.1016/j.jaip.2018.01.025.
- Kim S, Lim SW, Choi J. 2022a. Drug discovery inspired by bioactive small molecules from nature. Anim Cells Syst (Seoul). 26(6):254–265. doi:10.1080/19768354.2022.2157480.
- Kim SH, Park HJ, Lee CM, Choi IW, Moon DO, Roh HJ, Lee HK, Park YM. 2006. Epigallocatechin-3-gallate protects toluene diisocyanate-induced airway inflammation in a murine model of asthma. FEBS Lett. 580(7):1883–1890. doi:10.1016/j.febslet.2006.02.052.
- Kim SR, Seong KJ, Kim WJ, Jung JY. 2022b. Epigallocatechin gallate protects against hypoxia-induced inflammation in microglia via NF-κB suppression and Nrf-2/HO-1 activation. Int J Mol Sci. 23(7):4004. doi:10.3390/ijms23074004.
- King GG, James A, Harkness L, Wark PAB. 2018. Pathophysiology of severe asthma: we've only just started. Respirology. 23(3):262–271. doi:10.1111/resp.13251.
- Li MY, Liu HY, Wu DT, Kenaan A, Geng F, Li HB, Gunaratne A, Li H, Gan RY. 2022. L-Theanine: A unique functional amino acid in Tea (camellia sinensis L.). with multiple health benefits and food applications. Front Nutr. 9:853846. doi:10.3389/fnut.2022.853846.
- Li QS, Wang YQ, Liang YR, Lu JL. 2021. The anti-allergic potential of tea: a review of its components, mechanisms and risks. Food Funct. 12(1):57–69. doi:10.1039/d0fo02091e.
- Liang Y, Ip MSM, Mak JCW. 2019. (-)-Epigallocatechin-3-gallate suppresses cigarette smoke-induced inflammation in human cardiomyocytes via ROS-mediated MAPK and NF-κB pathways. Phytomedicine. 58:152768. doi:10.1016/j.phymed.2018.11.028.
- Lim JO, Song KH, Lee IS, Lee SJ, Kim WI, Pak SW, Shin IS, Kim T. 2021. Cimicifugae rhizoma extract attenuates oxidative stress and airway inflammation via the upregulation of Nrf2/HO-1/NQO1 and downregulation of NF-κB phosphorylation in ovalbumin-induced asthma. Antioxidants (Basel). 10(10):1626. doi:10.3390/antiox10101626.
- Loube JM, Gidner S, Venezia J, Ryan H, Neptune ER, Mitzner W, Dalesio NM. 2023. Nebulized caffeine alleviates airway hyperresponsiveness in a murine asthma model. Am J Physiol Lung Cell Mol Physiol. 325(4):L500–L507. doi:10.1152/ajplung.00065.2023.
- Luo W, Hu J, Xu W, Dong J. 2022. Distinct spatial and temporal roles for Th1, Th2, and Th17 cells in asthma. Front Immunol. 13:974066. doi:10.3389/fimmu.2022.974066.
- Manicone AM, McGuire JK. 2008. Matrix metalloproteinases as modulators of inflammation. Semin Cell Dev Biol. 19(1):34–41. doi:10.1016/j.semcdb.2007.07.003.
- Miller RL, Grayson MH, Strothman K. 2021. Advances in asthma: New understandings of asthma's natural history, risk factors, underlying mechanisms, and clinical management. J Allergy Clin Immunol. 148(6):1430–1441. doi:10.1016/j.jaci.2021.10.001.
- Mishra V, Banga J, Silveyra P. 2018. Oxidative stress and cellular pathways of asthma and inflammation: therapeutic strategies and pharmacological targets. Pharmacol Ther. 181:169–182. doi:10.1016/j.pharmthera.2017.08.011.
- Mokra D, Adamcakova J, Mokry J. 2022. Green Tea polyphenol (-)-epigallocatechin-3-gallate (EGCG): A time for a New player in the treatment of respiratory diseases? Antioxidants (Basel). 11(8):1566. doi:10.3390/antiox11081566.
- Motolani A, Martin M, Sun M, Lu T. 2020. Phosphorylation of the regulators, a complex facet of NF-κB signaling in cancer. Biomolecules. 11(1):15. doi:10.3390/biom11010015.
- Pak So-Won, Lee Ik Soo, Kim Woong-Il, Lee Se-Jin, Yang Yea-Gin, Shin In-Sik, Kim Taesoo. 2024. Melia azedarach L. reduces pulmonary inflammation and mucus hypersecretion on a murine model of ovalbumin exposed asthma. Journal of Ethnopharmacology. 320:117426. doi:10.1016/j.jep.2023.117426.
- Sarkar J, Nandy SK, Chowdhury A, Chakraborti T, Chakraborti S. 2016. Inhibition of MMP-9 by green tea catechins and prediction of their interaction by molecular docking analysis. Biomed Pharmacother. 84:340–347. doi:10.1016/j.biopha.2016.09.049.
- Sharangi AB. 2009. Medicinal and therapeutic potentialities of tea (camellia sinensis L.)–A review. Food Res Int. 42(5-6):529–535.
- Shin NR, Lee AY, Song JH, Yang S, Park I, Lim JO, Jung TY, Ko JW, Kim JC, Lim KS, et al. 2020. Scrophularia buergeriana attenuates allergic inflammation by reducing NF-κB activation. Phytomedicine. 67:153159. doi:10.1016/j.phymed.2019.153159.
- Tacon CE, Wiehler S, Holden NS, Newton R, Proud D, Leigh R. 2010. Human rhinovirus infection up-regulates MMP-9 production in airway epithelial cells via NF-{kappa}B. Am J Respir Cell Mol Biol. 43(2):201–209. doi:10.1165/rcmb.2009-0216OC.
- Welsh EJ, Bara A, Barley E, Cates CJ. 2010. Caffeine for asthma. Cochrane Database Syst Rev. 2010(1):CD001112. doi:10.1002/14651858.CD001112.pub2.
- Zhao T, Li C, Wang S, Song X. 2022. Green Tea (camellia sinensis): A review of Its phytochemistry, pharmacology, and toxicology. Molecules. 27(12):3909. doi:10.3390/molecules27123909.