ABSTRACT
The aim of this study was to observe the impact of the mammalian sterile 20-like kinase 1-c-Jun N-terminal kinase (MST1-JNK) signaling pathway on apoptosis in colorectal cancer (CRC) cells induced by Taurine (Tau). Caco-2 and SW620 cells transfected with p-enhanced green fluorescent protein (EGFP)-MST1 or short interfering RNA (siRNA)-MST1 were treated with Tau for 48 h. Apoptosis was detected by flow cytometry, and the levels of MST1 and JNK were detected by western blotting. Compared with the control group, 80 mM Tau could significantly induce apoptosis of CRC cells, and the apoptotic rate increased with increasing Tau concentration (P < 0.01). Meanwhile, the protein levels of MST1 and phosphorylated (p)-JNK in Caco-2 cells increased significantly (P < 0.01). The apoptotic rate of the p-EGFP-MST1 plasmid-transfected cancer cells was significantly higher than that of the control group (P < 0.05); however, the apoptotic rate of the p-EGFP-MST1+Tau group was increased further (P < 0.01). Silencing the MST1 gene could decrease the apoptotic rate of cancer cells, and Tau treatment could reverse this decrease. Blocking the JNK signaling pathway significantly reduced the Tau-induced apoptotic rate of CRC cells. Thus, the MST1-JNK pathway plays an important role in Tau-induced apoptosis of CRC cells.
1. Introduction
Colorectal cancer (CRC) is one of the most common malignancies in humans [Citation1]. The International Agency for Research on Cancer (IARC) reported that the incidence of CRC ranks third among global malignancies, and mortality rate due to CRC has risen to fourth for malignant tumors [Citation1]. Therefore, the prevention and treatment of CRC has become a hotspot in cancer control research. Presently, research on tumor prevention and treatment is directed toward exploring the association between abnormal cell signaling pathways and the occurrence and development of tumors. The Hippo pathway is a hotspot for studying the occurrence, development, and targeted therapy for CRC. The mammalian sterile 20-like kinase 1 (MST1/2) is a core member of the Hippo signaling pathway, and its abnormality is commonly observed in CRC [Citation2] and liver cancer [Citation3]. Many studies have confirmed the importance of the MST-Hippo pathway in the occurrence and development of CRC [Citation4,Citation5]. Inhibitors targeting this pathway play important roles in preventing and treating CRC. Other studies have shown that the c-Jun N-terminal kinase (JNK) signaling pathway is involved in cell proliferation and differentiation. Its dysfunction can cause ischemia/reperfusion injury [Citation6], diabetes [Citation7], or tumors [Citation8]. Thus, the JNK signaling pathway has become a potential clinical molecular therapeutic target.
Taurine (Tau) is an endogenous cell protective agent. Recent studies have found that it has good anti-tumor effects [Citation9–Citation14]. For example, Tau can inhibit the in vitro invasion and migration of breast cancer cells and has a better therapeutic effect against methylbenzanthracene (DMBA)-induced breast cancer [Citation11,Citation12]. Our previous studies have shown that Tau can inhibit the apoptosis of cardiomyocytes subjected to ischemia/reperfusion [Citation15] and can induce (or promote) apoptosis in colon cancer [Citation10], breast cancer [Citation13,Citation14], or liver cancer cells [Citation12]. This effect is achieved by up-regulating the expression of BCL-2, PMA, and P53, while down-regulating the expression of BCL-2. Furthermore, Tau has better inhibitory effect toward the proliferation of P53−/− tumor cells than toward P53+/+ tumor cells [Citation10], indicating that the Tau-induced apoptosis of cancer cells is not only related to the mitochondrial apoptotic pathway but also involves other apoptotic signaling pathways. Recent studies have found that MST1 [Citation16] and JNK [Citation17] play important roles in the occurrence and development of colon cancer; however, whether the MST1-JNK signaling pathway is involved in the Tau-induced apoptosis of CRC cells has not been reported till date. In the present study, the CRC cell lines, SW620 and Caco-2, were used as the study subjects, in which the expression of the MST1 gene was regulated and the JNK pathway was inhibited to investigate the effect of the MST1-JNK signaling pathway in Tau-induced apoptosis of CRC cells. This allowed us to explore the molecular mechanism underlying the inhibition of the growth of CRC by Tau, thus, providing experimental evidence for the clinical application of Tau to treat CRC.
2. Materials and methods
2.1. Cell line and culture
The human CRC cells Caco-2 and SW620 (purchased from Cell Bank of Type Culture Collection Committee, CAS) were cultured in the complete Dulbecco’s modified eagle’s medium (DMEM) (Hyclone) containing 10% fetal bovine serum (FBS) (TransGen Biotech Co. Ltd.) at 37°C under 5% CO2 conditions.
2.2. MTS colorimetry
The cells in the logarithmic growth phase were randomly divided into eight groups [Control (CON), Tau 10 mM (T10), Tau 20 mM (T20), Tau 40 mM (T40), Tau 80 mM (T80), Tau 160 mM (T160), Tau 320 mM (T320), and DDP 5 μg/mL (DDP)] and seeded into culture plates with appropriate amount of sterile phosphate-buffered saline (PBS). The cells were incubated with the aforementioned factors for 24, 48, and 72 h, respectively, and then 20 μl/well Cell Titer 96® AQueous One Solution Reagent (containing3-(4,5-dimethylthiazol-2-yl)-5-(3-carboxymethoxyphenyl)-2- (4-sulfophenyl)-2H-tetrazolium) (MTS)) was added; the samples were incubated for another 4 h. The OD value of each well was measured at 490 nm using a microplate reader to plot the cell growth curve. Proliferation inhibition rate (%) = (1-OD value of test group/OD value of CON) × 100%.
2.3. Flow cytometry
The cells in the logarithmic growth phase were randomly grouped and treated with different methods. Cells (1–5 × 105) were then collected, and 500 μl of Binding Buffer was added to suspend the cells. Annexin V-FITC (5 μl; BestBio, Shanghai, China) was then added to the cells, vortexed, and 5 μl of propidium iodide was added, mixed evenly, and incubated for 5–15 min at room temperature in the dark. Apoptotic cells were then detected using a FACScalibur flow cytometer (Becton Dickinson, USA).
2.4. Hoechst 33342 staining
The cells in the logarithmic growth phase were randomly grouped and treated with different methods. The cells were placed in CO2 incubator for 48 h at 37°C. After incubation, the cells were washed with PBS three times. The solution of Hoechst 33342 was added to the cells, and after 20 min of staining the cells were observed under a fluorescence microscope at 200 × magnification. Apoptosis rate (%) = apoptotic cells/total cells × 100%.
2.5. Plasmid vector and transfection
The plasmid p-EGFP-MST1, which overexpresses the enhanced green fluorescent protein (EGFP) fused to the human wild-type MST1, and the empty vector p-EGFP-NC, were synthesized and characterized by Chuanming Xu in our laboratory.
The recombinant plasmid, pEGFP-MST1, and the empty vector, pEGFP-NC, (Clontech, Mountain View, USA) were treated with the PolyJet™ in vitro DNA transfection reagent (Cat. # SL100688; SignaGen, Rockville, USA) and transfected into SW480 cells. First, the SW480 cells were cultured in 6-well plate still 60%–70% confluence was achieved. The original medium was discarded, and 900 ml of fresh complete medium was added to each well; the cells were cultured for another 30–60 min. Thereafter, 50 μl of transfection solution was added and gently mixed; the cells were cultured at 37°C under 5% CO2 for 5 h. The medium was then replaced with 10% FBS-containing DMEM (Hyclone), and the cells were incubated for another 24–48 h. The transfection efficiency was then determined, and the cells were photographed.
Preparation of the transfection solution: for solution A, 1 μg of plasmid was dissolved in 50 μl of serum-free DMEM medium and pipetted three to four times; for solution B, 3 μl of PolyJet™ reagent was dissolved in 50 μl of serum-free DMEM medium and pipetted three to times; and for solution C (transfection solution), solution B was added to solution A and rapidly pipetted three to four times, and the cells were cultured for 15 min at room temperature.
Grouping and treatment for pEGFP-MST1 transfection: (1) Control (CON): the cells were cultured with FBS-free DMEM for 6 h, and the medium was then changed to the complete medium; the cells were cultured for another 48 h. (2) In the p-EGFP-NC group, the cells were transfected with the empty vector, p-EGFP-NC, and cultured for 48 h. (3) In the p-EGFP-MST1 group, the cells were transfected with plasmid, p-EGFP-MST1, and cultured for 48 h. (4) In the p-EGFP-MST1+ Tau group, the cells were transfected with plasmid, p-EGFP-MST1, on the second day of seeding, cultured for 24 h, 160 mmol/l of Tau was then added, and the cells were cultured for another 24 h.
Grouping and treatment for siRNA-MST1 transfection: (1) CON: the cells were cultured with FBS-free DMEM for 6 h, and medium was then changed to the complete medium; the cells were cultured for another 48 h. (2) In the siRNA-NC group, the cells were transfected with the control short interfering RNA (siRNA-NC) and cultured for 48 h. (3) In the siRNA-MST1 group, the cells were transfected with siRNA-MST1 and cultured for 48 h. (4) In the siRNA-MST1+Tau group, the cells were transfected with siRNA-MST1 on the second day of seeding, cultured for 24 h, 160 mmol/l of Tau was added, and the cells were cultured for another 24 h.
2.6. Western blotting
Total proteins were extracted from the cells and denatured; the extracted protein (30 μg/well protein) was subjected to SDS-PAGE. The proteins were then transferred onto a polyvinylidene difluoride membrane (PVDF, Millipore, USA) by the wet transfer method. The membrane was then incubated with an MST1 monoclonal antibody (ab51134, Abcam), JNK1 polyclonal antibodies (ab134466, Abcam), phosphorylated (p)-JNK polyclonal antibodies (ab18680, Abcam), BAX polyclonal antibodies (50599-2-lg, SAB), and BCL-2 polyclonal antibodies (#21059, SAB) (1:200) overnight at 4°C. The membrane was then incubated with the horseradish peroxidase-conjugated secondary antibodies (1:2000) for 1.5 h; the ECL immunoassay was then performed in the dark. The immunoreactive protein bands were scanned using the Image-Pro Plus 6.0 image analysis software and subjected to semi-quantitative analysis using antibodies against β-actin (AF7018, Santa Cruz) as the control.
2.7. Impact of blocking the JNK pathway on the levels of apoptosis-related proteins
Cells in the logarithmic growth phase were randomly divided into five groups and treated with different methods:
CON: The original medium was changed to fresh complete medium on the second day of plate seeding, and the cells were cultured for 48 h.
DMSO group: The cells were cultured in DMSO (at the same final concentration of DMSO as that in the JNK group) for 1 h, and then the DMSO was replaced with fresh culture medium; the cells were cultured for another 48 h.
JNK group: The cells were incubated with the JNK inhibitor, SP600125(final concentration: 25 μM in DMSO), for 1 h and then the inhibitor containing medium was replaced with fresh culture medium; the cells were cultured for another 48 h.
SP600125+Tau group: The cells were cultured with the JNK inhibitor SP600125 (final concentration: 25 μM in DMSO) for 1 h, and then SP600125 containing medium was removed; the cells were cultured with Tau (final concentration:160 mM) for another 48 h.
SP600125 + p-EGFP-MST1 group: The p-EGFP-MST1-transfected CRC cells were cultured with the JNK inhibitor SP600125 (final concentration: 25 μM in DMSO) for 1 h, and then the medium containing SP600125 was removed; the cells were cultured for another 48 h.
From all five groups, the cells were collected and the total proteins were extracted for protein detection.
2.8. Statistical analysis
SPSS19.0 statistical software was used to compare the parameters among different groups using one-way analysis of variance (ANOVA); the pairwise comparison of inter-group parameters used the q test. P < 0.05 was considered statistically significant.
3. Results
3.1. Impact of Tau on proliferation and apoptosis of CRCs
shows that after Caco-2 and SW620 cells were treated with Tau (0, 80, 160, and 320 mM) and DDP (5 μg/mL) for 48 h, Tau concentrations higher than 80 mM significantly induced apoptosis in Caco-2 and SW620 cells. Compared with that in the CON group, the apoptosis rate in the two types of cells increased gradually with increasing Tau concentration, and the differences were all statistically significant (P < 0.01).
3.2. Impact of Tau on MST1, JNK, and p-JNK proteins in CRC cells
shows that in Caco-2 cells, the protein levels of MST1 and p-JNK increased gradually as the Tau concentration increased (P < 0.01); however, there was no significant difference in the level of JNK. High concentrations of Tau had no significant impact on the protein levels of MST1, JNK, and p-JNK in SW620 cells (P > 0.05).
Figure 2. Impact of Tau on Mst1, JNK, and p-JNK proteins in Caco-2 and SW620. (A) In Caco-2, the protein expressions of Mst1 and p-JNK increased gradually with the increase of the Tau concentration. (B) In SW620, Tau had no significant impact on the protein expressions of Mst1, JNK, and p-JNK in SW620. **P < 0.01 and *P < 0.05 vs. Control.
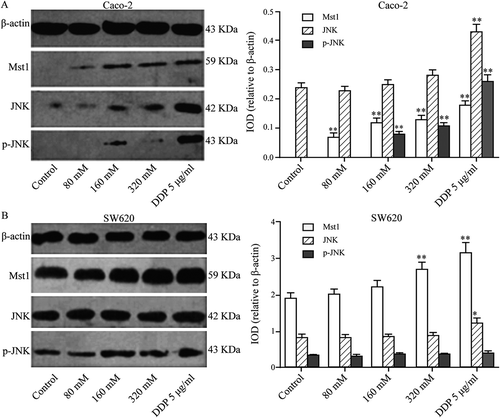
3.3. Impact of MST1 transfection on Tau-regulated proliferation and apoptosis of CRC cells
The MST1-transfected Caco-2 and SW620 cells were treated with Tau (160 mM) for 48 h, and the impact of Tau on the proliferation and apoptosis of these two CRC cell lines was observed. shows that the proliferation inhibition rate of the two cells after MST1 transfection was significantly increased compared with that of the p-EGFP-NC group, and the differences were statistically significant (P < 0.01). Compared with that in the p-EGFP-NC group, the proliferation inhibition rate in the p-EGFP-MST1+Tau group for both these two cell types was significantly increased (P < 0.01), and was much higher than that in the cells only transfected with MST1 (P < 0.05). The results showed that high expression of MST1 gene could inhibit the proliferation of CRC cells, and the treatment with Tau could further inhibit their proliferation ()).
Figure 3. Impact of Mst1 and Tau (160 mM) on proliferation and apoptosis of Caco-2 and SW620. The high expression of Mst1 alone or in combination with Tau can greatly improve the proliferation inhibitory (A) and apoptosis rate (B, C) in both Caco-2 and SW620. The Q2 (AnnexinV+ PI+) indicates the late stage for cell death and Q3 (Annexin+PI-) presents the early stage for cell. **P < 0.01 vs. group p-EGFP-NC; #P < 0.05 vs. group p-EGFP-Mst1.
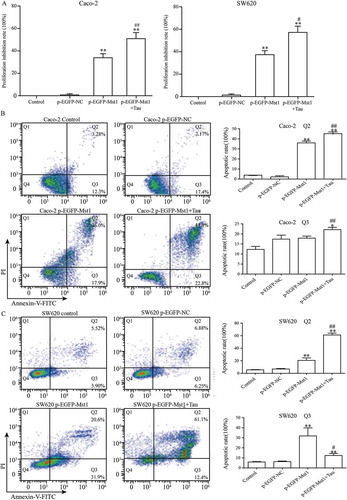
The MST1-transfected Caco-2 and SW620 cells were treated with Tau for 48 h and then apoptosis was determined. ), () show that the apoptotic rates of the two cell lines after MST1 transfection were significantly increased compared with those in the p-EGFP-NC group (P < 0.01). The total apoptotic rates in the p-EGFP-MST1+Tau groups for the two cell lines were further significantly increased (P < 0.05). It can be seen from that the nuclei in the control group and the p-EGFP-NC group are light blue with intact cell morphology, but the nuclei in the p-EGFP-Mst1 group and the p-EGFP-Mst1+Tau group are darker blue with broken cells and visible apoptotic bodies. The results showed that the apoptosis in the p-EGFP-Mst1 group was significantly higher than the p-EGFP-NC group, and the increase of apoptosis was more significant in the p-EGFP-Mst1+Tau group. (P < 0.01);Compared with the p-EGFP-Mst1 group, the apoptosis rate in the p-EGFP-Mst1+ Tau group also increased significantly (P < 0.01). The results showed that MST1 can promote the apoptosis of CRC cells, which can be further increased by the combined effect of MST1 and Tau; therefore, MST1 and Tau have synergistic effects on apoptosis in CRC cells.
Figure 4. Fluorescence microscopic (at 200× magnification) study of apoptosis in both Caco-2 and SW620 cells using Hoechst 33342 staining dye. The high expression of Mst1 alone or in combination with Tau can greatly improve the apoptosis rate in both Caco-2 and SW620. **P < 0.01 vs. group p-EGFP-NC; ##P < 0.01 vs. group p-EGFP-Mst1.
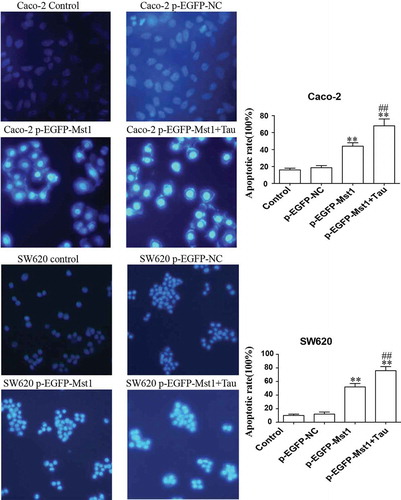
3.4. Impact of MST1 and tau on JNK, p-JNK, BAX, and BCL-2 proteins in CRC cells
shows that compared with those in the CON group, the protein levels ofMST1, JNK, p-JNK, and BAX in Caco-2 cells in the p-EGFP-MST1 group were significantly upregulated (P < 0.01); however, the level of BCL-2 was downregulated (P < 0.01). In the p-EGFP-MST1+ Tau group, for the Caco-2 cells, the levels of JNK, p-JNK, and BAX proteins in group were more significantly upregulated (P < 0.01), while that of BCL-2 was significantly downregulated (P < 0.01). Similar results were also observed for the SW620 cells ().
Figure 5. Impact of Mst1 and Tau on JNK, p-JNK, Bax, and Bcl-2 proteins in CRCs. The protein levels of Mst1, JNK, p-JNK, and Bax of Caco-2 and SW60 were significantly upregulated but Bcl-2 was downregulated in both group p-EGFP-Mst1 and p-EGFP-Mst1+ Tau. *P < 0.05, **P < 0.01 vs group Control or p-EGFP-NC; #P < 0.05, ##P < 0.01 vs group p-EGFP-Mst1.
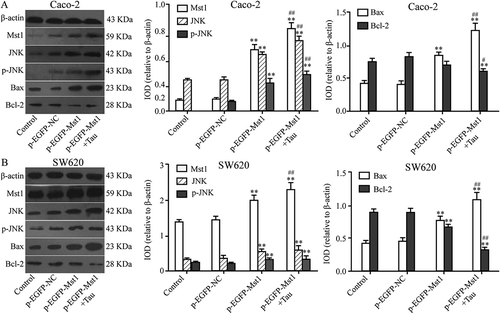
3.5. Impact of silencing MST1 on Tau-induced apoptosis of Caco-2 and SW620 cells
shows that the apoptotic rates of the two cell lines after silencing the MST1 gene decreased significantly compared with those of the cells of the siRNA-NC group (P < 0.01). The apoptotic rates for both cell lines in thep-EGFP-MST1+Tau group were significantly increased compared with those in the siRNA-NC group (P < 0.05); the apoptotic rates further significantly increased compared with those for the two cell lines in the siRNA-MST1 group (P < 0.01). shows that compared with the siRNA-NC group, the apoptosis is significantly decreased in the siRNA-Mst1 group, but the apoptosis is significantly increased in the siRNA-Mst1+Tau group (P < 0.01); compared to the siRNA-Mst1 group, the siRNA-Mst1+Tau group shows more significantly increased apoptosis rate (P < 0.01). The results showed that downregulating the MST1 gene could inhibit apoptosis in CRC cells, but Tau treatment could significantly promote apoptosis.
Figure 6. Impact of silencing Mst1 on Tau-promoted apoptosis of CRCs. The apoptotic rates of the Caco-2 and SW620 were significantly decreased in group siRNA-Mst1 but increased in group siRNA-Mst1+ Tau. The Q2 (AnnexinV+ PI+) indicates the late stage for cell death and Q3 (Annexin+ PI-) presents the early stage for cell. *P < 0.05, **P < 0.01 vs group Control or siRNA-NC; ##P < 0.01 vs group siRNA-Mst1.
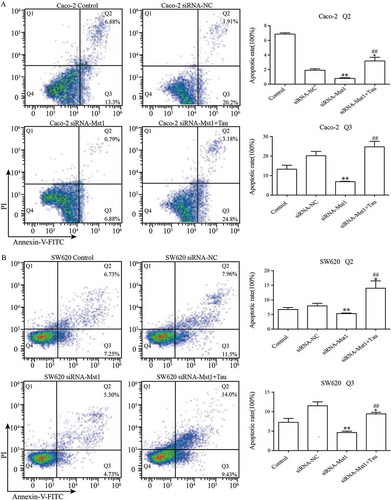
Figure 7. Fluorescence microscopic (at 400× magnification) study of apoptosis in both Caco-2 and SW620 cells using Hoechst 33342 staining dye. The apoptotic rates of the Caco-2 and SW620 were significantly decreased in group siRNA-Mst1 but increased in group siRNA-Mst1+ Tau. **P < 0.01 vs group Control or siRNA-NC; ##P < 0.01 vs group siRNA-Mst1.
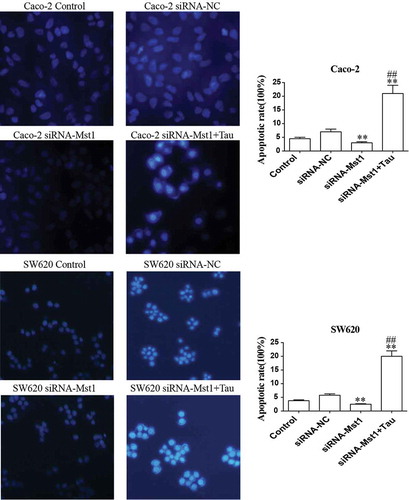
3.6. Impact of silencing MST1 on JNK, BAX BCL-2, and p-JNK proteins in tau-regulated CRC cells
The impact of silencing MST1 on JNK, BAX BCL-2, and p-JNK protein levels in Tau-regulated Caco-2 and SW620 cells was tested by western blotting (). After silencing the MST1 gene, the BAX protein level was significantly downregulated compared with that in the CON group (P < 0.01); however, the BCL-2 protein level was significantly upregulated (P < 0.05); the JNK, p-JNK, and BAX levels in SW620 cells were significantly downregulated (P < 0.01); however, the level of BCL-2 was significantly regulated (P < 0.01). In Caco-2 cells ()), the level of BAX in the siRNA-MST1+Tau group was significantly higher than that in the CON or siRNA-MST1 groups (P < 0.01); however, the level of BCL-2 was significantly decreased (P < 0.01). In SW620 cells ()), the levels of JNK (P < 0.01), p-JNK (P < 0.01), and BCL-2 in the siRNA-MST1+Tau group were significantly reduced, while the BAX level was significantly increased compared with that in the CON group (P < 0.01). Compared with those in the siRNA-MST1 group, the levels of JNK, p-JNK, and BCL-2 in the siRNA-MST1+Tau were significantly reduced, while the BAX level was significantly increased (P < 0.01).
Figure 8. Impact of silencing Mst1 on apoptosis-related proteins in Tau-regulated CRCs. In contrast to group siRNA-Mst1, the protein expression of Bax increased and Bcl-2 decreased significantly in group siRNA-Mst1+ Tau in both Caco-2 and SW620. *P < 0.05, **P < 0.01 vs group Control or siRNA-NC; #P < 0.05, ##P < 0.01 vs group siRNA-Mst1.
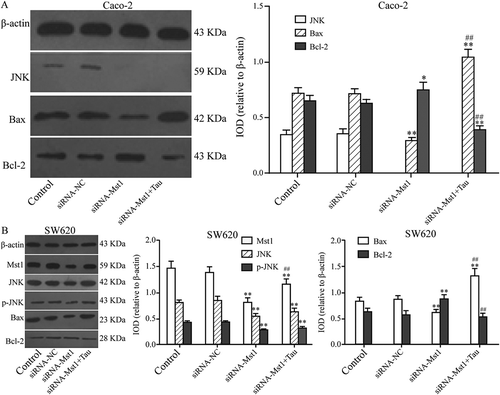
3.7. Impact of the JNK Inhibitor SP600125 and Tau on JNK, BAX BCL-2, and p-JNK proteins in Caco-2 and SW620 cells
The impact of SP600125 alone and of SP600125 in combination with Tau or p-EGFP-MST1, on the levels of MST1, JNK, p-JNK, BAX, and BCL-2 proteins in the two types of CRC cells, were analyzed by western blotting. shows that compared with their levels in the DMSO group, the levels of JNK, p-JNK, and BAX in both types of SP600125-treated cells were significantly decreased; however, the level of BCL-2 was significantly increased (P < 0.05). In contrast, the level of MST1 was not significantly different. Compared with those in the SP600125 group, the levels of MST1, JNK, p-JNK, and BAX in Caco-2 cells in the SP600125+Tau group were significantly increased ()) (P < 0.01); however, the level of BCL-2 was significantly decreased (P < 0.01). The levels of BCL1 and JNK in SW620 cells ()) did not show a significant change; however, the levels of p-JNK and BAX were significantly increased (P < 0.01) while the level of BCL-2 was significantly decreased (P < 0.01).
Figure 9. Impact of JNK inhibitor on apoptosis-related proteins in Tau-regulated CRCs. Compared to the group SP600125, the expressions of JNK, p-JNK, and Bax were increased and Bcl-2 decreased in group SP600125+ Tau and SP600125 + p-EGFP-Mst1 of Caco-2 and SW620. **P < 0.01 vs group DMSO or Control; ##P < 0.01 vs SP600125.
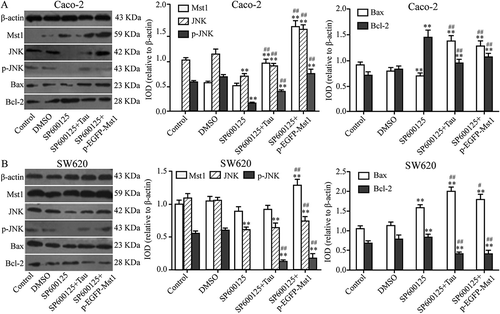
4. Discussion
Tau (β-aminoethanesulfonic acid, C2H7NO3S), also known as cholic acid, is the most abundant β-amino acid in vivo. It normally exists in tissues and cells in the free form, does not participate in protein synthesis, and is an important endogenous anti-injury substance in the human body [Citation18,Citation19]. Recent studies have shown that Tau is involved in the most important pathway related to tumor metabolism [Citation20,Citation21]. The anti-tumor mechanism of Tau is mainly related to Tau-induced apoptosis, synergism, and attenuation [Citation9–Citation13], via regulating the expression of key genes at both the transcriptional and translational levels. For example, Zhang et al. showed that Tau prompted the transcription and translation of the PUMA gene in HT-29 colorectal cancer cells [Citation10], and Sorensen et al. indicated that Tau increases LRRC8A expression in wild-type A2780 cells [Citation22]; however, the specific mechanisms underlying these effects have not been elucidated. In the present study, we investigated the impact of Tau on apoptosis in CRC cells by changing the expression of MST1 gene or inhibiting the JNK pathway, aiming to investigate the roles of the MST1-JNK signaling pathway in the Tau-induced apoptosis in CRC cells. The results confirmed that the MST1-JNK pathway plays an important role in Tau-induced apoptosis of CRC cells; Tau at concentrations greater than 80 mM could significantly induce apoptosis in Caco-2 and SW620 cells (P < 0.01), and the protein levels of MST1 and p-JNK also increased gradually with increasing Tau concentrations (P < 0.01). Overexpression of the MST1 gene could promote apoptosis in CRC cells and inhibit their proliferation. These effects were further enhanced when MST1 overexpression occurred in combination with Tau treatment (). In contrast, silencing the MST1 gene significantly reduced the apoptosis promoting and cancer cell proliferation inhibiting effects of Tau; the level of BAX significantly decreased (P < 0.01), but the level of BCL-2 significantly increased (P < 0.05). Our results showed that Tau could induce apoptosis in CRC cells and inhibit their proliferation, and the mechanism underlying these effects involves increasing the level of MST1 in CRC cells, which then upregulates the expression of pro-apoptotic proteins, such as BAX, but downregulates the expression of anti-apoptotic proteins, such as BCL-2. Recent studies have found that the expression levels of MST1 and YAP1 in human colon cancer tissue are significantly higher than those in the adjacent tissues [Citation23,Citation24]. In human primary colon cancer, adenomatous colonic polyposis, or mouse multiple intestinal tumor, the expression of YAP is upregulated, which could also promote the growth of transplanted tumors in a mouse model. Therefore, the MST-Hippo pathway plays an important role in the occurrence and development of CRC, and inhibitors against this pathway have become important targets for research to prevent and treat colon cancer [Citation4,Citation5].
JNK, also known as stress-activated kinase (SAPK), is one of the major members of the mitogen-activated protein kinase (MAPK) superfamily. JNK is related to the occurrence and development of tumors [Citation25], ischemia-reperfusion (I/R) injury [Citation26], immune inflammatory response [Citation27], and other diseases. JNK is mainly localized in the cytoplasm. When activated, partially activated JNK translocates into the nucleus to activate intranuclear transcription factors, such as c-JUN, ATF2, or p53, by phosphorylation [Citation28–Citation30], thus, enhancing the expression of downstream apoptosis-related genes and promoting apoptosis. The results of the present study showed that Tau could promote the expression of JNK and its phosphorylation in Caco-2 cells, and increase apoptosis of CRC cells by activating the MST1-JNK pathway. When the activity of JNK was inhibited, Tau-induced apoptosis of CRC cells was alleviated. In addition, activated JNK can also phosphorylate many proteins of the BCL-2 family, inhibiting the anti-apoptotic activity of BCL-2 and BCL-XL, and promoting the activation of BAX, BIM, and PUMA, thus, leading to mitochondrial dysfunction, the release of cytochrome C, and apoptosis [Citation31]. Ura et al. [Citation32] reported that JNK not only participates in MST1-induced apoptosis, but can also regulate the activity of MST1 via feedback mechanism, resulting in promotion of apoptosis [Citation33]. Thus, MST1 activates the JNK signaling pathway when it promotes apoptosis and in turn, the JNK signaling pathway also plays an important role in the proapoptotic effect of MST1. Strano et al. [Citation34] considered that the occurrence of tumors is the consequence of multiple signaling pathways, among which the Hippo signaling pathway and the p53 apoptotic signaling pathway have certain functional connections. In adrenal pheochromocytoma PC12 cells, YAP is phosphorylated at serine 127, prompting YAP to enter the nucleus and act with protein p73 of the p53 family [Citation35]. p73 then activates the apoptosis-related protein, PUMA, in the cytoplasm in a transcription-dependent manner, which, in turn, promotes the release of mitochondrial cytochrome C, leadingto apoptosis [Citation36]. Therefore, we hypothesized that the Tau-induced apoptosis of CRC cells might be the consequence of the combination of effects mediated by the MST1-JNK signaling pathway, the MST1-Hippo pathway, the p53 pathway, and the PUMA-mediated mitochondrial apoptotic signaling pathway. However, this study did not specifically investigate all these aspects, and further studies are underway to test this hypothesis.
Acknowledgments
This study was funded by the National Natural Science Foundation of China (No. 81360032); the Natural Science Foundation of Jiangxi (No. 20161BAB205206).
Disclosure statement
No potential conflict of interest was reported by the authors.
Additional information
Funding
References
- Torre LA, Bray F, Siegel RL, et al. Global cancer statistics. CA Cancer J Clin. 2015;65(2):1–12.
- Song H, Mak KK, Topol L, et al. Mammalian MST1 and MST2 kinases play essential roles in organ size control and tumor suppression. Proc Natl Acad Sci USA. 2010;107(4):1431–1436.
- Qin F, Tian J, Zhou D, et al. MST1 and MST2 kinases: regulations and diseases. Cell Biosci. 2013;3(1):31.
- Liang K, Zhou G, Zhang Q, et al. Expression of hippo pathway in colorectal cancer. Saudi J Gastroenterol. 2014;20(3):188–194.
- Zhou GX, Li XY, Zhang Q, et al. Effects of the Hippo signaling pathway in human gastric cancer. Asian Pac J Cancer Prev. 2013;14(9):5199–5205.
- Wang Z, Tang B, Tang F, et al. Protection of rat intestinal epithelial cells from ischemia/reperfusion injury by (D Ala2, D Leu5) enkephalin through inhibition of the MKK7 JNK signaling pathway. Mol Med Rep. 2015;12(3):4079–4088.
- Yanga SJ, Choib JM, Parkc SE, et al. Preventive effects of bitter melon (Momordica charantia) against insulin resistance and diabetes are associated with the inhibition of NF-κB and JNK pathways in high-fat-fed OLETF rats. J Nutr Biochem. 2015;26(3):234–240.
- Lou G, Dong X, Xia C, et al. Direct targeting sperm-associated antigen 9 by miR-141 influences hepatocellular carcinoma cell growth and metastasis via JNK pathway. J ExpClinCancer Res. 2016;35:14.
- Yousef HN, Aboelwafa HR. The potential protective role of taurine against 5-fluorouracil-induced nephrotoxicity in adult male rats. Exp Toxicol Pathol. 2017;69(5):265–274.
- Zhang X, Tu S, Wang Y, et al. The mechanism of taurine-induced apoptosis in human colon cancer cells. Acta Biochim Biophys Sin (Shanghai). 2014;46(4):261–272.
- Vanitha MK, Priya KD, Baskaran K, et al. Taurine regulates mitochondrial function during 7,12-dimethyl benz[a]anthracene induced experimental mammary carcinogenesis. J Pharmacopuncture. 2015;18(3):68–74.
- Tu S, Zhang X, Luo D, et al. Effect of taurine on proliferation and apoptosis of human hepatocellular carcinoma (HHCC) HepG2 cells. Exp Ther Med. 2015;10(1):193–200.
- Zhang X, Lu H, Wang Y, et al. Taurine induces apoptosis of breast cancer cells by regulating apoptosis-related proteins of mitochondria. Int J Mol Med. 2015;35(1):218–226.
- Choi EJ, Tang Y, Lee CB, et al. Effect of taurineon in vitro Migration of MCF-7 and MDA-MB-231 Human Breast Carcinoma Cells. Adv Exp Med Biol. 2015;803:191–201.
- Li Y, Hu Z, Chen B, et al. Taurineattenuates methamphetamine-inducedautophagy and apoptosis in PC12 cells through mTOR signaling pathway. Toxicol Lett. 2012 23;215(1):1–7.
- Tang F, Zhang L, Xue G, et al. hMOB3 modulates MST1 apoptotic signaling and supports tumor growth in glioblastoma multiforme. Cancer Res. 2014;74(14):3779–3789.
- Prause M, Mayer CM, Brorsson C, et al. JNK1 deficient insulin-producing cells are protected against interleukin-1 β-Induced apoptosis associated with abrogated myc expression. J Diabet Res. 2016;2016:1312705.
- Schaffer SW, Jong CJ, Ito T, et al. Effect of taurineon ischemia-reperfusion injury. Amino Acids. 2014;46(1):21–30.
- Marcinkiewicz J, Kontny E. Taurine and inflammatory diseases. Amino Acids. 2014;46(1):7–20.
- Huang S, Chong N, Lewis NE, et al. Novel personalized pathway-based metabolomics models reveal key metabolic pathways for breast cancer diagnosis. Genome Med. 2016;8(1):34.
- Zawaski JA, Sabek OM, Voicu H, et al. Effect of brain tumor presence during radiation on tissue toxicity: transcriptomic and metabolic changes. Int J Radiat Oncol Biol Phys. 2017;99(4):983–993.
- Sørensen BH, Thorsteinsdottir UA, Lambert IH. Acquired cisplatin resistance in human ovarian A2780 cancer cells correlates with shift in taurine homeostasis and ability to volume regulate. Am J Physiol Cell Physiol. 2014;307(12):C1071–1080.
- Wierzbicki PM, Rybarczyk A. The Hippo pathway in colorectalcancer. Folia Histochem Cytobiol. 2015;53(2):105–119.
- Konsavage WM, Kyler SL, Rennoll SA, et al. Wnt/beta-catenin signaling regulates Yes-associated protein (YAP) gene expression in colorectal carcinoma cells. J Biol Chem. 2012;287(15):11730–11739.
- Wang W, Cheng J, Zhu Y. The JNK signaling pathway is a novel molecular target for S-propargyl-L-cysteine, a naturally-occurring garlic derivatives: link to its anticancer activity in pancreatic cancer in vitro and in vivo. Curr Cancer Drug Targets. 2015;15(7):613–623.
- Ocuin LM, Zeng S, Cavnar MJ, et al. Nilotinib protects the murine liver from ischemia/reperfusion injury. J Hepatol. 2012;57(4):766–773.
- Lin MH, Yen JH, Weng CY, et al. Lipid peroxidation end product 4-hydroxy-trans-2-nonenal triggers unfolded protein response and heme oxygenase-1 expression in PC12 cells: roles of ROS and MAPK pathways. Toxicology. 2014;315:24–37.
- Saha MN, Jiang H, Yang Y, et al. Targeting p53 via JNK pathway: a novel role of RITA for apoptotic signaling in multiple myeloma. PLoS One. 2012;7(1):e30215.
- Kim CG, Choi BH, Son SW, et al. Tamoxifen-induced activation of p21 Waf1/Cip1 gene transcription is mediated by early growth response-1 protein through the JNK and p38 MAP kinase/Elk-1 cascades in MDA-MB-361 breast carcinoma cells. Cell Signal. 2007;19(6):1290–1300.
- Gozdecka M, Lyons S, Kondo S, et al. JNK suppresses tumor formation via a gene-expression program mediated by ATF2. Cell Rep. 2014;9(4):1361–1374.
- Wei Y, Sinha S, Levine B. Dual role of JNK1-mediated phosphorylation of Bcl-2 in autophagy and apoptosis regulation. Autophagy. 2008;4(7):949–951.
- Ura S, Nishina H, Gotoh Y, et al. Activation of the c-Jun N-terminal kinase pathway by MST1 is essential and sufficient for the induction of chromatin condensation during apoptosis. Mol Cell Biol. 2007;27(15):5514–5522.
- Bi W, Xiao L, Jia Y, et al. c-Jun N-terminal kinase enhances MST1-mediated pro-apoptotic signaling through phosphorylation at serine 82. J Biol Chem. 2010;285(9):6259–6264.
- Strano S, Fausti F, Di Agostino S, et al. PML surfs into HIPPO tumor suppressor pathway. Front Oncol. 2013;3:36.
- Zhang H, Wu S, Xing D. Inhibition of A beta (25–35)-induced cell apoptosis by low-power-laser-irradiation (LPLI) through promoting Akt-dependent YAP cytoplasmic translocation. Cell Signal. 2012;24(1):224–232.
- Yoon MK, Ha JH, Li MS, et al. Structure and apoptotic function of p73. BMB Rep. 2015;48(2):81–90.