ABSTRACT
Quercetin (QN) is a naturally occurring phenolic compound found largely in vegetables and fruits. Lycopene (LY) is yet another natural phytocompound, found abundantly in red-colored fruits and vegetables. Both have been reported to have beneficial activities in humans. In this study, we document in vivo experimental model for isoproterenol (ISO) cardiac injury toxicity in Sprague-Dawley (SD) rats and treatment with a combined optimized concentration of quercetin and lycopene (QL). Male SD rats of different groups were treated with QL (80 mg/kg QN and 3 mg/kg LY together p.o.) for 10 days with ISO administration (100 mg/kg i.p.) on days 7 and 8. After experimental period, CK-MB, TROP, AST, ALT, LDH, GST, GPx, CAT, SOD, Vit.E, Vit. C, GSH, GSSG and MDA were estimated. SD rats administered with ISO showed an obvious rise in the serum marker enzyme levels and tissue oxidative stress markers (MDA and GSSG). Furthermore, marked reductions in the body weight and increases enzymic and non-enzymic antioxidant levels were noticed. Histological features of the heart also indicated a disruption in the cardiac myofibrils structure of ISO-intoxicated rats. Also, quantitative PCR analysis revealed an involvement of antioxidant and related pathway genes such as Nrf2, HO-1, NQO1, GSTµ, SOD1, SOD2, CAT and BCl-2 genes. QL pretreatment prevented all these adverse effects of ISO cardiotoxicity and significantly reduced the myocardial damage. Decrease in oxidative stress was observed, possibly through alterations in the expression levels of enzymic antioxidant genes (GSTµ, SOD1, SOD2 and CAT). In general, QL exert a strong protective effect through the modulations in enzymic antioxidant activity and associated molecular pathways-regulating effect in cardiovascular disease.
1. Introduction
Cardiovascular disease (CVD) is a group of disease that involves blood vessels of heart, brain, arms and legs. People with CVD are presented with various forms of complications such as atherosclerosis, heart failure and stroke. Normally, both heart failure and strokes are caused by a blockage of fatty deposits on the inner walls of blood vessels that supply blood to the heart or brain. Such blockage is associated with numerous behavioral activity such as unhealthy diet, lack of exercise, excessive alcohol and tobacco use as well as history of diabetic and hypertension (Turer, A.T. and Hill, J.A. Citation2010). Often, many alarming warnings of CVD are prominent, which includes numbness or pain in face and chest area, shortened breath, nausea, breaking into a cold sweat and loss of coordination. Manifestation of untreated CVD complications may lead to lethal outcomes in myocardial infarction (MI). Myocardial ischemia (MI) refers to an exacerbation of cardiac tissue injury due to decreased oxygen supply and/or decreased blood supply to cardiac tissues (Kocak et al., Citation2016).
World Health Organization (WHO) reported that MI is expected to be the main reason of mortality, with fatality rate increasing to 20 million cases. At present, 130 million people are living with CVD with more cases in males (68%) than in females and (32%). Roughly, three quarters of CVD deaths are from low- and middle-income countries such as Indonesia, Pakistan, Philippines and Bangladesh (Fajobi et al., Citation2020). Most people from low- and middle-income countries have restricted access to health care treatments to respond to their needs. As a result, people with risk factors are diagnosed in the later stage and die younger due to CVDs. The average age of death caused by CVD in low- and middle-income countries is around 40 years while it is around 80 years in developed countries. However, a rapid rise in CVD incidence and prevalence across developed countries highlight the specific characteristic of Westernization phase, addressing an alarming concern in the management of CVD despite rapid advancements in the health care services (Ricardo et al., Citation2019).
Progression of the incidence, associating disability and the intimidating health quality with increasing mortality rate in both developing and developed countries, instigated a substantial interest in research of therapeutic management with a suitable compound for regulating CVD in terms of the drug efficiency and safety. Though certain drugs prevent CVD, these drugs are either not very effective in combating disease or associated with severe side effects. Studies have suggested that inflammation plays an important role in CVD pathogenesis (Pantazi et al., Citation2016). An increased inflammatory response results in series of abnormal metabolic and biochemical fluctuations in cardiac and brain cell. The prolonged oxygen deprivation leads to lactic acid accumulation and reduction in the intracellular pH. This acidic conditions lead to stimulation of reactive oxygen species (ROS). Accumulation of ROS subsequently activates inflammatory response which further elevates the contractile dysfunction, hypertrophy, fibrosis and cell death. These abnormalities may conjointly react to severely ignite the whole process of CVD (Tsutsui et al., Citation2011).
A combination of antioxidants and anti-inflammatory actions can avert these detrimental events and mitigate CVD and its associated complications. With this theory, it was proposed that use of some bioactive compounds from natural products may defend an individual from CVD. Quercetin (QN) and lycopene (LY) are natural antioxidants found mainly in fruits and vegetables, in combination has unique biological properties such as anti-inflammatory, anti-hypertensive, anti-oxidant and anti-cancer (Twinkle et al., Citation2019). Though both QN and LY have a remarkable pre-clinical standard in pharmacology field, strategies aimed at inhibiting the inflammatory response in combination of QN and LY (QL) have not been explored yet against CVD. In addition, improvement of CVD through therapeutic administration of QL could be a promising approach against CVD. With this information, in the present study, an in vivo experiment was performed to evaluate cardioprotective efficacy of QL against isoproterenol (ISO) induced cardiotoxicity in rats.
2. Material and methods
2.1. Chemicals and reagents
QN and LY of analytical grade were purchased for the present investigation and were prepared in 80% ethanol. The following chemicals were procured from Sigma-Aldrich: ISO was dissolved in phosphate buffer saline (PBS), 2,3,5-triphenyltetrazolium chloride (TTC) staining solution, sodium dodecyl sulphate (SDS), 1-chloro, 2,4-dinitrobenzene (CDNB), meta-phosphoric acid (MPA), fluoromethane sulfonate (M2VP), hematoxylin and eosin (H&E) staining solution. All other chemicals used in the experiment were of analytical grade.
3. Experimental protocol
3.1. Animal model
A total of 32 Sprague-Dawley rats (7–9 weeks; 170–190 g) free from any disease were caged under controlled laboratory environment and allowed freely to drinking water and rodent pellets. Acclimatization to experimental condition was performed for seven days in animal house prior to commencement of the experiments. All further protocols were performed to minimize suffering. Experimental protocol was designed according to the international guidelines and was approved by the Institutional Animal Ethics Committee (IAEC).
4. Experimental design and drug administration
SD rats were grouped into four groups with eight animals each (n = 8). Effective dose of QL was fixed based on the activities of serum creatine kinase-MB (CK-MB) in the preliminary study for dosage optimization (data not shown) and it was 80 mg/kg QN together with 3 mg/kg LY. Rats in group I (control) received 0.1% NaCl throughout the experimentation (10 days). Rats in group II received oral administration of QL for ten days. On the sixth and seventh day, the rats in group III and IV were intraperitoneally administered with isoproterenol (100 mg/kg b.w.). Also, group IV rats were administered QL simultaneously as in group II. Following were the assigned group information:
Group 1: Control (0.1% NaCl)
Group II: QL (Quercetin [QN] 80 mg/kg and lycopene [LY] 3 mg/kg p.o.)
Group III: Isoproterenol (ISO; 100 mg/kg i.p.)
Group IV: ISO + QL (as in group III and group II)
Body weights of the rats were recorded daily until end of the experimentation. On tenth day, all animals were sacrificed by cervical decapitation under anesthesia with pentobarbital sodium (40 mg/kg). Blood samples and heart tissues were quickly prepared for various analyses.
5. Assessment of organ indexes (heart weight to body weight) in experimental rats
Heart tissue samples were washed with ice-cold potassium chloride (KCl) and were blotted with filter paper for complete dryness and weighed. Organ indexes (heart weight to body weight) were calculated using these values.
6. Assessment of serum biochemical markers in experimental rats
Following decapitation, blood samples were collected for serum samples preparation by centrifugation. Activities of serum cardiac marker enzyme (CK-MB), cardiac troponin T (TROP) and lactate dehydrogenase (LDH) were determined by the methods of Okinaka et al. [Citation1957]. Activities of serum aspartate transaminase (AST) and alanine aminotransferase (ALT) were done by the method of Reitman and Frankel [Citation1961]. All the analyses were carried out using assay kits and as instructed in the manufacturer’s protocol. Absorbance values were recorded using microplate reader. Total protein in the samples was determined by the method of Lowry et al. [Citation1951].
7. Assessment of oxidative stress markers using serum and heart tissue homogenate in experimental rats
MDA:
Malondialdehyde (MDA) content was analyzed for thiobarbituric acid reactive substance (TBARS) products in serum and heart tissue homogenate. Preparation of heart tissue homogenates was done by homogenizing each heart sample and then centrifuged at low speed for 30 mins. For TBARS, recovered supernatants and serum were added with 0.1 mL of 8.1% SDS, 1 mL of 20% acetic acid, and 1 mL of 8% TBA. Reaction mixture was heated for an hour. Following incubation, tubes were cooled and further centrifuged for 15 mins. Supernatant was used for the absorbance measurement at a wavelength of 532 nm. TBARS levels were expressed as nmol of MDA per liter in serum and nmol of MDA per gram in heart tissue.
GSSG:
GSSG level was determined according to the method described by Li et al. [Citation2012] with a modification. For determination of GSSG, test sample was mixed with MPA and M2VP for further oxidation. Sample mixture’s absorbance values were determined at 370 nm and expressed as nmol/g tissue.
8. Assessment of myoglobin (MYO) concentration in experimental rats
For determination of MYO concentration, heart samples were first fixed in paraformaldehyde and subsequently in glutaraldehyde solution for 15 mins. Then, the samples were incubated for 1 h in Tris/80 mM KCl buffer, O-toluidine, 90% ethanol and 70% tertiary-butyl-hydroperoxide at 50°C. Following, absorbance was measured at 436 nm and expressed in µg/L.
9. Assessment of enzymic antioxidants and non-enzymic antioxidants in experimental rats
Supernatants of tissue homogenate were used for the determinations of activities of antioxidant enzymes.
Superoxide dismutase (SOD):
SOD value was measured according to the method of Marklund and Marklund [Citation1974]. Principally, SOD concentration was determined on its capability to prevent the superoxide-mediated reduction. One unit of SOD was assumed as enzyme activity to inhibit 50% of pyrogallol oxidation. Test sample was mixed with prepared solution and buffer. Mixture was mixed well before incubation at 37°C for 20 minutes. Absorbance was recorded at 450 nm and SOD activity was expressed as U/mg of protein.
Catalase (CAT):
Catalase (CAT) activity was determined by the method of Aebi [Citation1983]. Test sample was incubated with substrate (H2O2 and NaCl) at 37°C for a minute. Enzymatic reaction was halted upon formation of yellow complex in the solution and absorbance was measured at 405 nm. One unit of CAT was defined as the enzyme activity essential to decompose 1 μM of H2O2 per min. CAT enzyme activity was expressed as µM of H2O2 decomposed per min per mg of protein.
Glutathione Peroxidase (GPx):
Glutathione peroxidase (GPx) activity was completed according to the method described by Flohe and Gunzler [Citation1984]. Supernatant was mixed with PBS, GSH, sodium azide and H2O2 to make 1 mL of reaction mixture. Following incubation for 15 min at 37°C, the reaction was arrested by adding 5% TCA. Tubes were centrifuged at 1000 g for 8 min and the resulting supernatant was obtained. Absorbance was measured at 420 nm and the GPx activity was expressed as µM of GSH oxidized per min per mg of protein.
Glutathione-S-transferase (GST):
Total GST activity was measured according to the method of Habig et al. [Citation1974]. Test sample was mixed with 1-chloro-2,4-dinitrobenzene (CDNB) and treated with 10 nM TCDD for 24 h. Conjugation of CDNB was measured at 340 nm and the activity was expressed in nM of CDNB conjugated per min per mg of protein.
Reduced glutathione (GSH):
Levels of GSH was analyzed by the method of Aebi [Citation1983]. Test sample was diluted with phosphate-EDTA buffer and incubated at room temperature for 5 minutes. After constant mixing and incubation for 15 min at room temperature, the solution was measured at 350 nm and expressed as mg/dl in serum and µmol/g in tissues.
Vitamin E and vitamin C:
Levels of vitamin E and vitamin C were determined by the method of Quaife et al. [Citation1949] using the colorimetric principle. Assay based on the decrease in concentration of tocopherols due to the conversion of ferric into ferrous ions (red complex). Test sample was added with ethanol and mixed vigorously. Then, the mixture was mixed with xylene and protected from light. After centrifugation for 30 minutes, ferric chloride solution was added. After complete mixing, absorbance was recorded at 562 nm and expressed as µmol per liter for both vitamins.
10. Assessment of histopathological injury in experimental rats
Heart tissue section was immersed in 10% buffered formalin for two days and then serially dehydrated in series of graded ethanol. After further dehydration with formalin, tissues were entrenched in paraffin. Using microtome, tissue section of 5 µm thickness was sliced and stained with H&E for analysis using a light microscope. Tissue sections were imaged at 40X magnification to assess the histopathological changes.
11. Quantitative PCR in experimental rats
Nrf2/HO-1 gene and its associated gene expression levels such as Nrf2, HO-1, BCl-2, GSTµ, SOD1, SOD2, CAT and NQO1 were investigated using RT-PCR. Total RNA from tissue homogenate was extracted using TRIZOL reagent followed by DNase-I treatment to remove traces of DNA as per the manufacturer’s manual. Purity of the extracted RNA was determined by a spectrophotometer at 260 nm and stored at –70°C until further use. One μg of total RNA was used to synthesize cDNA using commercial cDNA synthesis kit. Expressions of all the genes were performed using a Maxima SYBR green qPCR master mix. Data were screened and normalization was done against GAPDH gene. Fold change was calculated using the 2–ΔΔCT method for each gene. Primers used were listed below:
12. Statistical analysis
Data were presented as mean ± SD for each group and was calculated using SPSS 17.0 software package. Level of significance was calculated by Student’s t-test with appropriate post-hoc analysis. A P ≤ 0.05 was considered as statistically significant.
13. Results
13.1. Effect of QL on the heart weight to body weight ratio in experimental rats
Ratio of the heart weight to body weight (Hw/Bw) is shown in . As depicted, ratio of Hw/Bw was comparable in all experimental groups. No significant alterations were noticed in the Hw/Bw ratio between group I (control) and group II (QL). However, a significant decrease (P < 0.05) in the Hw/Bw ratio in group III (ISO) was noticed in comparison to group I. Upon treatment with QL (group IV) in ISO intoxicated rats, a significant Hw/Bw ratio recovery was noticed. This confirmed a preliminary cardioprotective effect of QL in ISO toxicities in rats.
Table 1. Effects of ISO and treatment with QL on heart weight/body weight in experimental rats
14. Effect of QL on the cardiac biomarkers in experimental rats
Serum cardiac marker levels such as creatine kinase-MB (CK-MB) and cardiac troponin T (TROP) were summarized in and respectively. QL group showed almost normal levels of both cardiac marker enzymes (CK-MB and TROP) when compared with group I (control) rats. Group III (ISO) showed a significant (p < 0.05) increase in CK-MB and TROP levels as compared with group I. Treatment with QL (group IV) during ISO intoxication decreased the elevated CK-MB and TROP levels to near normal. This result revealed the potential ameliorative effect of QL in ISO toxicities in rats.
Figure 1. (A-D): Effects of isoproterenol (ISO) and treatment with quercetin-lycopene (QL) on the marker enzymes creatine kinase-MB (CK-MB), aspartate transaminase (AST), alanine transaminase (ALT) and lactate dehydrogenase (LDH). Data presented were of mean±SD. Results were significant at P ≤ 0.05 in comparison with control group (*) and with ISO group (#)
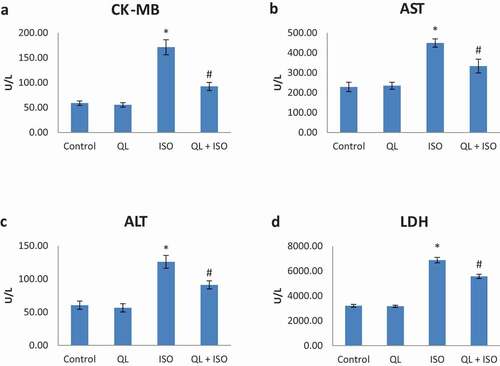
15. Effect of QL on marker enzymes in experimental rats
Oral administration of QL on marker enzymes in ISO induced rats was shown in . Administration of QL alone (group II) showed no significant alteration on marker enzymes (ALT and AST) in serum when compared with group I (control) rats. Intoxication of ISO in group III causes a significant (P < 0.05) elevation of ALT and AST levels when compared with control group of rats. QL treatment (group IV) significantly prevented the elevation of serum activities of ALT and AST that depicted the cardioprotection of QL in ISO intoxicated rats.
16. Effect of QL on serum LDH activity in experimental rats
Activities of LDH in experimental rats were shown in . Results signify that the administration of QL alone in group II did not caused any alterations in the activities of serum LDH compared with group I (control) rats. ISO intoxication in rats showed a significant elevation (P < 0.05) in LDH activities (group III) and treatment with QL (group IV) significantly controlled the elevation. These results indicated that the cardiotoxicity produced by ISO was efficiently nullified with QL treatment.
17. Effect of QL on MYO level in experimental rats
Alterations of MYO level in the serum of normal and experimental rats were shown in . Rats intoxicated with ISO (group III) presented a significant increase (p < 0.05) in the level of MYO compared to the control (group I) rats. QL treatment significantly reversed the abnormal levels of MYO in group IV rats. Hence ISO induced myoglobin abnormality towards cardiac injury was effectively suppressed by QL treatment.
18. Effect of QL on oxidative stress markers in experimental rats
Lipid peroxidation alterations in heart tissue and serum of normal and experimental rats were shown in and respectively. Rats induced with ISO (group III) presented a significant increase (p < 0.05) in the levels of MDA and GSSG when compared to the control (group I) rats. No significant alterations in these levels were noticed with QL alone administration. QL treatment significantly reversed the elevated levels of lipid peroxidation products in ISO intoxication in group IV. Hence, lipid peroxidation products were significantly suppressed during QL treatment.
Table 2. Effects of ISO and treatment with QL on oxidative stress level in the heart of experimental rats
19. Effect of QL on enzymic and non-enzymic antioxidants in experimental rats
Levels of non-enzymic antioxidant (GSH, Vit-C and Vit-E) and antioxidant enzyme (SOD, CAT, GPx and GST) activities of experimental rats were depicted in respectively. All were significantly reduced (P < 0.05) in ISO intoxicated animals (group III) with significant normalization during QL treatment in group IV. QL administration alone showed no significant alterations. From these results, it was evident that QL was effective in improving the tissue antioxidant status against ISO intoxication in rats.
Table 3. Effects of ISO and treatment with QL on non-enzymic anti-oxidant levels
Table 4. Effects of ISO and treatment with QL on enzymic antioxidant levels
20. Effect of QL on histopathological injuries in experimental rats
Evidence of cardiac injury was depicted via histopathological studies in . Histopathological results of the control (group I) rats and QL alone administered (group II) showed normal cardiac tissue architecture. Following ISO intoxication in group III rats, area of infarction with intense damage in cardiac tissues and increased inflammatory spots were identified. Treatment with QL in group IV rats showed a significant decrease in the degree of the histopathological damage as compared to ISO intoxicated (group III) rats. This result showed that QL proved a significant protection against ISO-induced cardiac injuries in rats.
21. Effect of QL on gene expressions in experimental rats
Expression of genes (Nrf2, HO-1, BCL-2, GSTµ, SOD1, SOD2, CAT and NQO1) in control and experimental rats are shown in . Data depicted no alterations in Nrf2, HO-1, GSTµ, SOD1, SOD2, CAT, NQO1 and BCl-2 mRNA expression levels of group II when compared with control (group I) rats. However, there was a significant decrease in the entire target mRNA levels in ISO intoxicated rats (group III). Remarkably, QL treatment in ISO intoxicated rats (group IV) restored the activities of GSTµ, SOD1, SOD2, CAT and BCL2 genes. However a noticeable increase in the expression levels of Nrf2, HO-1 and NQO1 were noticed without significance. These results suggest that QL treatment could significantly modify the expression levels of enzymic antioxidants through up-regulation of its mRNA levels.
Figure 4. Effects of isoproterenol (ISO) and treatment with quercetin-lycopene (QL) on Nrf2/HO-1 signaling gene network. Data presented were of mean±SD of triplicate measurements. Statistical significant level was calculated by ANOVA and post-hoc by DMRT analysis. Results were significant at P ≤ 0.05 in comparison with control group (*) and with ISO group (#)
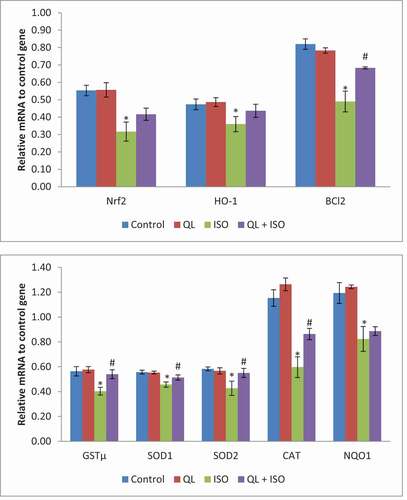
22. Discussion
Isoproterenol (ISO) is a potent β-adrenergic receptor agonist, metabolized in human body by an enzyme known as catechol-O-methyl transferase. In higher dose, metabolic products of ISO causes cardiac toxicity by producing excessive amount of free radicals, increased intracellular Ca2+, mitochondrial dysfunction due to insufficient blood supply, and eventually leading to severe cardiac infarction and necrotic damage (Wong et al., Citation2017). Higher dose of ISO intoxication animal models depict the injuries similar to ischemic cardiovascular disease in humans. Hence, ISO was used by many researchers as a preferred model of ischemic cardiovascular disease to explore the effects of various treatment drugs. ISO rat model have advantages of higher efficiency, consistency, reduced mortality and morbidity rate during the experimental period (Brindha and Rajasekapandiyan, Citation2015). ISO intoxication showed a significant elevation on Hw/Bw ratio that could be due to accumulation of water in edematous intramuscular space and inflammatory cells infiltration to damaged areas in the cardiac tissue (Ibrahim et al., Citation2015). Previous reports documented that 10% of cardiac function could be impaired with a 1% increase in water content and ISO cardiotoxicity could alter the cardiac membrane permeability, which is the key contributing factors to cardiac injury (Ibrahim et al., Citation2015). In the present study, improvement in the Hw/Bw might be due to the control of water accumulation and alteration in the cardiac membrane permeability, thereby leading to prevention of cardiac damage.
Exposure to toxic agents such as ISO or other synthetic drugs could elicit pathological changes causing membrane leakage of marker enzymes, ALT and AST into bloodstream. Earlier studies documented that elevation in the activities of serum marker enzymes was an important indicator of tissue damage (Sidahmed et al., Citation2016; Boarescu et al., Citation2019). Increased activities of serum AST and ALT evidently confirmed the ISO cardiotoxicity and eventual decrease in the activities of these enzymes during QL treatment probably due to the protective effect of QL towards cardiomyocytes.
ISO cardiac injury could increase the cell membrane permeability of cardiac cells that could allow the leakage of cytosolic enzymes into the blood stream. CK-MB, LDH and TROP were the vital enzymes/proteins in heart that could support for ATP levels (a phosphate energy storage form), while MYO facilitates the gas exchange in the cardiac cells (Volker et al., Citation2010). Hence, cardiac cells become permeable and leakage to CK-MB, LDH, TROP and MYO, a valuable diagnostic tool for myocardial injury (Serra et al., Citation2010). In the present study, significant elevation of CK-MB, LDH, TROP and MYO in ISO intoxication indicated the damage/injury to the cardiac cells. However, treatment with QL decreased the elevation of these parameters in serum, probably by maintaining the integrity of the cardiac cellular membrane against ISO injuries. Hence, it was reasoned that the presence of QL might exert protection against cardiac cell injury and avoided the leakage from the cardiac cells.
Excessive production of oxidative stress may lead to proteins and lipids oxidative damage that could interfere with normal functioning of the cardiac tissue. Consequently, it could affect the permeability of mitochondrial and cytoplasmic membranes and release oxidative products into circulation. TBARS is a well-established index of MDA content, an oxidative stress marker for lipid peroxidation in tissues (Kang et al., Citation2018). Also, GSSG, an important oxidative tripeptide thiol that depicts the oxidative stress status in various inflammation related disorders (Shahsavani et al., Citation2010). Augmented production of MDA and GSSG in ISO intoxication was efficiently controlled during treatment with QL led to significant cardioprotective efficiency. Therefore, it was convincing that constituents of QL inhibit and/or scavenge oxidative stress products related to ISO toxicity and exert protection against cardiac injuries.
Production of free radicals in ISO intoxication initiates oxidative stress, thereby damaging cardiac cellular architecture. Endogenous antioxidants defense systems disrupt the process of free radicals production within the cells and protect the cells from free‐radical assault (Rajesh et al., Citation2010). Hence, the balance between free radicals and antioxidant is vital, because an inequity would result in the free radicals stimulated toxic accumulation which obviously leads to cell damage (Prakash et al., Citation2013). Presence of enzymic and non-enzymic antioxidants provide endogenous defense against oxidants causing cardiac tissue injuries. As expected, activities of SOD, CAT, GST and GPx and levels of GSH, Vit-C and Vit-E depleted in ISO toxicity was a suggestive of oxidative stress in the cardiac tissue, which was efficiently normalized with QL treatment. Hence, these data backed the scavenging efficiency of QL in cardiac injuries. Also, it was justified with histopathological analysis that avoided the pathological architecture change of the cardiac tissues that supported the cardioprotective role of QL in oxidative stress induced cardiac injuries.
Nuclear factor erythroid 2-related factor 2 (Nrf2) was known as a vital transcription factor of heme oxygenase-1 (HO-1), where upregulation of HO-1 upon nuclear translocation was demonstrated to activate antioxidant genes (GST, SOD1, SOD2, CAT, NQO1) against oxidative stress (Lim et al., Citation2015). In addition, cardiovascular disease demonstrated a loss of cardiomyocyte through apoptosis pathway. Hence, in the process of apoptosis, BCl-2 plays an important role to prevent cell death by maintaining the integrity of the mitochondrial membrane, which is essential in averting intrinsic apoptotic pathway (Khadrawy et al., Citation2019). Consistent with the report, ISO intoxication significantly decreased the expression of Nrf2, HO-1, BCl-2, GSTµ, SOD1, SOD2, CAT and NQO1 and significant restoration of BCl-2, GSTµ, SOD1, SOD2 and CAT gene expressions during QL treatment. However, a non-significant increase in the expressions of Nrf2, HO-1 and NQO1 was also considered worth in depicting the protective efficacy of QL. Consistent with our reports, study conducted by Jeong et al., Citation2019, demonstrated similar protective effect of baicalein towards Nrf2/HO-1 signaling against oxidative stress-induced DNA damage in HEI193 Schwann cells. To summarize, the cardioprotective effect of QL was attributed to its efficient activation of enzymic antioxidant gene expression pathways and its antioxidant activities.
23. Conclusion
In summary, this study reveals for the first time that the administration of QL combination was effective in ameliorating the extent of myocardial injuries and significantly counteracted the oxidative stress in ISO-induced cardiac damage in rats through activation of enzymic antioxidant defense gene expression pathways. Therefore, combination of quercetin and lycopene considered more advantageous and warrants more studies in the future, which can be developed as a potential natural cardioprotective agent in clinical practice.
Disclosure of potential conflicts of interest
No potential conflict of interest was reported by the author(s).
References
- Turer AT, Hill JA. Pathogenesis of myocardial ischemia-reperfusion injury and rationale for therapy. Am J Cardiol. 2010;106(3):360–10.
- Kocak C, Kocak FE, Akcilar R, et al. Molecular and biochemical evidence on the protective effects of embelin and carnosic acid in isoproterenol-induced acute myocardial injury in rats. Life Sci. 2016;147:15–23.
- Fajobi AO, Okon BOE, Oyedapo OO, et al. Cardioprotective activities of Pterocarpus mildbraedii leaves on isoproterenol-induced myocardial infarction in rats. Eur J Med Plants. 2020;31:20–37.
- Ricardo OS, Soares DM, Losada MC, et al. Ischemia/reperfusion injury revisited: an overview of the latest pharmacological strategies. Int J Mol Sci. 2019;20(20):5034.
- Pantazi E, Bejaoui M, Folch-Puy E, et al. Advances in treatment strategies for ischemia reperfusion injury. Expert Opin Pharmacother. 2016;17(2):169–179.
- Tsutsui H, Kinugawa S, Matsushima S. Oxidative stress and heart failure. Am J Physiol Heart Circ. 2011;301(6):2181–2190.
- Twinkle F, Vishnu P, Gayathri R. A comparative study on the efficacy of lycopene and quercetin in oral submucous fibrosis cell lines. Drug Invent Today. 2019;12:1345–1351.
- Li Y, Wende AR, Nunthakungwan O, et al. Cytosolic, but not mitochondrial, oxidative stress is a likely contributor to cardiac hypertrophy resulting from cardiac specific GLUT4 deletion in mice. FEBS J. 2012;279(4):599–611.
- Reitman S, Frankel S. A colorimetric method for the determination of serum glutamate oxaloacetic and glutamate pyruvic transaminases. Am J Clin Pathol. 1961;28(1):56–63.
- Okinaka S, Kumagai H, Ebashi S. Serum creatine phosphokinase: activity in progressive muscular dystrophy and neuromuscular diseases. Arch Neurol. 1957;4(5):520–525.
- Lowry OH, Rosebrough NJ, Farr AL, et al. Protein measurement with the folin-phenol reagent. J Biol Chem. 1951;193(1):265–275.
- Marklund S, Marklund G. Involvement of the superoxide anion radical in the autoxidation of pyrogallol and a convenient assay for superoxide dismutase. Eur J Biochem. 1974;47(3):469–474.
- Aebi HE. Catalase. In: Bergmeyer HU, editor. Methods of enzymatic analysis. Vol. 3. Weinheim Germany: Verlag Chemie; 1983. p. 273–286.
- Flohe L, Gunzler WA. Assays of glutathione peroxidase. Meth Enzymol. 1984;105:114–120.
- Habig WH, Pabst MJ, Jakoby WB. Glutathione S-transferases. The first enzymatic step in mercapturic acid formation. J Biol Chem. 1974;249(22):7130–7139.
- Quaife ML, Dju MY. Chemical estimation of vitamin E in tissue and the tocopherol content of some normal human tissues. J Biol Chem. 1949;180(1):263–272.
- Wong ZW, Thanikachalam PV, Ramamurthy S. Molecular understanding of the protective role of natural products on isoproterenol‐induced myocardial infarction: a review. Biomed Pharmacother. 2017;94:1145–1166.
- Brindha E, Rajasekapandiyan M. Preventive effect of phytic acid on isoproterenol-induced cardiotoxicity in wistar rats. Int J Biomed Sci. 2015;11:35‐41.
- Ibrahim KM, Istiyak A, Romana A, et al. Amelioration of isoproterenol-induced oxidative damage in rat myocardium by Withania somnifera leaf extract. Biomed Res Int. 2015;624159.
- Volker E, Gerolf G, Klaus DJ. Significance of myoglobin as an oxygen store and oxygen transporter in the intermittently perfused human heart: a model study. Cardiovasc Res. 2010;87(1):22–29.
- Serra M, Papakonstantinou S, Adamcova M, et al. Veterinary and toxicological applications for the detection of cardiac injury using cardiac troponin. VET J. 2010;185(1):50–57.
- Sidahmed HM, Hashim NM, Mohan S, et al. Evidence of the gastroprotective and anti-Helicobacter pylori activities of β-mangostin isolated from Cratoxylumar borescens (vahl) blume. Drug Des Devel Ther. 2016;10:297–313.
- Boarescu PM, Chirila I, Bulboaca AE. Effects of curcumin nanoparticles in isoproterenol-induced myocardial infarction. Oxid med cell longev. 2019;7847142.
- Kang B, Wang X, Xu Q, et al. Effect of 3-nitropropionic acid inducing oxidative stress and apoptosis of granulosa cells in geese. Biosci Rep. 2018;38(5):208–274.
- Shahsavani D, Mohri M, Gholipour KH. Determination of normal values of some blood serum enzymes in Acipenser stellatus Pallas. Fish Physiology and Biochemistry. 2010;36(1):39–43.
- Rajesh M, Mukhopadhyay P, Batkai S, et al. Cannabidiol attenuates cardiac dysfunction, oxidative stress, fibrosis, and inflammatory and cell death signaling pathways in diabetic cardiomyopathy. J Am Coll Cardiol. 2010;56(25):2115–2125.
- Prakash O, Kumar A, Kumar P, et al. Anticancer potential of plants and natural products. Am J Pharm Sci. 2013;1:104–115.
- Jeong JY, Cha HJ, Choi EO, et al. Activation of the Nrf2/HO-1 signaling pathway contributes to the protective effects of baicalein against oxidative stress-induced DNA damage and apoptosis in HEI193 Schwann cells. Int J Med Sci. 2019;16(1):145–155.
- Lim J, Ortiz L, Nakamura BN, et al. Effects of deletion of the transcription factor Nrf2 and benzopyrene treatment on ovarian follicles and ovarian surface epithelial cells in mice. Reprod Toxicol. 2015;58:24–32.
- Khadrawy O, Gebremedhn S, Salilew-Wondim D, et al. Endogenous and exogenous modulation of Nrf2 mediated oxidative stress response in bovine granulosa cells: potential implication for ovarian function. Int J Mol Sci. 2019;20(7):1635.