ABSTRACT
Background: An alternative approach for managing Candida infections in the oral cavity by modulating the oral microbiota with probiotic bacteria has been proposed.
Objective: The aim was to investigate the antifungal potential of the probiotic bacterium Lactobacillus reuteri (DSM 17938 and ATCC PTA 5289) against six oral Candida species (C. albicans, C. glabrata, C. krusei, C. tropicalis, C. dubliniensis, and C. parapsilosis).
Design: The lactobacilli were tested for their ability to co-aggregate with and inhibit the growth of the yeasts assessed by spectrophotometry and the agar overlay inhibition assay. Additionally, the pH was evaluated with microsensors, and the production of hydrogen peroxide (H2O2) by the lactobacilli was verified.
Results: Both L. reuteri strains showed co-aggregation abilities with the yeasts. The lactobacilli almost completely inhibited the growth of C. albicans and C. parapsilosis, but did not affect C. krusei. Statistically significant differences in co-aggregation and growth inhibition capacities between the two L. reuteri strains were observed (p<0.001). The pH measurements suggested that C. krusei can resist the acids produced by the lactobacilli.
Conclusions: L. reuteri exhibited antifungal properties against five of the six most common oral Candida species. Further, the results reconfirms that the probiotic capacity of L. reuteri is strain specific.
Candida species are commensals in the oral cavity and part of the normal microbiota in 25–75% of healthy individuals [Citation1]. Nevertheless, Candida species are opportunistic pathogens which under certain circumstances cause infections in the oral mucosa, termed oral candidiasis. Infections are primarily caused by Candida albicans that has the ability to switch between the blastospore form and the more invasive hyphae form. Other Candida species have been isolated from infected sites in the oral cavity, including C. glabrata, C. krusei, C. tropicalis, C. dubliniensis, and C. parapsilosis [Citation2,Citation3]. Currently, an increase in fungal resistance to antimycotic therapy causes concern, and as a consequence an alternative bio-ecological approach of fungal management has been proposed [Citation4].
There is a growing interest in probiotic bacteria to prevent and combat oral diseases [Citation5]. Probiotic bacteria are defined by the World Health Organization as ‘Live microorganisms that, when administered in adequate amounts, confer a health benefit on the host’ [Citation6]. The most common probiotic genera Bifidobacterium and Lactobacillus are believed to act by competitive exclusion of pathogens from the oral mucosal adhesion sites, by competing for available nutrients, and by altering the mucosal immune host defence [Citation7,Citation8]. In addition, some probiotic bacteria produce acids from carbohydrate fermentation rendering a low pH, and some lactic acid bacteria produce hydrogen peroxide (H2O2) and bacteriocins which are harmful to pathogens [Citation5]. The probiotic Lactobacillus reuteri has been shown to survive the passage through the ventricle and to colonize the human gastrointestinal tract transiently [Citation9]. Under certain favourable conditions, L. reuteri produces a broad-spectrum antimicrobial substance, reuterin, assumed to inhibit DNA synthesis in pathogens in the surrounding environment [Citation10]. In a randomized placebo-controlled clinical trial, the authors’ research group has recently reported a significant reduction of the oral Candida load in a group of frail elderly persons after probiotic intervention with L. reuteri DSM 17938 and ATCC PTA 5289 in a combined lozenge [Citation11].
The ability of lactobacilli to co-aggregate with pathogens such as Candida is central in biofilm formation and is a desired feature of probiotics. Through co-aggregation, the lactobacilli may achieve an adequate mass and thereby have the ability to create a hostile micro-environment around the Candida species with high concentrations of substances such as acids, H2O2, bacteriocins, and so on that possibly inhibit the pathogens’ growth [Citation12]. In vitro studies have investigated co-aggregation and growth inhibition of probiotic and pathogenic bacteria [Citation12–Citation14], with the focus being on caries- and periodontitis-associated microorganisms [Citation13–Citation15]. For C. albicans, probiotic interference has been demonstrated with probiotic lactobacilli, for example L. rhamnosus GR-1, L. reuteri RC-14, and L. reuteri ATCC PTA 5289 [Citation16–Citation18]. To the authors’ knowledge, only a few studies have looked into the antifungal effect of probiotic lactobacilli on non-albicans Candida species commonly found in the oral cavity [Citation19–Citation21]. The aim of this study was therefore to investigate the in vitro abilities of two strains of the probiotic L. reuteri, with demonstrated ability to reduce the Candida load in vivo in a vulnerable population and to co-aggregate with and inhibit the growth of six opportunistic pathogenic oral Candida species. Additionally, the pH, as an expression of acid production by the lactobacilli, was evaluated through agar plates with Candida-Lactobacillus co-cultures. Moreover, the ability of the lactobacilli to produce H2O2 was verified.
Materials and methods
Strains and culture conditions
Two strains of the probiotic L. reuteri (DSM 17938 and ATCC PTA 5289; Biogaia, Stockholm, Sweden) were used in this study. The Candida strains used were six laboratory reference strains from the Culture Collection, University of Gothenburg, Sweden: C. albicans CCUG 46390; C. dubliniensis CCUG 48722; C. glabrata CCUG 63819; C. krusei CCUG 56126; C. parapsilosis CCUG 56136, and C. tropicalis CCUG 47037. In addition, six clinically isolated strains from humans were included, generously provided by the Department of Clinical Microbiology, Rigshospitalet, Copenhagen, Denmark. Prior to the in vitro studies, the clinical strains were characterized by matrix-assisted laser desorption/ionization time-of-flight (MALDI-TOF) mass spectrometry [Citation22] to confirm their identity as C. albicans CBS 562 NT, C. dubliniensis 41_3 ZZMK, C. glabrata CBS 863, C. krusei (Issatchenkia orientalis RV 491), C. parapsilosis 26 PBS, and C. tropicalis DSM 7524.
The lactobacilli were initially cultured on de Man Rogosa Sharpe (MRS) agar (Oxoid Ltd., Basingstoke, Hampshire, UK) for 24 h in an anaerobic chamber at 37°C (10% H2, 5% CO2, and 85% N2). The Candida strains were cultured on BD Difco™ Sabouraud Maltose (DSM) agar (Becton, Dickinson and Company, Sparks, MD) for 24 h in ambient air at 37°C.
Co-aggregation assay
The co-aggregation was determined spectrophotometrically (Genesys™ 10S UV-Vis Spectrophotometer; Thermo Fisher Scientific, Waltham, MA) and executed as described previously by Collado et al. [Citation12]. In brief, one distinct colony of overnight cultured lactobacilli was transferred to 5 mL MRS broth and incubated at 37°C for 24 h under anaerobic conditions. The Candida strains were similarly harvested and aerobically incubated in DSM broth at 37°C for 24 h. After incubation, the lactobacilli and Candida were harvested by centrifugation at 855 g (Sigma 2-6 Compact Centrifuge, Sigma, Poole, UK) for 10 min at room temperature, washed three times in phosphate-buffered saline (PBS), and resuspended in neutral 10 mmol/L PBS (pH 7.0). The absorbance was adjusted to an optical density (OD) of 0.5 at 600 nm (approximately 108 cfu/mL of the lactobacilli) by using the spectrophotometer to ensure identical densities at baseline. Equal volumes (1.0 mL) of the lactobacilli and Candida strains were mixed and incubated aerobically at 37°C for 1, 2, and 4 h without agitation, but were vortexed prior to each OD measurement. Co-aggregation was calculated by using the equation [Citation12,Citation15]:
where OD0 is the absorbance of the mixed suspension at baseline (0 h) and ODh is the absorbance of the mixed solutions at different time points (1, 2, and 4 h). The assays were carried out in duplicate and repeated four times on different occasions.
Agar overlay interference test
The growth inhibition assay was performed as described earlier [Citation14,Citation23]. In brief, one distinct colony of overnight cultured lactobacilli was transferred to 5 mL MRS broth and incubated at 37°C for 24 h under anaerobic conditions. The following day, the lactobacilli were harvested by centrifugation at 855 g for 10 min at room temperature. The supernatants of the two Lactobacillus strains were obtained after centrifugation and filter sterilized by the aid of sterile 20 mL syringes (Omnifix®; B. Braun, Melsungen, Germany) and syringe filters, 0.8/0.2 µm pore size membranes (Acrodisc® PF Syringe Filter; Pall Life Sciences, Lund, Sweden). The pellets were washed three times in PBS and resuspended in MRS broth. The OD was adjusted spectrophotometrically to 1.8 at 630 nm (corresponding to approximately 109 cfu/mL). The cultures were then serially diluted in MRS broth in 10-fold steps. One millilitre of the supernatants, undiluted suspensions, and cell suspensions corresponding to approximately 107, 105, and 103 CFU/mL were added to 24 m sterilized molten MRS agar (~45°C) in Petri dishes, and the agar was allowed to solidify. The plates were incubated overnight at 37°C under anaerobic conditions.
One single colony of each of the overnight cultured Candida strains was added to 5 mL DSM broth and aerobically incubated at 37°C for 24 h. The following day, one additional layer of 25 mL of molten sterile DSM agar was poured on top of the MRS agar with grown lactobacilli and supernatants, and was allowed to solidify and air-dry for 3 h at room temperature. The overnight-cultured Candida strains were diluted in DSM broth to a final OD of 0.2 at 500 nm. The Candida suspensions were stamped on the plates with a Steers steel-pin replicator (CMI-Promex ICN, Pedricktown, NJ) and left to dry for 1 h at room temperature. The plates were subsequently aerobically incubated overnight at 37°C. As controls, the Candida strains were also stamped on top of plates with no lactobacilli within the bottom MRS agar layer.
The assays were carried out in duplicate and repeated four times on different occasions. The results were evaluated in accordance with Simark-Mattson et al. [Citation23] as follows: a score of 0 = complete inhibition (no visible colonies); a score of 1 = slight inhibition (at least one visible colony but definitely smaller amounts than at control plate); and a score of 2 = no inhibition (colonies equal to those at the control plate). Two observers scored the plates independently, and in case of disagreement, consensus was reached through discussion.
Microsensor measurements of pH
To estimate the acid production of the lactobacilli and the effect of pH on Candida growth, pH was measured with a pH microelectrode (pH-25; Unisense A/S, Århus, Denmark) using a modification of a previously described procedure [Citation24] in selected plates from the abovementioned agar overlay interference test. A reference electrode was used to establish a reference potential against the pH microelectrode (ref-100, Unisense A/S). The electrodes were mounted in a motorized PC-controlled profiling setup (MM33 and MC-232, Unisense A/S). Positioning and data acquisition were controlled by dedicated software (Sensortrace Pro 2.0, Unisense A/S). The pH and reference electrodes were calibrated with buffers of pH 4 and 7 at room temperature. The pH microelectrode had a detection limit of 0.1 pH units. The pH was measured on the final day of the agar overlay interference test after scoring the plates. Selection of the plates was based on the results from the interference test: pH was measured through dense, non-inhibited colonies of Candida (C. krusei CCUG 56126 and C. tropicalis CCUG 47037) and through vague, almost completely inhibited colonies (C. albicans CCUG 46390 and C. glabrata CCUG 63819) incubated on plates with L. reuteri DSM 17938 and ATCC PTA 5289 (103 CFU/mL), respectively. In addition, pH was measured in control plates containing only the lactobacilli in the bottom agar layer, but with no Candida incubated, and in a control plate with Candida strains but without lactobacilli. Finally, pH was measured at a control agar plate without lactobacilli or Candida strains. Before commencing the measurements, the pH and reference electrodes were placed approximately 2 mm above the surface of the Candida colonies. The electrodes were set to move in steps of 100 µm, and pH was measured from the surface of the colonies and then for every 100 µm until the depth of 4.5 mm into the agar to make sure the sensors had reached the bottom agar layer containing the lactobacilli. The experiment was repeated twice at two different occasions.
H2O2 production by the lactobacilli
In order to verify the ability of the two L. reuteri strains to produce H2O2, a method described by Kang et al. [Citation25] was used. Briefly, one single colony of overnight cultured L. reuteri strains was plated onto MRS agar containing 0.25 mg/mL 3,3',5,5'-tetramethylbenzidine (Sigma, Poole, UK) and 0.01 mg/mL peroxidase from horseradish (Sigma) and incubated anaerobically for 48 h at 37°C. As controls, two bacterial strains known to produce H2O2 (Streptococcus sanguinis and S. mitis) and one strain known not to produce H2O2 (S. salivarius) [Citation26] were grown on similar plates incubated under the same conditions. After incubation, the plates were exposed to ambient air, and colonies displaying a surrounding blue halo were considered to produce H2O2.
Statistical analysis
Statistical analyses were processed with SAS Enterprise guide software v7.1 (SAS Institute, Inc., Cary, NC). A p-value of <0.05 was considered statistically significant. Data analysis on co-aggregation was subjected to one-way analysis of variance and Tukey’s post-hoc analysis, and presented as mean and standard deviations (SD). Data obtained from the agar overlay interference test were analysed by the chi-square test and presented as the median inhibition score.
Results
Co-aggregation between lactobacilli and yeasts
L. reuteri ATCC PTA 5289 and L. reuteri DSM 17938 showed the ability to co-aggregate with both clinical and reference Candida strains. Co-aggregation increased significantly over time (1, 2, and 4 h) for all combinations of Candida and probiotic strains (p <0.05). For all the tested Candida strains, L. reuteri PTA 5289 showed a statistically significantly higher co-aggregation ratio than L. reuteri DSM 17938 after 4 h incubation (p <0.001). The results of the co-aggregation ratios (%) after 4 h are presented in .
Figure 1. Co-aggregation ratio (%) between Candida strains and the Lactobacillus reuteri strains after 4 h of incubation. Mean, error bars indicate standard deviations.
*Statistically significant differences (p < 0.05) .L. reuteri DSM 17938: C. tropicalis DSM 7524 showed significantly higher co-aggregation ability compared with C. krusei CCUG 56126, C. dubliniensis 41_3 ZZMK, C. parapsilosis 26 PBS, C. glabrata CBS 863, and C. parapsilosis CCUG 56136. L. reuteri ATCC PTA 5289: C. tropicalis DSM 7524 showed significantly higher co-aggregation ability compared with C. glabrata CBS 863 and C. parapsilosis CCUG 56136. C. krusei CCUG 56126 showed a significantly greater ability to co-aggregate compared with C. parapsilosis CCUG 56136.
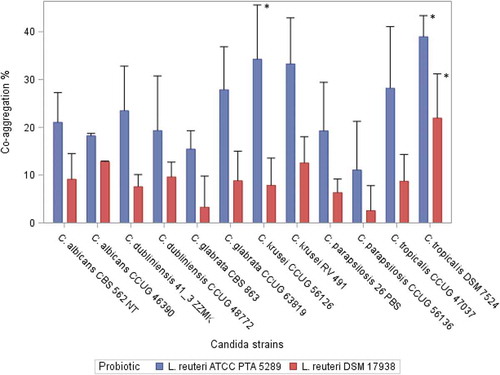
Growth inhibition of Candida by L. reuteri
The results of the growth inhibition assays are summarized in . Both L. reuteri strains were able to inhibit the growth of five of the tested Candida strains but not the two C. krusei strains. In general, L. reuteri DSM 17938 was significantly better at inhibiting the growth of the Candida strains than L. reuteri PTA 5289 was (p <0.001); the latter showed no complete inhibition of any of the Candida strains. No statistically significant differences were found between the two L. reuteri strains for C. krusei. High cell concentrations (109 cfu/mL) of L. reuteri displayed no superiority at inhibiting the Candida strains compared with lower cell concentrations of the bacteria (<107 cfu/mL) in the bottom agar layer. For C. tropicalis CCUG 47037, an even weaker inhibition tendency was observed at high L. reuteri DSM 17938 cell concentrations compared with lower cell concentrations, whereas C. glabrata CBS 863 was completely inhibited at high L. reuteri DSM 17938 cell concentrations compared with slight inhibition at lower concentrations.
Table 1. Growth inhibition of six clinical isolates and six reference Candida strains by two strains of the probiotic Lactobacillus reuteri at different cell concentrations.
Bacterial acid production measured by ph
Typical results of the pH microsensor measurements in the agar overlay cultures of the lactobacilli and Candida strains and control plates are shown in and . Similar pH microprofiles appeared for both strains of the lactobacilli incubated with the Candida; pH measured through the dense colonies of the Candida species that were not inhibited by the lactobacilli at the inhibition assay (C. krusei CCUG 56126 and Candida tropicalis CCUG 47037) was approximately pH 6.0 at the surface of the colonies, remaining above pH 4.5 until a depth of 1,500 µm, and only slowly becoming more acidic throughout the agar layers approaching approximately pH 4.0 in the bottom agar layer. In contrast, the pH measured through the almost completely inhibited colonies of C. albicans CCUG 46390 and C. glabrata CCUG 63819 already reached a very acidic level of approximately pH 3.6 only a few 100 µm under the surface and remained stable throughout the agar layers.
Figure 2. Microsensor measurement of pH at selected Candida strains grown by the agar overlay technique with L. reuteri ATCC PTA 5289 (103 cfu/mL) in the bottom agar layer. Zero on the vertical axis represents the first reading when the sensors hit either a Candida colony or the agar plates without Candida colonies. For the Candida controls without lactobacilli, data are only presented for C. krusei CCUG 56126.
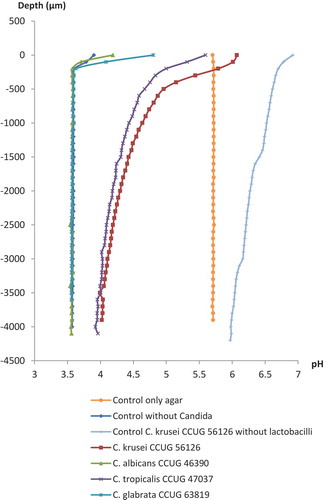
Figure 3. Microsensor measurement of pH at selected Candida strains grown by the agar overlay technique with L. reuteri DSM 17938 (103 cfu/mL) in the bottom agar layer. Zero on the vertical axis represents the first reading when the sensors hit either a Candida colony or the agar plates without Candida colonies. For the Candida controls without lactobacilli, data are only presented for C. krusei CCUG 56126.
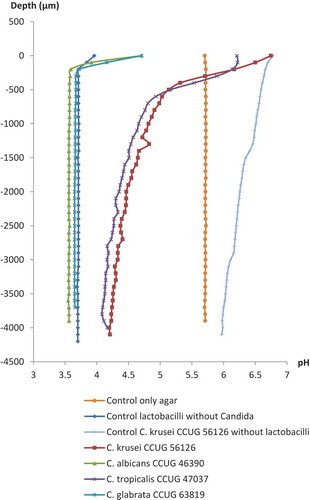
H2O2 production by the lactobacilli
Both L. reuteri DSM 17938 and L. reuteri PTA 5289 showed a positive reaction for H2O2 production by displaying clear-blue haloes around incubated colonies after around 1 h exposure to ambient air. A similar colour change was observed for S. sanguinis and S. mitis but not for S. salivarius, and the two L. reuteri strains were therefore verified as H2O2 producers.
Discussion
To the authors’ knowledge, this is the first study to demonstrate that probiotic lactobacilli can hamper the growth of a selection of the most commonly isolated Candida species in the oral cavity under in vitro conditions. It was found that both L. reuteri strains were able to co-aggregate with the yeasts, displaying the highest co-aggregation ratios with C. tropicalis and C. krusei. Unfortunately, these two Candida species seemed less susceptible to the substances produced by the lactobacilli, as their growth was only slightly inhibited in the agar overlay inhibition assay. In an in vitro study by Verdenelli et al. [Citation20], L. plantarum 319 displayed the highest degree of co-aggregation with C. glabrata and C. albicans among five Lactobacillus strains tested. As is the case for many probiotic properties of lactobacilli, co-aggregation also appears to be strain specific and is unique for each Lactobacillus strain involved. This was confirmed in this study, since L. reuteri ATCC PTA 5289 generally showed the highest co-aggregation capacity with all the Candida strains, and interestingly, this probiotic strain originates from the oral cavity. Co-aggregation is a recognized feature of the early biofilm formation and involves adhesion receptor interactions between complementary molecules on the microbial cell surfaces [Citation27]. As such, probiotic competition with pathogenic Candida strains for binding sites in the oral biofilms may partly explain the beneficial outcome of previous clinical trials [Citation5,Citation11].
The agar overlay interference test is a well-proven and relatively simple technique for exploring the inhibition capabilities of probiotic bacteria against oral pathogens [Citation14,Citation18,Citation23]. Moreover, the technique allows assessment of multiple Candida strains on a single plate and with different cell concentrations of the lactobacilli in the bottom agar layer. In this study, both L. reuteri strains were able to inhibit the growth of most of the Candida strains, except for C. krusei and to some extent C. tropicalis. This confirms the findings of other in vitro studies that investigated the antifungal effect of lactobacilli on Candida using varying techniques [Citation16,Citation19–Citation21]. Jiang et al. [Citation28] found that the most susceptible yeast to lactobacilli was C. albicans, whereas C. krusei was unaffected under all experimental conditions. Likewise, Zhao et al. [Citation29] failed to inhibit C. krusei in a disc diffusion model. In this study, L. reuteri DSM 17938 demonstrated stronger inhibition ability than L. reuteri ATCC PTA 5289 did. The pH was measured through the agar layers after 48 h of incubation of the lactobacilli, and both L. reuteri strains were equally good at producing organic acids, resulting in a pH close to 3.6 in the agar. Hence, superior interference of L. reuteri DSM 17938 must likely be explained by other factors, such as an enhanced H2O2 or reuterin production which has been suggested in other studies [Citation10,Citation16,Citation30].
It is likely that the observed inhibition of the yeasts is caused by the acidic environment in the agar due to lactic acid and other organic acids produced by the two L. reuteri strains, either directly or due to the production of bacteriocins at low pH [Citation31]. Neutral-to-alkaline extracellular pH is considered an optimal pH for the growth of Candida species, since it induces hyphal morphogenesis in C. albicans [Citation32], while at low pH, the fungus remains in the less virulent budding yeast form [Citation33]. In this study, a very acidic environment was found in the agar only a few 100 µm under the surface of the almost completely inhibited colonies of C. albicans and C. glabrata. The un-dissociated form of lactic acid has been shown to pass the plasma membrane of yeast cells, leading to an increased activity of an energy-consuming plasma membrane H+-ATPase that removes protons from the intracellular environment. The increased H+-ATPase activity exhausts the available energy for growth and metabolism, leading to growth inhibition and finally cell death [Citation31,Citation34]. Moreover, in this study, a completely different result was observed for the non-inhibited C. krusei and to some extent C. tropicalis. Somehow, these fungi had neutralized the acids, creating a pH of almost 6.0 at the surface and only slowly decreasing down through the agar. This indicates that these fungi inhibit the acidification caused by the lactobacilli to ensure their survival. In experimental studies, Halm et al. [Citation31] found C. krusei to be much more tolerant to lactic acid at low pH than Saccharomyces cerevisiae, since lactic acid only induced a weak short-term response in the intracellular pH of C. krusei. It is possible that C. krusei (i) actively produces extracellular ammonia (NH3) which will increase the surrounding pH [Citation32,Citation35], (ii) has less permeable plasma membranes to lactic acid, (iii) has a higher buffer capacity inside the cells, or (iv) has a higher H+-ATPase capacity than the other Candida species tested in this study [Citation31]. A combination of the abovementioned factors may also occur.
Acid production by the lactobacilli may be a concern in regard to the development of new caries lesions in the oral cavity. Lactobacilli and streptococci, especially S. mutans, are abundant acidogenic species in caries lesions in the oral cavity [Citation36]. Yet, L. reuteri strains have been shown to reduce the number of S. mutans in clinical trials after a continuous regular intake due to its production of substances with potent inhibitory activity on a wide range of bacterial species [Citation37]. However, the species cannot colonize the oral cavity after ended intake and is therefore not considered a threat to the development of new carious lesions [Citation38].
The strong oxidant H2O2 is a potent growth inhibitor and is mainly produced by oxidases in the carbon and energy metabolism of micro-organisms. The present study was able to verify the H2O2 production of both L. reuteri strains. However, the method used was more qualitative than quantitative and did not allow the amount of H2O2 produced by the two strains to be determined. Candida species have developed mechanisms to detoxify reactive oxygen species (ROS), such as H2O2, in order to survive and evade the host immune system [Citation39]. Ramírez-Quijas et al. [Citation39] found that C. albicans was more susceptible to H2O2 compared with C. glabrata, C. parapsilosis, and especially C. krusei which was the most resistant of the four species. There is a good possibility that the non-albicans Candida species possess mechanisms other than those possessed by C. albicans to detoxify ROS. Accordingly, it has been proposed that the resistance of C. krusei and C. parapsilosis to H2O2 is due to the presence of an oxidase which is part of an alternative oxidative pathway [Citation40].
It is important to stress that in vitro studies always have limitations, since they can never mimic the complex microbiota found in the oral cavity. In general, the effects of probiotic bacteria are highly strain specific, which was confirmed in this study. Even at strain level, significant differences in co-aggregation and growth inhibition abilities were found between the two L. reuteri strains. This indicates that probiotics as such cannot just be put in one barrel and that further in vitro and, more importantly, in vivo investigations are needed to increase the understanding on the role of probiotic supplements in the prevention and management of Candida infections in the oral cavity.
Conclusions
The two probiotic L. reuteri strains exhibited antifungal properties against five of the six most common Candida species in the oral cavity. The findings clearly showed that some Candida species, particularly C. krusei, were not affected by the probiotic interference. The probiotic properties differed significantly between the two Lactobacillus strains. Thus, the understanding that the impact of probiotic supplements is strain and host specific was reconfirmed.
Acknowledgements
The authors would like to thank Lasse A. Kvich, Department of Clinical Microbiology, Rigshopitalet, Copenhagen, Denmark, for generously providing the clinical isolated Candida strains. We would also like to thank laboratory technician Mia Ekstrand for her assistance in the laboratory. The experimental products were generously provided by Biogaia AB, Lund, Sweden. We are thankful for the financial support provided by the Danish Dental Association.
Disclosure statement
Dr. Jørgensen has received a faculty PhD grant at the University of Copenhagen, partly funded by Biogaia AB, Sweden. No potential conflict of interest was reported by the remaining authors.
Additional information
Funding
References
- Barros PP, Ribeiro FC, Rossoni RD, et al. Influence of Candida krusei and Candida glabrata on Candida albicans gene expression in in vitro biofilms. Arch Oral Biol. 2016;64:1–8.
- Sardi JC, Scorzoni L, Bernardi T, et al. Candida species: current epidemiology, pathogenicity, biofilm formation, natural antifungal products and new therapeutic options. J Med Microbiol. 2013;62:10–24.
- Kragelund C, Kieffer-Kristensen L, Reibel J, et al. Oral candidosis in lichen planus: the diagnostic approach is of major therapeutic importance. Clin Oral Investig. 2013;17:957–965.
- Matsubara VH, Bandara HM, Mayer MP, et al. Probiotics as antifungals in mucosal candidiasis. Clin Infect Dis. 2016;62:1143–1153.
- Laleman I, Teughels W. Probiotics in the dental practice: a review. Quintessence Int. 2015;46:255–264.
- Sanders ME. Probiotics: definition, sources, selection, and uses. Clin Infect Dis. 2008;46(Suppl 2):S58-S61; discussion S144-S151.
- Jorgensen MR, Keller MK, Kragelund C, et al. Lactobacillus reuteri supplements do not affect salivary IgA or cytokine levels in healthy subjects: a randomized, double-blind, placebo-controlled, cross-over trial. Acta Odontol Scand. 2016;74:399–404.
- Oever JT, Netea MG. The bacteriome-mycobiome interaction and antifungal host defense. Eur J Immunol. 2014;44:3182–3191.
- Valeur N, Engel P, Carbajal N, et al. Colonization and immunomodulation by Lactobacillus reuteri ATCC 55730 in the human gastrointestinal tract. Appl Environ Microbiol. 2004;70:1176–1181.
- Cleusix V, Lacroix C, Vollenweider S, et al. Inhibitory activity spectrum of reuterin produced by Lactobacillus reuteri against intestinal bacteria. BMC Microbiol. 2007;7:101.
- Kraft-Bodi E, Jorgensen MR, Keller MK, et al. Effect of probiotic bacteria on oral Candida in frail elderly. J Dent Res. 2015;94:181S–6S.
- Collado MC, Meriluoto J, Salminen S. Adhesion and aggregation properties of probiotic and pathogen strains. Eur Food Restechnol. 2008;226:1065–1073.
- Twetman L, Larsen U, Fiehn NE, et al. Coaggregation between probiotic bacteria and caries-associated strains: an in vitro study. Acta Odontol Scand. 2009;67:284–288.
- Keller MK, Hasslof P, Stecksen-Blicks C, et al. Co-aggregation and growth inhibition of probiotic lactobacilli and clinical isolates of mutans streptococci: an in vitro study. Acta Odontol Scand. 2011;69:263–268.
- Saha S, Tomaro-Duchesneau C, Rodes L, et al. Investigation of probiotic bacteria as dental caries and periodontal disease biotherapeutics. Benef Microbes. 2014;5:447–460.
- Kohler GA, Assefa S, Reid G. interference of Lactobacillus rhamnosus GR-1 and Lactobacillus reuteri RC-14 with the opportunistic fungal pathogen Candida albicans. Infect Dis Obstet Gynecol. 2012;2012:1–14. DOI:10.1155/2012/636474
- Strus M, Kucharska A, Kukla G, et al. The in vitro activity of vaginal Lactobacillus with probiotic properties against Candida. Infect Dis Obstet Gynecol. 2005;13:69–75.
- Hasslof P, Hedberg M, Twetman S, et al. Growth inhibition of oral mutans streptococci and candida by commercial probiotic lactobacilli - an in vitro study. BMC Oral Health. 2010;10:18.
- Coman MM, Verdenelli MC, Cecchini C, et al. In vitro evaluation of antimicrobial activity of Lactobacillus rhamnosus IMC 501(R), Lactobacillus paracasei IMC 502(R) and SYNBIO(R) against pathogens. J Appl Microbiol. 2014;117:518–527.
- Verdenelli MC, Coman MM, Cecchini C, et al. Evaluation of antipathogenic activity and adherence properties of human Lactobacillus strains for vaginal formulations. J Appl Microbiol. 2014;116:1297–1307.
- Chew SY, Cheah YK, Seow HF, et al. Probiotic Lactobacillus rhamnosus GR-1 and Lactobacillus reuteri RC-14 exhibit strong antifungal effects against vulvovaginal candidiasis-causing Candida glabrata isolates. J Appl Microbiol. 2015;118:1180–1190.
- Angeletti S, Lo Presti A, Cella E, et al. Matrix-assisted laser desorption/ionization time of flight mass spectrometry (MALDI-TOF MS) and Bayesian phylogenetic analysis to characterize Candida clinical isolates. J Microbiol Methods. 2015;119:214–222.
- Simark-Mattsson C, Emilson CG, Hakansson EG, et al. Lactobacillus-mediated interference of mutans streptococci in caries-free vs. caries-active subjects. Eur J Oral Sci. 2007;115:308–314.
- Kolpen M, Appeldorff CF, Brandt S, et al. Increased bactericidal activity of colistin on Pseudomonas aeruginosa biofilms in anaerobic conditions. Pathog Dis. 2016;74. DOI:10.1093/femspd/ftv086
- Kang MS, Oh JS, Lee HC, et al. Inhibitory effect of Lactobacillus reuteri on periodontopathic and cariogenic bacteria. J Microbiol. 2011;49:193–199.
- Ryan CS, Kleinberg I. Bacteria in human mouths involved in the production and utilization of hydrogen peroxide. Arch Oral Biol. 1995;40:753–763.
- Jenkinson HF, Lala HC, Shepherd MG. Coaggregation of Streptococcus sanguis and other streptococci with Candida albicans. Infect Immun. 1990;58:1429–1436.
- Jiang Q, Stamatova I, Kari K, et al. Inhibitory activity in vitro of probiotic lactobacilli against oral Candida under different fermentation conditions. Benef Microbes. 2015;6:361–368.
- Zhao C, Lv X, Fu J, et al. In vitro inhibitory activity of probiotic products against oral Candida species. J Appl Microbiol. 2016;121:254–262.
- Eschenbach DA, Davick PR, Williams BL, et al. Prevalence of hydrogen peroxide-producing Lactobacillus species in normal women and women with bacterial vaginosis. J Clin Microbiol. 1989;27:251–256.
- Halm M, Hornbaek T, Arneborg N, et al. Lactic acid tolerance determined by measurement of intracellular pH of single cells of Candida krusei and Saccharomyces cerevisiae isolated from fermented maize dough. Int J Food Microbiol. 2004;94:97–103.
- Vylkova S, Carman AJ, Danhof HA, et al. The fungal pathogen Candida albicans autoinduces hyphal morphogenesis by raising extracellular pH. MBio. 2011;2:e00055–e00011.
- Buffo J, Herman MA, Soll DR. A characterization of pH-regulated dimorphism in Candida albicans. Mycopathologia. 1984;85:21–30.
- Holyoak CD, Stratford M, McMullin Z, et al. Activity of the plasma membrane H+-ATPase and optimal glycolytic flux are required for rapid adaptation and growth of Saccharomyces cerevisiae in the presence of the weak-acid preservative sorbic acid. Appl Environ Microbiol. 1996;62:3158–3164.
- Zikanova B, Kuthan M, Ricicova M, et al. Amino acids control ammonia pulses in yeast colonies. Biochem Biophys Res Commun. 2002;294:962–967.
- Ahola AJ, Yli-Knuuttila H, Suomalainen T, et al. Short-term consumption of probiotic-containing cheese and its effect on dental caries risk factors. Arch Oral Biol. 2002;47:799–804.
- Cagetti MG, Mastroberardino S, Milia E, et al. The use of probiotic strains in caries prevention: a systematic review. Nutrients. 2013;5:2530–2550.
- Caglar E, Topcuoglu N, Cildir SK, et al. Oral colonization by Lactobacillus reuteri ATCC 55730 after exposure to probiotics. Int J Paediatr Dent. 2009;19:377–381.
- Ramirez-Quijas MD, Lopez-Romero E, Cuellar-Cruz M. Proteomic analysis of cell wall in four pathogenic species of Candida exposed to oxidative stress. Microb Pathog. 2015;87:1–12.
- Costa-de-Oliveira S, Sampaio-Marques B, Barbosa M, et al. An alternative respiratory pathway on Candida krusei: implications on susceptibility profile and oxidative stress. FEMS Yeast Res. 2012;12:423–429.