ABSTRACT
Tetracycline resistance can be achieved through tet genes, which code for efflux pumps, ribosomal protection proteins and inactivation enzymes. Some of these genes have only been described in either Gram-positive or Gram-negative bacteria. This is the case of tet(B), which codes for an efflux pump and, so far, had only been found in Gram-negative bacteria. In this study, tet(B) was detected in two clinical Streptococcus oralis strains isolated from the gingival sulci of two subjects. In both cases, the gene was completely sequenced, yielding 100% shared identity and coverage with other previously published sequences of tet(B). Moreover, we studied the expression of tet(B) using RT-qPCR in the isolates grown with and without tetracycline, detecting constitutive expression in only one of the isolates, with no signs of expression in the other one.
This is the first time that the presence and expression of the tet(B) gene has been confirmed in Gram-positive bacteria, which highlights the potential of the genus Streptococcus to become a reservoir and a disseminator of antibiotic resistance genes in an environment so prone to horizontal gene transfer as is the oral biofilm.
Since their discovery in the 1940s, tetracyclines have been used for many years to treat a wide spectrum of infections. However, resistance to these antibiotics soon appeared, and only the discovery of new derivatives allowed their continued use until the 1980s, when they were gradually replaced by other antibiotics such as fluoroquinolones [Citation1].
Despite their widespread resistance, some tetracyclines are still useful in some treatments, such as in acne vulgaris, periodontitis and infections caused by multidrug resistant (MDR) Acinetobacter baumannii, Helicobacter pylori and methicillin-resistant Staphylococcus aureus [Citation2–Citation4]. Resistance can occur through efflux pumps, ribosomal protection or enzymatic inactivation. According to http://faculty.washington.edu/marilynr/(last update May 1st, 2019), 60 tetracycline resistance genes have been detected that code for these mechanisms, along with 11 mosaic genes that code for ribosomal protection proteins. Some of these genes, such as tet(C) and tet(32), have only been found in Gram-positive bacteria, while others such as tet(B) have only been found in Gram-negative bacteria. In a recent study by Chander et al., tet(B) was found in Streptococcus suis isolated from pigs [Citation5], but a lack of full sequencing of the gene prevented the confirmation of such a finding.
In oral biofilm, streptococci are a great reservoir of tetracycline resistance genes [Citation6–Citation8], and one of the most common in the oral environment is S. oralis [Citation9]. S. oralis is a member of the Streptococcus mitis group, known to be a commensal bacterium and opportunistic pathogen [Citation10]. S. oralis is an early colonizer of the oral biofilm and plays an important role in its establishment and homeostasis [Citation11]. Moreover, it has been described to antagonize bacteria such as Streptococcus mutans, thus preventing the development of dysbiotic biofilm [Citation12]. However, it is one of the most isolated bacteria in endocarditis infections [Citation13,Citation14] and it has been found in numerous types of infection sites [Citation15–Citation19].
Transformation among streptococci is a common event, as they exhibit natural competence and easily acquire foreign DNA. Therefore, the transference of antibiotic resistance genes has been largely studied in this genus [Citation20,Citation21]. Bacteria in the oral environment tend to grow by forming a multispecies biofilm, which increases the chances of horizontal gene transfer and the spread of antimicrobial resistance, creating a large reservoir of antibiotic resistance genes that could be transferred to other bacteria, pathogenic or not [Citation22].
In this study we described the presence of tet(B) in two S. oralis isolates obtained from subgingival samples of healthy volunteers. Furthermore, in order to prove the presence and transcription of the gene in Gram-positive bacteria, the complete sequence of the gene was obtained, and its expression was evaluated in both isolates.
Materials and methods
Sample collection and culture
Subgingival samples were collected from periodontally healthy volunteers in the dental clinic of the Universitat Internacional de Catalunya (UIC), (Barcelona, Spain), and the research protocol was approved by the Ethics Committee of the UIC (study number: PER-ECL-2011–06-NF). Subjects had not received antibiotics 3 months prior to the study and did not show signs of gingivitis or periodontitis. Sterile paper points were inserted into the gingival sulci of teeth 16, 21, 36 and 41 and kept in place for 20 sec. These paper points were pooled in a vial containing 1.5 ml of sterilized reduced transport fluid (RTF) without EDTA [Citation23] and sent to the laboratory at 4ºC for processing within 24 h. Samples were serially diluted and plated on blood agar (Oxoid Nutrient Broth No. 2; Oxoid Ltd, Basingstoke, UK) containing 5% horse blood, hemin (5 mg/l), menadione (1 mg/ml) and 8 µg/ml of tetracycline (Sigma Aldrich, St. Louis, MO, USA) to screen for tetracycline resistant bacteria. Incubation was performed at 37ºC in anaerobic conditions, and after 72 h, all different colony morphologies were subcultured to obtain pure cultures. For each selected isolate, liquid cultures with and without tetracycline (8 µg/ml) were done using modified brain heart infusion (brain heart infusion broth [37 g/l], yeast extract [1 g/l], L-cysteine [0.1 g/l], sodium bicarbonate [2 g/l], and supplemented with hemin [5 mg/l] and glutamic acid [0.25%]). Cultures grew to an OD600 of 0.8–1 (≈ 108 cfu/ml), when DNA and RNA extractions were performed.
Detection of tet(B)
DNA was extracted from all the isolates using the QIAamp DNA Mini Kit (Qiagen, Hilden, Germany) according to the manufacturer’s instructions, quantified using a Nanodrop 2000 UV-vis spectrophotometer (Nanodrop Technologies, Wilmington, DE, USA) and visualized in a 0.5% agarose gel stained with ethidium bromide.
All the isolates were screened for a battery of tet genes (data not shown). To detect tet(B) the primers tetB-F and tetB-R were used () with the following PCR conditions: an initial denaturation of 5 min at 95ºC, 30 cycles of 30 sec of denaturation at 95ºC, 30 sec of annealing at 56.5ºC and 45 sec of extension at 72ºC, and a final extension of 10 min at 72ºC. The result of the PCR was visualized in a 3% agarose gel stained with ethidium bromide, where a band of 242 bp could indicate the presence of tet(B).
Table 1. List of primers used in this study.
DNA sequencing
tet(B) was found in two isolates, 444.1 and 469.4, from two different subjects. In order to identify each isolate to the species level, the 16S rRNA, sodA and groEL genes were amplified using the primers 27-F and 1544-R, sodA-F and sodA-R, and groEL-F and groEL-R, respectively (). The PCR products were purified using the E.Z.N.A.® Gel extraction Kit (Omega BIO-TEK, Norcross, GA, USA) and sequenced by primer walking at the Genomics and Bioinformatics Service of the Autonomous University of Barcelona (Barcelona, Spain). Primers tetB-insert-F and tetB-insert-R () were used to fully sequence the tet(B) gene. These primers amplified the gene 41 bp upstream of the start codon and 72 bp downstream of the stop codon. The 1,319 bp amplicons were obtained by PCR and were purified and sequenced by primer walking as described above. The sequences obtained were analyzed using BLASTn software at https://blast.ncbi.nlm.nih.gov/Blast.cgi. Reference sequences for alignment were obtained from the database on http://faculty.washington.edu/marilynr/, specifically the sequences with the accession numbers: J01830, AF223162, V00611, AL513383, AJ277653, AF326777 and AP000342. Additionally, other sequences of tet(B) from other species were used to ensure better reliability: CP015434.1, KX458222.1, CP015836.1, NG_048163.1 and LN908249.1. Alignment was performed using the Clustal Omega service at https://www.ebi.ac.uk/Tools/msa/clustalo/.
RNA isolation and expression of tet(B) gene
RNA was extracted from liquid cultures with and without tetracycline using the High Pure RNA Isolation kit (Roche Diagnostics, Mannheim, Germany) according to the instructions provided by the manufacturer. An extra step to remove any remaining DNA was done using the TURBO DNA-freeTM Kit (Thermo Fisher Scientific, Vilnius, Lithuania). The absence of DNA in the samples was verified by PCR using the sets of primers tetB-RT-F/tetB-RT-R, and 16S-So-F/16S-So-R (), visualizing the results in a 3% agarose gel. The remaining RNA was quantified in a Qubit 4 Fluorometer using the Qubit RNA XR Assay Kit (Thermo Fisher Scientific, Eugene, OR, USA), and its integrity was verified on a 2% agarose gel.
Expression of tet(B) was measured by Quantitative Reverse Transcription PCR (RT-qPCR). Reactions were performed with a LightCycler® 480 II Instrument using the LightCycler® 480 RNA Master Hydrolysis Probes kit (Roche Diagnostics, Mannheim Germany), following the instructions provided by the manufacturer. The sets of primers tetB-RT and 16S-So () were used together with probes 45 and 66 from the Roche Diagnostics’ Universal ProbeLibrary for the genes tet(B) and 16S rRNA, respectively. The RT-qPCR conditions were as follows: i) 75 ng of RNA were used in each reaction for the synthesis of cDNA, which was performed at 63ºC for 3 min; ii) cycling conditions were 95ºC for 30 sec, followed by 45 cycles of 15 sec at 95ºC, 40 sec at 60ºC and 1 sec at 72ºC. Data were analyzed using the LightCycler® 480 Software 1.5. The 16S rRNA gene was used as an endogenous control, and the formula 2ΔCptet(B)/2ΔCp16S rRNA was used to evaluate differences in the expression of tet(B) in the presence and absence of tetracycline.
Construction of tet(M) defective mutants
Isolate 469.4 was found to harbor the gene tet(M). Therefore, in order to determine the contribution of tet(B) to the tetracycline resistance of this isolate, disruption of the gene tet(M) was performed by introducing the gene erm(B), which confers resistance to erythromycin, between the positions 853 and 1,110 of the tet(M) gene. To this end, we made a construct consisting of the gene erm(B) surrounded by two sequences homologous to tet(M), which we named M1 (the 5ʹ region) and M2 (the 3ʹ region). erm(B) was extracted by PCR using the primers ermB-NdeI-F and ermB-HindIII-R from a S. oralis clinical strain resistant to erythromycin pertaining to the Dentaid Research Center (Cerdanyola del Vallès, Spain) strain collection. M1 and M2 sequences were obtained by PCR from the isolate 469.4 using the primers M1-F and M1-NdeI-R for the M1 sequence, and M2-HindIII-F and M2-R for the M2 sequence (). PCR products were purified using the E.Z.N.A.® Gel extraction Kit and digested with NdeI and HindIII (New England Biolabs Inc., Ipswich, MA, USA). Digested fragments were ligated using T4 ligase (Roche Diagnostics, Mannheim, Germany) according to the manufacturer’s instructions. The construct was then purified using the E.Z.N.A.® Gel extraction Kit and transformed into 469.4 by electroporation using the Gene Pulser Xcell (BioRad, Hercules, CA, USA). Electroporated cells were plated on blood agar plates containing 5 µg/ml of erythromycin (Sigma Aldrich, St. Louis, MO, USA) and incubated in anaerobic conditions for 24–48 h. The recombination event was confirmed by PCR using primers M1-F and M2-R.
Antibiotic susceptibility testing
Susceptibility to tetracycline was tested in 444.1, 469.4 and 469.4Δtet(M)::erm(B)(EryR) using E-test strips (Sigma Aldrich, St. Louis, MO, USA) according to manufacturer’s instructions. Streptococcus pneumoniae ATCC 49619 was used as a quality control strain.
Results
Species identification and tet(B) sequences
From a library of 448 tetracycline resistant isolates (data not shown), two isolates obtained from two different subjects were found to carry the tet(B) gene. Sequencing of the 16S rRNA gene revealed that both isolates were S. oralis and shared the same sequence, which showed 100% identity with the 16S rRNA sequences from S. oralis ATCC 700233, DSMZ 20,066 and CIP 103216. To further corroborate this result, sequences of the genes sodA and groEL were also aligned to streptococcal sequences, observing the highest homology with S. oralis sequences. To ensure the presence of tet(B), full sequencing of the gene and further alignment against reference sequences was performed, showing an identity of 99.99–100% of the tet(B) isolated in this study. Sequence J01830 was used as a reference, being the first published sequence of tet(B) [Citation24]. Point mutations were found in all of the sequences used compared to J01830 (Supplementary Figure 1). tet(B) sequences of isolates 444.1 and 469.4, as well as sequences AF223162, AF326777, AL513383, CP015836.1, KX458222.1, LN908249.1 and NG_048163.1, presented five point mutations in positions 463, 794, 854, 940 and 1012. Sequence CP015434.1 exhibited an additional point mutation in position 1,140, and sequence AP000342 had four of these point mutations in positions 794, 854, 940 and 1,012. All these mutations resulted in amino acid substitutions with the exception of the mutations in position 1,140 ( and Supplementary Figure 2).
Table 2. Positions of nucleotide substitutions in the tet(B) sequences used as comparison. Between parentheses, the positions and amino acid substitutions corresponding to each nucleotide substitution. NC: no changes.
Sequences obtained in this study were submitted to GenBank (https://www.ncbi.nlm.nih.gov/genbank/), and the following accession numbers were retrieved: MK611935 and MK611934 for the 16S rRNA gene of 444.1 and 469.4, respectively, and MK611936 and MK611937 for the tet(B) gene of 444.1 and 469.4, respectively.
Expression of tet(B)
The level of expression of tet(B) in isolates 444.1 and 469.4 was determined comparing log-phase liquid cultures with and without tetracycline of each isolate. The exposure to tetracycline did not decrease the growth rate of the bacteria recovered from the culture and yielded similar amounts of RNA.
A transcriptional analysis of the gene tet(B) was performed using RT-qPCR. tet(B) mRNA was detected in isolate 469.4 but not in isolate 444.1, despite showing very similar levels of 16S rRNA expression (). As for isolate 469.4, no differences were observed in the expression of tet(B) when applying tetracycline to the medium. The gene expression value, normalized with the 16S rRNA gene, was 0.06 (log2).
Figure 1. Amplification curves of the 16S rRNA and tet(B) genes obtained by RT-qPCR from RNA of 444.1 and 469.4.
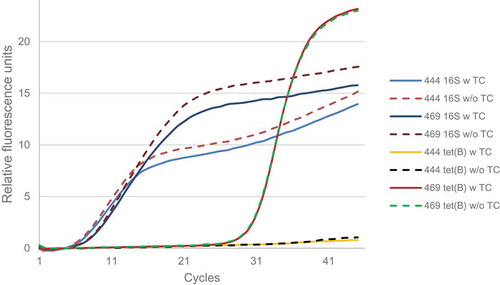
According to previous screening for tet genes, isolate 469.4 contained a tet(M) gene in addition to tet(B). In order to determine the influence of tet(B) on the tetracycline resistance of the isolate 469.4, the mutant 469.4Δtet(M)::erm(B)(EryR) was constructed.
Antibiotic susceptibility
Susceptibility to tetracycline was tested in isolates 444.1 and 469.4 using E-test strips, observing a minimum inhibitory concentration (MIC) of 32 µg/ml to tetracycline. In order to assess whether tetracycline resistance was being conferred by the gene tet(M) as opposed to tet(B), susceptibility to tetracycline in the mutant strain 469.4Δtet(M)::erm(B)(EryR) was also tested, observing a MIC of 32 µg/ml.
Discussion
Resistance to tetracyclines is widespread, and although tetracyclines are still useful in some treatments, only the most recent versions are being used to treat multidrug resistant bacteria [Citation25]. Nevertheless, a new formulation of minocycline, a second generation tetracycline, has been proven effective against MDR A. baumannii infections, allowing an old antibiotic to be used again [Citation4,Citation25]. Unfortunately, resistance to these antibiotics is possible through different mechanisms such as efflux pumps like the one coded by tet(B), which can recognize different tetracyclines as substrates, including minocycline, doxycycline and even glycylcyclines through punctual mutations [Citation26]. tet(B) is the most distributed tetracycline resistance gene among Gram-negatives [Citation27] and so far it has not been described in Gram-positives. However, a recent study [Citation5] reported to have found tet(B) in S. suis, being the first time to describe the presence of this gene in a Gram-positive bacteria. Nonetheless, and although sequencing was performed and 100% identity was obtained, only 54.55% of the gene was covered, raising doubts of whether the gene might or might not be tet(B). According to Levy et al. [Citation28], tetracycline resistance determinants should have ≤ 80% of amino acid identity in order to be considered different genes, which makes it impossible for the previously discussed analysis to determine if the gene studied was tet(B).
In our study we found two isolates of S. oralis susceptible of carrying tet(B). In both cases, we performed full sequencing of the gene, resulting in 100% coverage, and compared the sequences to those tet(B) sequences previously published, showing some minor differences involving up to six nucleotides in a sequence of 1,206 base pairs, which led to up to five amino acid substitutions in a sequence of 401 amino acids (100% – 98.75% identity). Most of the polymorphisms observed in the tet(B) sequences obtained from isolates 444.1 and 469.4 were shared by all the published sequences used in this study with the exception of V00611 and AJ277653, which were identical to the reference sequence J01830 (). These three sequences were obtained from Escherichia coli, although CP015836.1, another E. coli sequence used in this study, presented five point mutations compared to J01830, implying that these mutations might have occurred at some point during replication or transference and were maintained in certain strains.
tet(B) expression was studied to understand the functionality of the gene in the two S. oralis isolates obtained. It is known that, in the presence of tetracycline, the transcription of tetracycline resistance genes is induced [Citation29,Citation30]. For this reason, cultures were made with and without tetracycline and RNA was extracted to quantify the expression of the gene. tet(B) mRNA could only be found in isolate 469.4, meaning that the gene was not being expressed under those conditions in isolate 444.1. Furthermore, expression in 469.4 seemed to be constitutive, since its transcription did not depend on the addition of tetracycline, as it was observed once normalized with the expression values of the 16S rRNA gene. Both isolates carried tet(M) as well, which might have conferred resistance to tetracycline, as opposed to tet(B). We disabled the tet(M) gene in the 469.4 isolate through homologous recombination to determine its role in the level of tetracycline resistance, and we found no changes in the level of susceptibility, indicating that tet(B) could be responsible for tetracycline resistance in isolate 469.4, although it cannot be ruled out that resistance to tetracycline could be provided by another gene or mechanism.
The presence of tet(B) in Gram-positive bacteria is another step in the dissemination of antibiotic resistance genes. Although tet(B) seemed to be expressed in only one of the isolates, the presence of this tetracycline resistance gene had never been confirmed in Gram positives. Whether tet(B) is being expressed or not, it seems clear that the reservoir for this gene has expanded to the genus Streptococcus, an enormous bacterial group, increasing the chances for its dissemination. The transfer of antibiotic resistance genes between Gram-positive and Gram-negative bacteria has been previously reported [Citation31-Citation33], and the oral environment offers a great opportunity for these transfers to occur. The characteristics of the oral biofilm make it prone to genetic exchange, allowing the bacteria that live in such environment to acquire and share antibiotic resistance genes, among others [Citation22,Citation34-Citation36]. Furthermore, the oral environment is a transit area for many bacteria that might end up in other niches of the human body, thus creating the opportunity for these bacteria to acquire genetic determinants present in the oral microbiome. Given that the tet(B) sequence analyzed in this study was identical to other sequences of tet(B) observed in Gram-negatives, the functionality of this gene, being transferred to Gram-negatives, would be expected. In this study we confirmed the presence and expression of the gene tet(B) in Gram-positive bacteria. However, further studies should be conducted in order to establish the function of the gene tet(B) in isolates 444.1 and 469.4. Moreover, a full characterization of the surroundings of the gene and its ability to transfer among other streptococci might help to understand the actual potential of the tet(B) gene in Gram-positive bacteria.
Disclosure statement
No potential conflict of interest was reported by the authors.
References
- Thaker M, Spanogiannopoulos P, Wright GD. The tetracycline resistome. Cell Mol Life Sci. 2010;67(3):419–7.
- Smilack JD. The tetracyclines. Mayo Clin Proc. 1999;74(7):727–729.
- Herrera D, Alonso B, León R, et al. Antimicrobial therapy in periodontitis: the use of systemic antimicrobials against the subgingival biofilm. J Clin Periodontol. 2008;35(8 Suppl):45–66.
- Castanheira M, Mendes RE, Jones RN. Update on Acinetobacter species: mechanisms of antimicrobial resistance and contemporary In vitro activity of minocycline and other treatment options. Clin Infect Dis. 2014;59(6 Suppl):367–373.
- Chander Y, Oliveira SR, Goyal SM. Identification of the tet(B) resistance gene in Streptococcus suis. Vet J. 2011;189(3):359–360.
- Villedieu A, Diaz-Torres ML, Hunt N, et al. Prevalence of tetracycline resistance genes in oral bacteria. Antimicrob Agents Chemother. 2003;47(3):878–882.
- Lancaster H, Ready D, Mullany P, et al. Prevalence and identification of tetracycline-resistant oral bacteria in children not receiving antibiotic therapy. FEMS Microbiol Lett. 2003;228(1):99–104.
- Pompilio A, Di Bonaventura G, Gherardi G. An overview on Streptococcus bovis/Streptococcus equinus complex isolates: identification to the species/subspecies level and antibiotic resistance. Int J Mol Sci. 2019;20(3):480.
- Li J, Helmerhorst EJ, Leone CW, et al. Identification of early microbial colonizers in human dental biofilm. J Appl Microbiol. 2004;97(6):1311–1318.
- Mitchell J. Streptococcus mitis: walking the line between commensalism and pathogenesis. Mol Oral Microbiol. 2011;26(2):89–98.
- Kolenbrander PE, London J. Adhere today, here tomorrow: oral bacterial adherence. J Bacteriol. 1993;175(11):3247–3252.
- Thurnheer T, Belibasakis GN. Streptococcus oralis maintains homeostasis in oral biofilms by antagonizing the cariogenic pathogen Streptococcus mutans. Mol Oral Microbiol. 2018;33(3):234–239.
- Douglas CWI, Heath J, Hampton KK, et al. Identity of viridans streptococci isolated from cases of infective endocarditis. J Med Microbiol. 1993;39(3):179–182.
- Hosokawa S, Okayama H, Hiasa G, et al. Isolated left atrial infective mural endocarditis. Intern Med. 2018;57(7):957–960.
- Roy P, Srinivasan S, Chattoraj A. Balanoposthitis caused by Streptococcus mitis/oralis. Med J Armed Forces India. 2016;72(4):407–409.
- Shelburne SA, Sahasrabhojane P, Saldana M, et al. Streptococcus mitis strains causing severe clinical disease in cancer patients. Emerg Infect Dis. 2014;20(5):762–771.
- Thiagarajan S, Krishnamurthy S, Raghavan R, et al. Streptococcus oralis cerebral abscess following monkey bite in a 2-month-old infant. Paediatr Int Child Health. 2016;36(2):160–162.
- Chung JK, Lee SJ. Streptococcus mitis/oralis endophthalmitis management without phakic intraocular lens removal in patient with iris-fixated phakic intraocular lens implantation. BMC Ophthalmol. 2014;14:92.
- Poi BN, Pasupulety Venkata NK, Auckland CR, et al. Neonatal meningitis and maternal sepsis caused by Streptococcus oralis. J Neonatal Perinatal Med. 2018;11(3):331–334.
- Cvitkovitch DG. Genetic competence and transformation in oral streptococci. Crit Rev Oral Biol Med. 2001;12(3):217–243.
- Santoro F, Iannelli F, Pozzi G. Genomics and genetics of Streptococcus pneumoniae. Microbiol Spectr. 2019;7:3.
- Roberts AP, Mullany P. Oral biofilms: a reservoir of transferable, bacterial, antimicrobial resistance. Expert Rev Anti Infect Ther. 2010;8(12):1441–1450.
- Syed SA, Loesche WJ. Survival of human dental plaque flora in various transport media. Appl Microbiol. 1972;24(4):638–644.
- Hillen W, Schollmeier K. Nucleotide sequence of the Tn10 encoded tetracycline resistance gene. Nucleic Acids Res. 1983;11(2):525–539.
- Nguyen F, Starosta AL, Arenz S, et al. Tetracycline antibiotics and resistance mechanisms. Biol Chem. 2014;39(5):559–575.
- Guay GG, Tuckman M, Rothstein DM. Mutations in the tetA(B) gene that cause a change in substrate specificity of the tetracycline efflux pump. Antimicrob Agents Chemother. 1994;38(4):857–860.
- Chopra I, Roberts M. Tetracycline antibiotics: mode of action, applications, molecular biology, and epidemiology of bacterial resistance. Microbiol Mol Biol Rev. 2001;65(2):232–260.
- Levy SB, McMurry LM, Barbosa TM, et al. Nomenclature for new tetracycline resistance determinants. Antimicrob Agents Chemother. 1999;43(6):1523–1524.
- Hillen W, Berens C. Mechanisms underlying expression of Tn10 encoded tetracycline resistance. Annu Rev Microbiol. 1994;48:345–369.
- Saenger W, Orth P, Kisker C. et al. The tetracycline repressor-A paradigm for a biological switch. Angew Chem Int Ed Engl. 2000;39(12):2042–2052.
- Ojo KK, Tung D, Luis H, et al. Gram-positive merA gene in gram-negative oral and urine bacteria. FEMS Microbiol Lett. 2004;238(2):411–416.
- Ojo KK, Ulep C, Van Kirk N, et al. The mef(A) gene predominates among seven macrolide resistance genes identified in gram-negative strains representing 13 genera, isolated from healthy Portuguese children. Antimicrob Agents Chemother. 2004;48(9):3451–3456.
- Kurenbach B, Bohn C, Prabhu J, et al. Intergeneric transfer of the Enterococcus faecalis plasmid pIP501 to Escherichia coli and Streptomyces lividans and sequence analysis of its tra region. Plasmid. 2003;50(1):86–93.
- Hannan S, Ready D, Jasni AS, et al. of antibiotic resistance by transformation with eDNA within oral biofilms. FEMS Immunol Med Microbiol. 2010;59(3):345–349.
- Warburton PJ, Palmer RM, Munson MA, et al. Demonstration of in vivo transfer of doxycycline resistance mediated by a novel transposon. J Antimicrob Chemother. 2007;60(5):973–980.
- Willi K, Sandmeier H, Kulik EM, et al. Transduction of antibiotic resistance markers among Actinobacillus actinomycetemcomitans strains by temperate bacteriophages Aaφ23. Cell Mol Life Sci. 1997;53(11–12):904–910.
- Shigematsu T, Hayashi M, Kikuchi I, et al. A culture-dependent bacterial community structure analysis based on liquid cultivation and its application to a marine environment. FEMS Microbiol Lett. 2009;293(2):240–247.
- Poyart C, Quesne G, Coulon S, et al. Identification of streptococci to species level by sequencing the gene encoding the manganese-dependent superoxide dismutase. J Clin Microbiol. 1998;36(1):41–47.
- Glazunova OO, Raoult D, Roux V. Partial sequence comparison of the rpoB, sodA, groEL and gyrB genes within the genus Streptococcus. Int J Syst Evol Microbiol. 2009;59(9):2317–2322.
- Ng L-K, Martin I, Alfa M, et al. Multiplex PCR for the detection of tetracycline resistant genes. Mol Cell Probes. 2001;15(4):209–215.