ABSTRACT
Streptococcus mutans is recognized as the main cause of dental caries, and the formation of a plaque biofilm is required for caries development. This study aimed to determine the inhibitory effect of Lactobacillus plantarum FB-T9 on S. mutans biofilm formation in vitro and on the prevention and treatment of dental caries in rats. During in vitro experiments, FB-T9 exhibited good bacteriostatic ability in a plate competition assay. This strain also significantly reduced the biomass and viability of S. mutans biofilms and induced structural damage during the early (6 h), middle (12 h) and late (24 h) stages of biofilm formation. In a 70-day in vivo experiment, FB-T9 significantly reduced the levels of S. mutans on the dental surfaces of rats by more than 2 orders of magnitude of the levels in the dental caries model group (p < 0.05). Moreover, FB-T9 significantly reduced the caries scores (modified Keyes scoring method) in both the prevention and treatment groups (p < 0.05) and had great colonization potential in the oral cavity. These results indicate the potential usefulness of L. plantarum FB-T9 as a probiotic for the prevention and treatment of caries.
Introduction
Caries is a common type of oral bacterial infectious disease. According to the World Health Organization (WHO), caries is the third most common non-communicable disease after cancer and cardiovascular disease. According to data from a previous Lancet publication [Citation1], dental caries of the permanent teeth was the most prevalent disease afflicting humans. In 2016, caries in permanent teeth and caries in deciduous teeth ranked second and fifth, respectively, among the 10 diseases with the highest global incidence.
The formation of a plaque biofilm of bacteria is a prerequisite for the occurrence of a caries. A mature dental plaque biofilm is a three-dimensional micro-ecological environment comprising various bacteria embedded in a matrix mainly composed of water-insoluble polysaccharides with a certain thickness. In a normal dental plaque biofilm, oral microflora exist in a dynamic equilibrium. When host, dietary or microbial growth factors alter the ecological balance, acidifying bacteria in the plaque decrease the pH of the biofilm environment. Subsequently, the demineralization–remineralization balance in the tooth shifts toward mineral loss, leading to tooth decay [Citation2]. Streptococcus mutans, which has strong acid-producing and acid-resistant capacities, has been recognized as the main type of cariogenic bacteria [Citation3]. A large number of extracellular polysaccharides synthesized by S. mutans are important to the complex tri-dimensional structure of a dental plaque. Indeed, many studies have reported the relationship between the presence of S. mutans, biofilm formation and the related risk of caries [Citation4–Citation6].
Currently, dental plaque is mainly controlled and prevented using mechanical removal techniques and antimicrobial agents. However, tooth brushing, the most common mechanical plaque removal method, cannot fundamentally reduce the number of cariogenic bacteria such as S. mutans. Indeed, the applications of some mechanical treatments in pediatric and elderly populations are limited by personal preferences, and the effects are relatively superficial. The use of antibiotics is also limited by the close structure of dental plaque biofilm that makes the bacteria embedded in this complex community more resistant to antibiotics compared with the planktonic state. Accordingly, the concentration of antibiotics required to kill bacteria in a biofilm is hundreds of times higher than that required to kill planktonic bacteria [Citation7]. In addition, the long-term use of antibiotics may destroy the balance of the oral ecosystem and increase bacterial resistance. Therefore, research into caries prevention and treatment fundamentally relies on a safe and effective caries prevention method.
Probiotics have long been thought to contribute to human gastrointestinal health. To date, studies have confirmed that probiotics can help to prevent and treat diarrhea due to rotavirus infection, antibiotic use, ulcerative colitis and pouch enteritis [Citation8,Citation9]. Probiotics can also prevent respiratory tract infections and osteoporosis in middle-aged women, regulate the balance of oral flora and prevent dental caries, periodontitis and other oral diseases [Citation10–Citation12].
Previous studies have demonstrated that Lactobacillus could inhibit the growth of S. mutans biofilm and caries-related multispecies biofilms in vitro [Citation13–Citation15]. Clinical data have revealed the ability of some probiotics to colonize the oral cavity [Citation16] and significantly reduce the oral population of S. mutans. Lee and Kim found that Lactobacillus rhamnosus LGG suppressed S. mutans biofilm formation by reducing glucan production and antimicrobial activity [Citation17]. Skim soy milk fermented with L. paracasei subsp. paracasei NTU101 could considerably reduce the population of S. mutans in rats and inhibit the development and deterioration of dental caries [Citation18]. Hence, the screening of candidate oral probiotics based on their abilities to inhibit S. mutans growth and biofilm formation may represent an alternative means of preventing tooth decay.
In our previous study, L. plantarum FB-T9 isolated from healthy human feces exhibited an excellent antibacterial ability against S. mutans. In this study, we evaluated the antibacterial ability of L. plantarum FB-T9 on a half-strength plate and investigated the effect of this strain on S. mutans biofilm formation over different time periods. We also examined oral S. mutans colonization and caries formation in rats using bacterial counts and caries scores to evaluate the effect of L. plantarum FB-T9 on the prevention or treatment of caries in vivo. These results will support and inform the development of probiotics to prevent caries.
Materials and methods
Bacterial strains
L. plantarum FB-T9 was isolated from healthy human feces and inoculated in De Man, Rogosa and Sharpe (MRS) broth (DifcoTM, Detroit, MI, USA) under anaerobic conditions at 37°C. S. mutans ATCC 25,175 was purchased from the China Common Microbial Species Preservation and Management Center (CGMCC, Beijing China) and cultured in Tryptic Soy Broth (TSB, DifcoTM, Detroit, MI, USA) under anaerobic conditions at 37°C. Another strain, L. plantarum 5D-3 (5D-3), was selected from a healthy oral cavity and used as a control strain in animal tests. All strains were frozen in 30% (v/v) glycerol broth at −80°C and routinely streaked on corresponding ager plates. The plates were cultured in an anaerobic incubator (Electrotek AW500SG, England) at 37°C for 48 h. A single colony was inoculated into 5 mL corresponding broth tubes anaerobically at 37°C for 16 h and sub-cultured twice using 2% (v/v) inoculum in 5 mL broth before use.
Competition assay on plate
The experimental method broadly followed Tong et al. [Citation19]. After 16 h culture in broth tubes, the bacterial cultures were centrifuged at 3000 × g for 10 min and washed twice with sterile saline solution. The bacteria were then re-suspended in saline solution and diluted to a suspension with 1 × 105 living cells by colony counting prior to use. FB-T9 and S. mutans (10 µL of each) were inoculated on tryptic soy ager plates (TSA) or half-strength TSA (1/2TSA) simultaneously or sequentially. Under the former condition, 10 µL of both strains suspension was inoculated simultaneously and co-cultured at 37°C for 32 h. Under the latter condition, 10 µL of the first colonizer (FB-T9/S. mutans) was inoculated and grown for 16 h, after which 10 µL of the second colonizer (S. mutans/FB-T9) was inoculated near to the first organism and incubated for another 16 h. All the plates were cultured anaerobically at 37°C.
Biofilm formation assay
A biofilm formation assay was conducted as proposed by Loo, with slight modification [Citation20]. After 16 h culture, FB-T9 and S. mutans were centrifuged and re-suspended in corresponding medium supplemented with 0.2% sucrose, then diluted to a concentration of 1 × 105 CFU/mL by colony counting as described above. Next, a 100-μL aliquot of S. mutans suspension was added to each well of a 96-well microplate (Costar, USA) and incubated at 37°C for 24 h. To test the intervening effects of FB-T9 on the formation of the S. mutans early-stage biofilm, 40 μL of FB-T9 suspension were added to each well at 0, 6 or 12 h and cultured to a total time interval of 24 h. To assess the effects of FB-T9 on S. mutans middle- and mature-stage biofilms, S. mutans was initially cultured for 24 or 48 h. After removing the supernatant, 200 μL of FB-T9 suspension was added, and the cultures were incubated for another 24 h. Subsequently, planktonic bacteria were gently aspired from the microplates, which were rinsed with sterile physiological saline solution. Each well was then stained with 200 μL of crystal violet for 30 min at room temperature. After two rinses with saline solution, 100 μL of 95% alcohol was added to release the dye. Biofilm formation was then quantified by measuring optical density at 600 nm (OD600) in each well using a microplate reader (BioTek, Winooski, VT, USA). The negative control group was treated with the same volume of saline instead of FB-T9 suspension.
Biofilm viability assay
An in vitro biofilm model was established using a cover slide as carrier [Citation21]. A sterile cover glass was placed in a glass Petri dish with a 6-cm diameter, to which 4 mL of a S. mutans suspension was added. At 0, 6 or 12 h, 200 μL of FB-T9 suspension was added to the dish, and the culture was incubated up to a total of 24 h. Otherwise, a S. mutans biofilm was allowed to form for 24 or 48 h, after which 4 mL of FB-T9 suspension was added. The culture was then incubated for another 24 h before the biofilm was washed twice with phosphate-buffered saline. Finally, the glass slides were removed and subjected to fluorescence staining as described by Khan [Citation22]. Carboxyfluorescein diacetate, succinimidyl ester (CFDA-SE, 65-0850-84, InvitrogenTM) was used to label all viable bacteria with green fluorescence, while propidium iodide (PI, P3566, InvitrogenTM) was used to label in red all bacteria with damaged membranes (i.e., non-viable bacteria). The biofilm structure was observed using a confocal laser scanning microscope (CLSM; LSM 710, Zeiss, Germany), and the Z-stack analysis was performed using Zen 2010 software (Carl Zeiss, Germany) [Citation23]. The areas of viable and non-viable bacteria and the biofilm thickness were recorded. The biofilm activity was calculated as the percentage of viable bacteria. All control groups were administered an equivalent volume of saline instead of FB-T9 suspension.
Caries reduction in vivo
Animals and general procedures
Female SPF Wistar rats (21 days old) were purchased from Charles River Laboratories (Beijing, China) and reared with approval according to the guidelines of the Animal Care and Use Committee at the Jiangsu Institute of Parasitic Diseases. And the procedures were carried out in accordance with European Community guidelines (Directive 2010/63/EU) for the care and use of experimental animals. All of the rats were allowed to adapt to the diet and environment for 3 days and were then divided randomly into eight groups, comprising three treatment groups, three prevention groups and one group each for the naïve controls and caries models. A flow chart of group allocation is shown in . The rats in the dental caries control group (C) were subjected to 5 consecutive days of dental caries modeling. Briefly, after 16 h culture, S. mutans cultures were centrifuged and diluted to 108 CFU/mL in saline solution. A sterile cotton swab saturated with this bacterial suspension then applied to the rat oral cavity for 15 s per quadrant, as described by Beiraghi et al. [Citation24]. On day 6, oral swabs were spread on mitis salivarius agar plates supplemented with 200 μg/mL streptomycin sulfate (MMS, DifcoTM, Detroit, MI, USA) to confirm S. mutans colonization in the oral cavity. The intervention groups were treated with chlorhexidine (0.02%) (T1), FB-T9 (T2) or 5D-3 (T3) for another 5 consecutive days after S. mutans colonization and thrice weekly thereafter. The prevention groups (P1 0.02% chlorhexidine treated; P2 FB-T9 treated; and P3 5D-3 treated) were first colonized with 108 CFU/mL of lactobacilli for 5 consecutive days and then infected with S. mutans for another 5 consecutive days. The caries-free control group (CF) consumed a regular diet and water throughout the 10-week experimental period. All of the other groups were fed a cariogenic diet 2000 (obtained from Nantong Trophy Feed Technology Co., Ltd.) supplemented with a 5% (w/v) sucrose solution.
Microbial analysis and caries scoring
The rats were weighed daily to monitor growth in each group. During the experiment, the rats were sampled on days 6, 13 and 70. The collected samples were plated on two types of agar [Tanzer et al., 2010]: MMS agar plate was used to enumerate S. mutans against streptomycin, while MRS supplemented with 12 μg/mL vancomycin was used to enumerate lactobacilli [Montella et al., 2013]. At the end of the 10-week experimental period, the rats were anesthetized and sacrificed. The heart, liver, spleen, lungs and kidneys were harvested and weighed. After decapitation, the skull was removed and placed in an autoclave at 121°C for 15 min. The attached soft tissue was peeled off with a scalpel, and the jaw was cleaned and dried at room temperature. All of the specimens were immersed in a 0.4% ammonium purpurate staining solution for 12 h, rinsed and semi-sectioned along the occlusal surfaces of maxillary and mandibular molars using a diamond cutter (thickness: 0.1 mm). Caries on the rat molars was observed and evaluated under a stereomicroscope according to the caries diagnosis and scoring method reported by Keyes [Citation25].
Statistical analysis
SPSS Statistics 22.0 (SPSS, Inc., Chicago, IL, USA) was used for the analysis. A single factor variance was used to analyze the experimental results (one-way analysis of variance). A p-value <0.05 was considered to indicate a statistically significant difference. Origin Pro 8.5 was used to map and analyze the data.
Results
Antagonism between L. plantarum and S. mutans on a growth plateFB-T9
A plate competition experiment was used to evaluate the competitive effects of FB-T9 and S. mutans in an in vitro environment with limited space and nutrients. Both FB-T9 and S. mutans grew well on TSA and 1/2TSA plates (–). When FB-T9 and S. mutans were spotted on TSA plates simultaneously, the growth of S. mutans was suppressed near the FB-T9 colony (), but this suppression was not obvious on 1/2TSA (). Interestingly, the growth of S. mutans was inhibited severely when FB-T9 was inoculated first on both types of media (–). However, no obvious competitive inhibition was observed when S. mutans was inoculated first (–), indicating that although FB-T9 could inhibit S. mutans under either nutrient-rich or nutrient-deficient conditions, S. mutans had no inhibitory effect on FB-T9.
Figure 2. The inhibition effect of L. plantarum FB-T9 on S. mutans. (A and B) L. plantarum FB-T9 on TSA and 1/2 TSA plates; (C and D) S. mutans on TSA and 1/2TSA plates. Competition between FB-T9 and S. mutans: (E, G, I) on TSA plates; (F, H, J) on 1/2 TSA plates; (E and F) S. mutans and L. plantarum FB-T9 were inoculated on the plates at the same time; (G and H) L. plantarum FB-T9 was first inoculated on the plates; (I and J) S. mutans was first inoculated on the plates
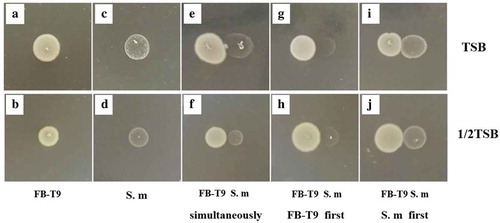
S. Mutans biofilm formation mediated by FB-T9 in vitro
According to a report by Nobbs and colleagues [Citation26], the formation of an early biofilm of S. mutans can be observed at 24 h. The formation period includes five key time points: 0 h, initial bacterial adherence; 6 h: initial bacterial colonization; 12 h: initial early biofilm formation; 24 h: maturation of early-stage biofilm and 48 h: maturation of the later-stage biofilm. In this study, we added the FB-T9 fermentation supernatant at these five time points to mediate the formation of the S. mutans biofilm. The S. mutans biofilm biomass, total number of bacteria in the biofilm and viability of biofilm were recorded under different experimental conditions.
The biomass of the S. mutans biofilm increased gradually from 0 to 48 h (). The addition of FB-T9 at 0, 6 and 12 h significantly influenced the formation of the biofilm (p < 0.05). The most obvious decreases were observed at 0 and 6 h. In particular, the S. mutans biomass at 6 h was only a third of the control value. Moreover, the addition of FB-T9 at 24 and 48 h significantly inhibited the S. mutans biofilm biomass (p < 0.05).
Figure 3. Biomass of S. mutans mediated by L. plantarum FB-T9 at different stages. C12, biofilm of negative control at 12 h. C24, biofilm of negative control at 24 h. C48, biofilm of negative control at 48 h. *P < 0.05 when compared with the control treatment and compared between groups
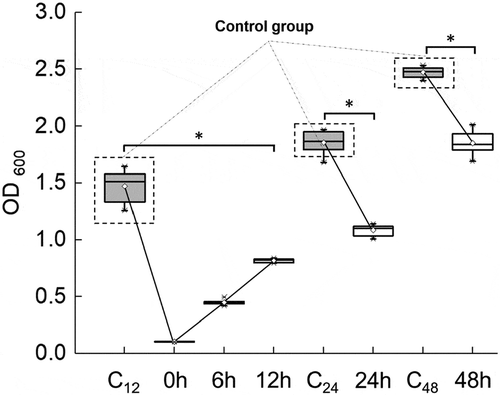
CLSM was used to visualize viable and non-viable bacteria. When FB-T9 was added to the S. mutans suspension at 0 h, no attached bacteria were observed after a 24-h culture (–). Meanwhile, the areas of bacteria in the biofilms of cultures treated at 6 and 12 h increased gradually, indicating that the biofilms accumulated gradually before the intervention (–). Additionally, the biofilms formed in the negative control group cultures (24 and 48 h) were dense and concentrated, with large areas containing viable bacteria (). In the cultures treated at 24 and 48 h, larger proportions of non-viable bacteria were observed, and biofilm structure was relatively loose and dispersed (). Visually, no obvious differences were observed between the control and mediation groups at 24 and 48 h. Hence, the total bacterial area and biofilm viability were calculated.
Figure 4. CLSM of S. mutans biofilm on glass coverslips. Addition of FB-T9 bacterial suspension at 0 h (A and B), 6 h (C and D), 12 h (E and F), 24 h (H and L) and 48 h (J and N) respectively. (G and K) S. mutans biofilm after incubation for 24 h with medium alone. (I and M) S. mutans biofilm after incubation for 48 h with medium alone. And A, C, E, G, H, I, J are corresponding 3D graphs
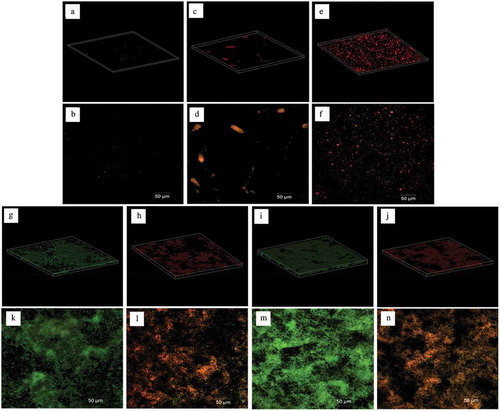
The bacterial areas of the early-treated (0, 6 and 12 h) biofilms ranged from 0.33 to 5.34 × 104 μm2 (), while that of the 12-h negative control biofilm was 16.08 × 104 μm2. The bacterial areas of the treated biofilms were significantly smaller than that of the control biofilm (p < 0.05), representing a reduction of more than 65%. Furthermore, the medium-term (24 h) bacterial area decreased by more than 50% after treatment. In the later-stage of biofilm maturation, the total bacterial areas in the 48-h mediation groups decreased only slightly when compared with the 48-h negative control, and this difference was not significant (p = 0.063). Interestingly, the viability of the biofilm decreased gradually with the time interval after the intervention, whereas the value of the negative control increased gradually. These results indicate that earlier FB-T9 mediation led to a better inhibitory effect on S. mutans biofilm formation (p < 0.05).
Figure 5. Total bacterial area of biofilm and biofilm viability after intervention by L. plantarum FB-T9 at different stages. The left axis corresponded to the histogram, showing the bacterial area of biofilm (×104 μm2). Biofilm mediated by FB-T9 at 0 h, 6 h, 12 h was compared to the 12 h negative control, while biofilm mediated by FB-T9 at 24 h and 48 h were compared to the 24 h and 48 h negative control, respectively. The dashed line indicated the biofilm viability of three controls, and the solid line indicated the biofilm viability after treatment at different stages. C12, biofilm of negative control at 12 h. C24, biofilm of negative control at 24 h. C48, biofilm of negative control at 48 h. The assay was performed three times and data are expressed as mean ± standard error of the mean. *P < 0.05 when compared with the control treatment and compared between groups
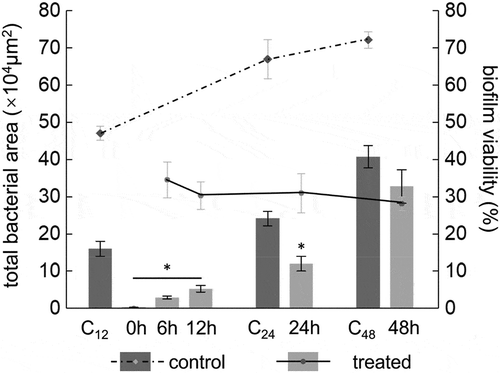
Evaluation of a caries-induced rat model
Bacterial colonization of the rat oral cavity
No significant differences in weight gain and visceral coefficients were observed between the groups of rats (p > 0.05, data not shown), and all animals appeared to be in good physical condition throughout the experiment. The concentrations of S. mutans on the molars of the dental caries model group and the intervention group ranged from 1 to 3 × 105 CFU/mL (), indicating that S. mutans had successfully colonized the oral cavities after 5 days of infection. When chlorhexidine was applied for another 5 days (second sampling), the S. mutans concentration decreased to 0.1 × 105 CFU/mL and stabilized at 0.2 × 105 CFU/mL at the end of the treatment (third sampling). However, population of S. mutans after FB-T9 treatment decreased two orders of magnitude from 3.2 × 105 to 0.03 × 105 CFU/mL (third sampling) which showed a greater extent than chlorhexidine (p < 0.05). Interestingly, treatment with 5D-3 also reduced the population of S. mutans, although it could not inhibit biofilm formation in vitro (data unpublished). Briefly, no significant differences in the effects of the interventions were observed between the three groups at the second and third samplings.
Table 1. S. mutans counts from rat dental samples at different periods
To evaluate the preventive effects of lactobacilli, rats were initially inoculated with lactobacilli for 5 days and then continuously infected with S. mutans for 5 days. Compared with the initial S. mutans infection (), the S. mutans population in prevention groups decreased by 1–2 orders of magnitude (), second sampling). In particular, the population of S. mutans in the FB-T9 prevention group (P2) was significantly lower than that in the other two groups (p < 0.05) at the second sampling and remained at the same level after 2 months (third sampling). In contrast, the number of S. mutans in the other two prevention groups increased significantly (p < 0.05) during the experiment (P1, P3).
Figure 6. S. mutans & lactobacilli count from rat dental samples at different periods in prevention groups. (A) S. mutans counts; (B) lactobacilli counts; Data are expressed as mean ± standard error of the mean (n = 8)
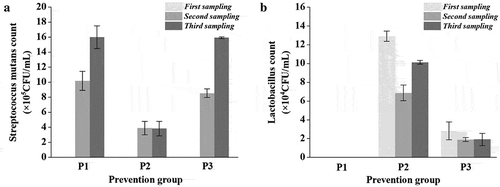
Initially, FB-T9 colonized the oral cavity to a concentration of approximately 105 CFU/mL ()), which was significantly higher than that induced by 5D-3 (104 CFU/mL). Meanwhile, the number of FB-T9 initially decreased in the second sampling but increased in the third sampling. It was speculated that FB-T9 may successfully colonize the oral environment via a competitive process. However, 5D-3 did not undergo a similar process, indicating that FB-T9 was generally retained better than 5D-3 in the rat oral environment.
Caries lesion evaluation by Keyes scoring
The cheek, tongue and adjacent surfaces and sulcal of a rat molar can be divided into several dental caries units according to the extent of caries damage, as shown in . Whereas demonstrates how the dental caries units were determined and scored, the score results for the corresponding caries are shown in . Dentin caries (Dm) occurred in the model and treatment groups but not in the prevention groups, whereas deep dentin caries (Dx) did not occur in any experimental groups. The FB-T9 prevention group (P2, 14.7) and intervention group (T2, 23.9) received the two lowest total dental caries scores (E; 14.7 and 23.9, respectively). Both of these scores were less than half of the score in the model group, indicating that FB-T9 significantly prevented and treated dental caries in vivo. Overall, caries reduction was more efficient in the prevention groups than in the intervention groups. However, the effect in the 5D-3 prevention group (P3) did not surpass that in the chlorhexidine prevention group (P4), and 5D-3 (T3) had no significant treatment effect (p > 0.05). Interestingly, the Dm score in the FB-T9 group was significantly lower than that in the model group (p < 0.05), whereas no significant difference was observed between the chlorhexidine and model groups (p > 0.05). FB-T9 appeared to have a better therapeutic effect with respect to deep caries, whereas chlorhexidine had a more superficial therapeutic effect.
Table 2. Number of linear units assigned to each molar
Table 3. Effect of different groups on caries development (incidence and severity) in rats
Discussion/conclusion
Dental caries is associated closely with cariogenic bacterial species, such as S. mutans. Although it is impossible to eradicate such microorganisms completely, interest in the potential use of probiotics to prevent oral caries has been increasing in recent years. The inhibitory effects of probiotics against S. mutans may be due to antimicrobial substances produced by probiotics, including organic acids, hydrogen peroxide, bacteriolytic enzymes, bacteriocins and biosurfactants [Citation27,Citation28]. Organic acids and hydrogen peroxide can enable probiotics to tolerate certain acidic conditions and to survive in an oral environment containing a certain amount of lysozyme [Citation3]. According to , when FB-T9 was spotted first on a culture plate, it had a stronger inhibitory effect on S. mutans than when S. mutans was spotted first, implying that substances produced by FB-T9 may contribute to its effects against S. mutans.
Oral cavity is a nutrient fluctuation environment which creates scarce resources such as nutrients and space for bacteria, so they are more likely to metabolize some inhibitory substances to suppress the surrounding microorganisms to ensure their nutritional needs and survival, which involves a mechanism called quorum sensing [Citation29,Citation30]. Nutritional limitations and environmental fluctuations stimulate the synthesis of signal molecules in bacterial cells. When the concentrations of these signal molecules reach a threshold, appropriate target genes are transcribed and used to produce active substances, thus inhibiting competitive bacteria [Citation31,Citation32]. Kreth et al. [Citation33] observed that the nutritional richness can interfere with the inhibitory capacity of Streptococcus sanguinis against S. mutans. In general, under nutrient-rich conditions, the magnitudes of inhibition were higher than under nutrient-limited conditions, except for a few species in specific environmental conditions. One can consider that under nutrient-limited conditions, the energy expenditure of the bacteria was employed for bacterial survival and not for the production of H2O2 [Citation34]. In our study, we use 1/2 TSA to simulate the condition of nutrient deficiencies and observed the interaction between S. mutans and Lactobacillus. The result showed that FB-T9 not only exerted a strong inhibitory effect on S. mutans, the main cariogenic bacteria, but also exhibited a strong competitive advantage under nutrient-deficient conditions. Therefore, we may infer that FB-T9 is a strong competitor against S. mutans for temporal and spatial niches.
Dental caries is well established as a representative biofilm-dependent oral disease. Although S. mutans is not always the most abundant bacteria in the oral cavity, it is a key producer of matrix and coordinator of cariogenic biofilm formation [Citation35]. Many studies have proven the effects of Lactobacillus on S. mutans biofilms at 24 h [Citation36–Citation38]. To date, however, the mechanism by which Lactobacillus interferes with the formation of S. mutans biofilms at different stages and the correlation between the biofilm biomass and activity at different stages remains unknown. In this study, S. mutans biofilm formation was mediated by FB-T9 at five key time points (0, 6, 12, 24 and 48 h), as described above. The following key indicators were used: (i) crystal violet staining to characterize the biofilm biomass, (ii) total bacterial area and proportions of viable and non-viable bacteria and (iii) biofilm structure. The results showed that earlier mediation yielded better effects against the biofilm. In addition, almost no biofilm formation was observed at 0 h mediation (-), possibly because the initial adhesion of S. mutans was inhibited by Lactobacillus. FB-T9 remained effective even against an initially maturing or already mature S. mutans biofilm, as demonstrated by the inactivation of all bacteria in the mediated biofilm. Lin et al. [Citation15] also used fluorescence staining to determine the viability of S. mutans biofilm after the intervention of five Lactobacillus strains, which indicated the inhibitory effect of five Lactobacillus strains on S. mutans biofilm. Moreover, the structure of the mediated biofilm in our study was looser than that of the control, suggesting that Lactobacillus had a significant effect at 48 h (). We speculated that although S. mutans had formed a mature biofilm at 48 h, Lactobacillus inhibited the proliferation of S. mutans in the dense biofilm and destroy the dense, cross-linked biofilm structure. Many biofilm structures contain channels through which environmental fluids can move. These channels often act as transport vessels to deliver nutrients, remove waste products and serve as conduits for messenger molecules [Citation39]. Destroying the biofilm structure will affect the physiological activity of the biofilm. The analysis of CLSM suggested that the effect of biofilm inhibition is not necessarily to reduce the bacterial area of biofilm, but also to destroy the structure of biofilm and reduce its viability.
The inhibitory effects of Lactobacillus on cariogenic bacteria and biofilm formation observed in vitro do not necessarily mean that Lactobacillus has the same effects in vivo. Therefore, this study further examined the anti-caries activity of FB-T9 in a rat model of dental caries. L. plantarum 5D-3 that exhibited poor inhibitory activity against biofilms in previous experiments (data unpublished), was selected as control. During a 70-day experimental period, S. mutans counts and caries scores used to evaluate the decay process demonstrated that the number of S. mutans decreased from 105 to 103 CFU/mL after intervention (). In each intervention group, the number of S. mutans differed significantly in the second and third sampling related to the first sampling (p < 0.05), indicating significant reductions in the population of S. mutans. In particular, no S. mutans was detected in the second and third samplings after FB-T9 mediation. In , the number of S. mutans after FB-T9 colonization was significantly lower than the number in the other two groups, suggesting that FB-T9 had more effectively prevented the colonization of S. mutans than had chlorhexidine and 5D-3. Meanwhile, the FB-T9 prevention group received the lowest caries score of all of the groups (). As shown in ), FB-T9 appeared to better prevent caries because of its strong ability to colonize the rat oral cavity. Previous studies showed that L. rhamnosus SD11 was detected in large quantities in the oral cavity even 4 weeks after the cessation of consumption and had a significant inhibitory effect on the population of S. mutans in the oral cavity [Citation40,Citation41], which is similar to our findings. The ability of a probiotic to colonize the oral cavity is key to its function, and this ability is strain-specific. For example, 5D-3 may effectively prevent biofilm formation, perhaps because it had the advantage of pre-colonization of the oral cavity (,b) and ). Hence, the ability of Lactobacillus spp. to colonize oral cavity should be considered an indicator of oral probiotics. FB-T9 exhibited excellent efficacy in both the prevention and treatment groups and demonstrated that a potential probiotic could outperform chlorhexidine in the treatment of dental caries (). Current studies have shown that probiotic products can reduce the population of S. mutans in the human oral cavity [Citation42,Citation43].
In conclusion, this study enabled us to identify L. plantarum FB-T9 as a highly suitable probiotic. This bacterium antagonized S. mutans in vitro, inhibited biofilm formation and reduced viability in the biofilm at different stages of formation. Moreover, the in vivo animal experiments revealed that FB-T9 could significantly reduce the population of S. mutans in the rat oral cavity, as well as the caries score. It appears that L. plantarum FB-T9 is a potential new oral probiotic, which can be further developed into a probiotic preparation with practical application value to prevent dental caries.
Disclosure statement
No potential conflict of interest was reported by the authors.
Additional information
Funding
References
- Vos T, Abajobir AA, Abate KH, et al. Global, regional, and national incidence, prevalence, and years lived with disability for 328 diseases and injuries for 195 countries, 1990–2016: a systematic analysis for the Global Burden of Disease Study 2016. Lancet. 2017;390(10100):1211–10.
- Featherstone JD. The continuum of dental caries–evidence for a dynamic disease process. J Dent Res. 2004;83(83 Spec No C):39–42.
- Simón-Soro A, Mira A. Solving the etiology of dental caries. Trends Microbiol. 2015;23(2):76–82.
- Bowen WH, Koo H. Biology of Streptococcus mutans-derived glucosyltransferases: role in extracellular matrix formation of cariogenic biofilms. Caries Res. 2011;45(1):69–86.
- Mashima I, Nakazawa F. The interaction between Streptococcus spp. and Veillonella tobetsuensis in the early stages of oral biofilm formation. J Bacteriol. 2015;197(13):13–54.
- Huang R, Li M, Gregory RL. Bacterial interactions in dental biofilm. Virulence. 2011;2(5):435–444.
- Ready D, Roberts AP, Pratten J, et al. Composition and antibiotic resistance profile of microcosm dental plaques before and after exposure to tetracycline. J Antimicrob Chemother. 2002;49(5):769.
- Guandalini S. Probiotics for prevention and treatment of diarrhea. J Clin Gastroenterol. 2011;45:S149–S153.
- Floch MH. The role of prebiotics and probiotics in gastrointestinal disease. Gastroenterol Clinics. 2018;47(1):179–191.
- Gruner D, Paris S, Schwendicke F. Probiotics for managing caries and periodontitis: systematic review and meta-analysis. J Dent. 2016;48:16–25.
- Khani S. M, Hosseini H, Taheri M et al. Probiotics as an alternative strategy for prevention and treatment of human diseases: a review. Inflamm Allergy Drug Targets. 2012;11(2):79–89.
- Montazeri-Najafabady N, Ghasemi Y, Dabbaghmanesh MH, et al. Supportive role of probiotic strains in protecting rats from ovariectomy-induced cortical bone loss. Probiotics and antimicrobial proteins. 2018. p. 1–10.
- Ciandrini E, Campana R, Baffone W. Live and heat-killed Lactobacillus spp. interfere with Streptococcus mutans and Streptococcus oralis during biofilm development on titanium surface. Arch Oral Biol. 2017;78:48–57.
- Lin X, Chen X, Tu Y, et al. Effect of probiotic lactobacilli on the growth of streptococcus mutans and multispecies biofilms isolated from children with active caries. Med Sci Monit. 2017;23:4175–4181.
- Lin X. Chen X, Chen Y, et al. The effect of five probiotic lactobacilli strains on the growth and biofilm formation of Streptococcus Mutans. Oral Dis. 2015;21(1):e128–e134.
- Montalto M, Vastola M, Marigo L, et al. Probiotic treatment increases salivary counts of Lactobacilli: a double-blind, randomized, controlled study. Digestion. 2004;69(1):53–56.
- Lee SH, Kim YJ. A comparative study of the effect of probiotics on cariogenic biofilm model for preventing dental caries. Arch Microbiol. 2014;196(8):601–609.
- Lin TH, Pan TM. Inhibitory effect of Lactobacillus paracasei subsp. paracasei NTU 101 on rat dental caries. J Funct Foods. 2014;10:223–231.
- Tong H, Chen W, Merritt J, et al. Streptococcus oligofermentans inhibits Streptococcus mutans through conversion of lactic acid into inhibitory H2O2: a possible counteroffensive strategy for interspecies competition. Mol Microbiol. 2007;63(3):872–880.
- Loo C, Corliss D, Ganeshkumar N. Streptococcus gordonii biofilm formation: identification of genes that code for biofilm phenotypes. J Bacteriol. 2000;182(5):1374–1382.
- Lynch DJ, Fountain TL, Mazurkiewicz JE, et al. Glucan-binding proteins are essential for shaping Streptococcus mutans biofilm architecture. FEMS Microbiol Lett. 2010;268(2):158–165.
- Khan R. Zakir M, ., Khanam Z, et al. Novel compound from Trachyspermum ammi (Ajowan caraway) seeds with antibiofilm and antiadherence activities against Streptococcus mutans: a potential chemotherapeutic agent against dental caries. J Appl Microbiol. 2010;109(6):2151–2159.
- Wood SR, Kirkham J, Marsh PD, et al. Architecture of intact natural human plaque biofilms studied by confocal laser scanning microscopy. J Dent Res. 2000;79(1):21–27.
- Beiraghi S, Rosen S, Beck F. The effect of stannous and sodium fluoride on coronal caries, root caries and bone loss in rice rats. Arch Oral Biol. 1990;35(1):79–80.
- Keyes PH. Dental caries in the molar teeth of rats. II. A method for diagnosing and scoring several types of lesions simultaneously. J Dent Res. 1958;37(6):1088.
- Nobbs AH, Lamont RJ, Jenkinson HF. Streptococcus adherence and colonization. Microbiol Mol Biol Rev. 2009;73(3):407–450.
- Oelschlaeger TA. Mechanisms of probiotic actions–a review. Int J Med Microbiol. 2010;300(1):57–62.
- Wannun P, Piwat S, Teanpaisan R. Purification, characterization, and optimum conditions of fermencin sd11, a bacteriocin produced by human orally Lactobacillus fermentum SD11. Appl Biochem Biotechnol. 2016;179(4):572–582.
- Vendeville A, Winzer K, Heurlier K, et al. Making’sense’of metabolism: autoinducer-2, LuxS and pathogenic bacteria. Nature Rev Microbiol. 2005;3(5):383.
- Lekshmi M, Parvathi A, Kumar S, et al. Efflux pump-mediated quorum sensing: new avenues for modulation of antimicrobial resistance and bacterial virulence. Biotechnological Applications of Quorum Sensing Inhibitors. Springer; 2018. p. 127–142.
- McNab R, Lamont RJ. Microbial dinner-party conversations: the role of LuxS in interspecies communication. J Med Microbiol. 2003;52(7):541–545.
- Zhou J, Lyu Y, Richlen ML, et al. Quorum sensing is a language of chemical signals and plays an ecological role in algal-bacterial interactions. Crit Rev Plant Sci. 2016;35(2):81–105.
- Kreth J, Merritt J, Shi W, et al. Competition and coexistence between Streptococcus mutans and Streptococcus sanguinis in the dental biofilm. J Bacteriol. 2005;187(21):7193–7203.
- Herrero ER, Slomka V, Bernaerts K, et al. Antimicrobial effects of commensal oral species are regulated by environmental factors. J Dent. 2016;47:23–33.
- Bowen W, Koo H. Biology of Streptococcus mutans-derived glucosyltransferases: role in extracellular matrix formation of cariogenic biofilms. Caries Res. 2011;45(1):69–86.
- Wasfi R, Abd El‐Rahman OA, Zafer MM, et al. Probiotic Lactobacillus sp. inhibit growth, biofilm formation and gene expression of caries‐inducing Streptococcus mutans. J Cell Mol Med. 2018;22(3):1972–1983.
- Wu CC, Lin CT, Wu CY, et al. Inhibitory effect of Lactobacillus salivarius on Streptococcus mutans biofilm formation. Mol Oral Microbiol. 2015;30(1):16–26.
- Söderling EM, Marttinen AM, Haukioja AL. Probiotic lactobacilli interfere with Streptococcus mutans biofilm formation in vitro. Curr Microbiol. 2011;62(2):618–622.
- Wimpenny J, Manz W, Szewzyk U. Heterogeneity in biofilms. FEMS Microbiol Rev. 2000;24(5):661–671.
- Rungsri P, Akkarachaneeyakorn N, Wongsuwanlert M, et al. Effect of fermented milk containing Lactobacillus rhamnosus SD11 on oral microbiota of healthy volunteers: a randomized clinical trial. J Dairy Sci. 2017;100(10):7780–7787.
- Yli‐Knuuttila H, Snäll J, Kari K, et al. Colonization of Lactobacillus rhamnosus GG in the oral cavity. Oral Microbiol Immunol. 2006;21(2):129–131.
- Bafna HP, Ajithkrishnan C, Kalantharakath T, et al. Effect of short-term consumption of amul probiotic yogurt containing Lactobacillus acidophilus La5 and Bifidobacterium lactis Bb12 on salivary streptococcus mutans count in high caries risk individuals. Int J App Basic Med Res. 2018;8(2):111.
- Salem RG, Abd-El-Aziz AM, Erfan DM. Assessment of the effect of probiotic yoghurt and different probiotic strains on salivary Streptococcus mutans in children: an in vivo and an in vitro study. Egypt J Med Microbiol. 2016;38(103):1–7.