ABSTRACT
Atherothrombosis, leading to stroke and myocardial infarction, is responsible for most of the deaths in the world. An increased risk of atherothrombotic vascular events has been reported in patients with periodontitis. Periodontitis is a chronic multifactorial inflammatory disease, which involves a dysbiotic microbiota, and leads to a progressive destruction of the tooth-supporting apparatus. Transcient periodontal pathogen blood translocation, mainly bacteremia, has been associated with the severity of gingival inflammation. The identification of periodontal bacteria within atherothrombotic plaques is challenging and unpredictable. This review aims to summarize existing molecular technics for identifying periodontal microbiota in human atherothrombotic samples. A secondary objective is to describe a protocol for the identification of Porphyromonas gingivalis from highly calcified, atherothrombotic human samples that is based on our experience in translational cardiovascular research. Compared to direct real-time PCR, our protocol based on nested PCR has increased the detection of Porphyromonas gingivalis by 22.2% with good specificity.
Introduction
Atherothrombotic cardiovascular diseases have been linked to periodontitis primarily via associations with periodontal bacteria [Citation1]. Several hypotheses have been proposed to explain this link: (1) the direct role of bacteria or their byproducts (endotoxins, proteases, DNA), damaging the vascular wall and driving atherothrombotic plaque development [Citation2]; and (2) the role of the innate immune response to oral dysbiosis by establishing a prothrombotic state and intraplaque hemorraghes [Citation3,Citation4]. To decipher these mechanisms, many studies first investigated the presence of periodontal pathogens within human atherothrombotic plaques using techniques based on cellular and molecular biology [Citation5]. Although the identification of periodontal microbiota in other less complex types of human samples, like subgingival swab and blood, are now well established [Citation6], detection of periodontal bacteria within atherothrombotic plaques remains challenging, because of the characteristics of the pathological tissue highly enriched with oxidation, protease activity and cellular death leading to haemorraghes and calcifications.
Techniques based on amplification of the 16S rRNA bacterial gene by polymerase chain reaction (PCR) offer large scale applications in clinical vascular samples [Citation7]. Given the variety of PCR protocols used for identification of periodontal bacteria in atherothrombotic plaque samples, the outcomes across human studies show a wide range of heterogeneity [Citation5]. This review aims to summarize existing molecular protocols for identifying periodontal microbiota in human atherothrombotic samples. A secondary objective is to describe a protocol for the identification of Porphyromonas gingivalis from human highly calcified atherothrombotic samples, which is based on our experience in translational cardiovascular research.
Polymerase chain reaction
All of the PCR steps and reagents are points of potential variability that lead to different results across clinical studies that focus on identifying periodontal bacteria in human atherothrombotic plaques. Thus, we analyzed the literature regarding the charatecteritcs of the samples and the PCR protocols used (–). With regards to the sample sites, 13 studies used the carotid [Citation2,Citation4,Citation8–18], 8 the coronary [Citation18–25] and 7 the anevrysmal wall [Citation10,Citation16,Citation26–30]. Other studies used various vascular samples. In 4 studies [Citation9,Citation20,Citation26,Citation31], they were immediately frozen after sampling then transported in liquid medium before being processed, or in the case of 18 studies, they were further stored until processing [Citation2,Citation4,Citation8,Citation11–14,Citation16–19,Citation21–25,Citation27,Citation28] and 6 studies did not address the processing methods [Citation10,Citation15,Citation29,Citation30,Citation32,Citation33]. Samples were immediately frozen after their transport in 2 studies [Citation11,Citation23], or kept at −20°C in 10 studies [Citation12,Citation14–16,Citation18,Citation20,Citation21,Citation24,Citation28,Citation33] or at −70°C or lower in 10 studies [Citation9–11,Citation23,Citation26,Citation27,Citation29–31,Citation33]. In 3 studies, samples were cultured [Citation8], embedded in paraffin [Citation32] or kept in conditioned medium [Citation4] before being frozen. Samples have been mechanically homogenized in only 8 studies [Citation4,Citation10,Citation14,Citation15,Citation22,Citation27,Citation29,Citation31]. For 7 studies [Citation8,Citation9,Citation12,Citation13,Citation23,Citation25,Citation26], homogenization was addressed although details were not provided and for 12 studies [Citation2,Citation11,Citation16–18,Citation20,Citation21,Citation24,Citation28,Citation30,Citation32,Citation33] no details on tissue homogenization were provided.
Table 1. Identification of periodontal pathogens in atherothrombotic samples using PCR methods
Table 2. Vascular sample preparation for identifying periodontal pathogens
Table 3. PCR conditions and identification methods for periodontal pathogens in atherothrombotic samples
None of the studies used the exact same PCR protocol and DNA extraction kit. Seventeen studies implemented conventional qualtitative PCR to amplify DNA [Citation8,Citation9,Citation11,Citation16,Citation18–20,Citation22–28,Citation31–33], 7 used quantitative PCR [Citation2,Citation4,Citation12,Citation17,Citation21,Citation29,Citation30] and 4 used nested PCR [Citation10,Citation13–15]. The median number of cycles was 36 with a big range (30 [Citation8,Citation11,Citation13,Citation31]–60[Citation30]). The results of the PCR were confirmed by gel only in 9 studies [Citation8,Citation9,Citation12,Citation14,Citation18,Citation20,Citation26,Citation27,Citation29], by DNA sequencing in 8 studies [Citation4,Citation13,Citation15,Citation19,Citation22,Citation24,Citation25,Citation32] and by melting curve analysis in 4 studies [Citation2,Citation17,Citation21,Citation30].
These elements, which will be discussed below, are all parameters of variability that can explain the different results observed. Indeed, Actinobacillus actynomyctemcomitans, Porphyromonas gingivalis, Tanerella forsythia and Prevotella intermedia have been found in 58.3%, 64.3%, 55.0% and 77.8% of the studies, but were detected in a broad range in 19.2% (0–100; median: 7.1), 31.1% (0–100; median: 20.8), 15.9% (0–100; median: 4.4), 30.4 (0–100; median: 17.5) of the samples, respectively.
The heterogeneity of the protocols did not highlight one specific parameter of variability, but rather it raised the issue of the importance of each of these variables. Consequently, although PCR is a well-known technique, a strict protocol must be followed that also takes into account the proteolytic and oxidative nature of the samples, leading to hemorrages and calcifications. The second part of this manucript describes every step of our protocol based on nested PCR, from the collection to the final identification of periodontal bacteria, which is prone to bias and can lead to variable results. We then compare it to the use of direct real-time PCR.
Microbial whole genome sequencing
Microbial whole genome sequencing (WGS), a high-throughput approach to DNA sequencing using the concept of massively parallel processing, also called next-generation sequencing (NGS), has been used to explore oral bacterial communities in atherothrombotic plaques. Interestingly, the microbial community observed in atherothrombotic samples is similar to that observed in subgingival samples [Citation34]. However, no periodontal species have been identified by WGS in vascular samples [Citation35] but they have been identified by WGS in other samples [Citation36].
Some studies also identified gut microbiome members within atherothrombotic samples using WGS, however, the results are mixed [Citation37]. WGS is a useful and highly sensitive technique. However, minor changes in laboratory protocols, in the preparation of the samples or in the analysis of the data can modify the results [Citation38]. Thus, given this sensitivity to minor changes, WGS may not be suitable for the identification of periodontal bacterial species in atherothrombotic samples. Consequently, although WGS is a very sensitive technique for characterizing bacterial community diversity in human samples, the analysis of the results must be considered carefully for periodontal species in atherothrombotic tissues.
Proposed protocol for amplification of periodontal bacteria by PCR
Based on the data from the literature reviewed above, and taking into account our experience with the identification of periodontal bacteria in animals [Citation29] and human atherothrombotic plaques [Citation4], we suggest that the presence of periodontal pathogens should be explored by using the protocol discussed below () and described in detail in the supplemental material, for Porphyromonas gingivalis amplification. Indeed, we considered each parameters of variability to propose a protocol observing the rules of molecular biology adapted to the specificity of atherothrombotic and higly calcified samples.
Figure 1. Proposed protocol for Porphyromonas gingivalis 16S rDNA amplification in human calcified atherothrombotic samples in 5 steps. Sterile equipment must be used for all the following steps. (1.) Samples must be transported to the laboratory in a cold (4°C) sterile solution within a few hours after harvesting. (2.) Then, samples must be processed with homogenization. A liquid nitrogen electromagnetic grinding method is preferred over a bead-beating method to maximize nucleic acid preservation. Samples can be stored at −80°C while waiting for homogenization, but freezing and thawing steps should be minimized. (3.) DNA extraction is carried out after pre-incubation with a bacterial lysis buffer using the PrepFiler BTA forensic DNA extraction kit (Life Technologies®) to remove calcifications. (4.) Then, nested PCR is carried out, with conventional PCR as the first step and real-time PCR as the second step (≤40 cycles). (5.) For quality control, the molecular data is obtained using agarose gel migration, melting curve comparison, and sequencing
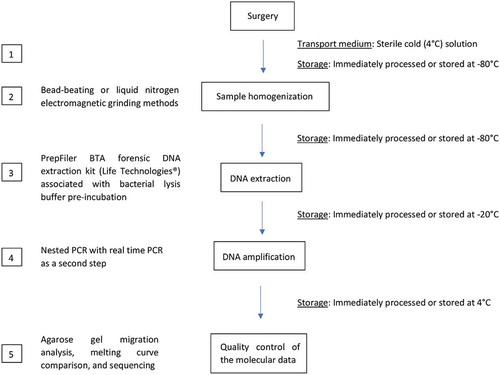
Vascular sample collection and preparation
summarises vascular sample preparation characteristics across studies exploring periodontal microbiota in atherothrombotic plaques. Besides transit and storage conditions, several other parameters may have a significant impact on DNA yield and quality. Little information is available on the cleaning of surgical specimens to prevent contamination althougt it is important. Given that DNA is denatured at room temperature, most of the studies indicate that samples are rapidly frozen at low temperature (from −20°C to −80°C). Details on the homogenization of the samples are scarce in the literature, although powerful mechanical grinding is necessary to release bacterial DNA. Bead beating [Citation39] and liquid nitrogen electromagnetic methods (cryopulverisation) [Citation29] appear to be those most suitable to give acces to the entire DNA and thus prevent false negative.
In our laboratory, the samples are stored at −80°C before being pulverized, using a freezer mill (Spex Certiprep Ltd) ensuring perfect homogenization of the samples without destroying the DNA by heating, which is not discussed in the reported studies. Briefly, the samples are placed one by one in a previously cooled tube containing a magnetic bar and then introduced into the grinding machine filled with liquid nitrogen. Importantly, tissue samples must be cleaned up under flow to avoid external contamination, then strongly homogenized before being processed. Ideally, to maintain optimal DNA integrity and yield and prevent its compromise due to thawing and tissue lysis, DNA extraction and the amplification of the targeted sequence by PCR must be performed just after sampling. However, this is not always possible in standard laboratory practice. Immediate storage at −80°C after freezing on dry ice or in liquid nitrogen is usally considered appropriate. However, freezing and thawing cycles must be kept to a minimum.
DNA extraction from atherothrombotic tissues
The DNA extraction method selected must be adapted for bacterial DNA extraction and to the specificity of the human tissue. Calcifications are a common macroscopic feature of atherothrombotic samples (Figure S1), which prevent optimal DNA extraction because they saturate and block the extraction column filter or interfere with free DNA [Citation40] or extraction reagents. This technical point is not addressed in the literature, and details are lacking on how to adjust for this limitation when using the DNA extraction kits (), while it is very important to limit false negative due to low yield. DNA yield and quality may be improved with commercial kits specifically designed for calcified tissues, like teeth or bone. For example, the PrepFiler BTA forensic DNA extraction kit (Life Technologies®) is meant for this type of application. In addition, considering human atherothrombotic samples, the bacterial DNA/human DNA ratio is low to very low, and this factor impairs the detection of bacterial DNA. Techniques for separating human DNA from bacterial DNA have been proposed and warrant more attention [Citation41]. This would make bacterial DNA amplification easier and more reliable, but (1) the yield would be low, (2) and the cost would be high. Therefore, some authors recommended the use of MoIYsis Complete5 kit (Molzym®) for bacterial DNA extraction from human tissues [Citation42]. In addition, bacterial characteristics of the sample should be considered, such as the presence of Gram + or Gram – bacteria. This consideration will help improve the isolation of the bacterial DNA from the tissue [Citation43]. For example, Gram-positive bacteria require pre-incubation with specific enzymes, such as lysozyme, to lyse the rigid multilayered cell wall. This can also be used with Gram-negative bacteria to ensure efficient DNA extraction.
Periodontal bacterial DNA amplification
Most studies implement conventional qualitative PCR to identify bacterial DNA, whereas others use real-time quantitative PCR or nested PCR (). All studies used previously published references but they often slightly modify the methodology, for example by customizing primers and/or PCR conditions.
Targeting specific 16s rRNA gene sequences for periodontal pathogens can be implemented with primers previously used in the literature or designed by dedicated software like Primer-BLAST. This tool is easy to use and it helps select custom primers that meet the specific requirements of the selected PCR protocol. However, these newly generated primers will not have been tested, unlike those published in the literature. Without a consensus on optimal primers, scientists must first systematically test the selected primers on known bacterial culture samples and on clinical subgingival bacterial samples. Once primers have been positively confirmed on standard samples, they can then be used for sequencing and comparison with a 16s rRNA gene database, like the one from the Ribosomal Database Project (RDP) [Citation44].
To improve bacterial detection, some studies increase the number of PCR cyles (>40) to increase the DNA copy number [Citation4,Citation21,Citation29,Citation30]. This approach is not recommended because it increases the risk of false positives, which decreases specificity. Some authors pooled duplicate or triplicate samples to increase the likelihood of detection [Citation34]. However, comparing results from duplicate or even triplicate samples instead of pooling, improves the sensitivity of bacterial identification. Based on our experience, we recommend using 3 dilutions of DNA extracts because human atherothrombotic plaque samples contain variable concentrations of bacterial DNA. This approach has been useful because we have found that at least one dilution is often positive for a given sample. This approach has not been described previously, although it impacts the PCR detection rate for periodontal pathogens, preventing false negative. In addition, since each Taq DNA polymerase and master mix have their own characteristics, testing at least 2 different commercial mixes is a good way to optimize the PCR conditions for the atherothrombotic plaque samples. Lastly, according to the primers and the PCR machine used, multiple PCR programs that call for different times and temperatures have been described in the literature (). For genomic DNA amplification, including bacterial DNA amplification, manufacturers recommend using standard protocols and not the fast programs present in newer devices.
Direct real-time PCR and nested PCR
Direct real-time PCR is a good technique for amplifying DNA. However, in our study, it showed poor results for the amplification of a specific fragment of the 16S rRNA gene of P. gingivalis in human atherosclerotic calcified samples. Indeed, only one sample out of 45 was positive (). When we tried to improve the sensitivity, by increasing the number of cycles of amplification from 40 to 50, we lost specificity ().
Figure 2. Images of agarose gels showing the migration of amplicons from an amplification by direct real-time PCR using primers designed for Porphromonas gingivalis. (a) PCR protocol using 40 cycles. (Lane 1:) Negative control. (Lane 2:) Positive control. (Lanes 3–13 and 15, 16:) Samples. (Lane 14:) Ladder. Only the last sample (lane 16) had an amplicon with the same molecular weight as the positive control. (b) PCR protocol using 50 cycles. (Lane 1:) Positive control. (Lanes 2–10 and 12:) Samples. (Lane 11:) Ladder. (Lane 13:) Negative control. All the samples present multiple amplicons
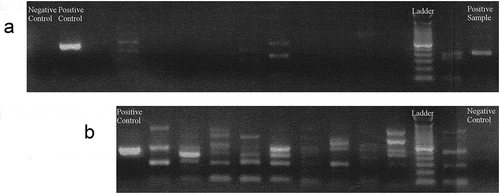
Nested PCR is a modification of the conventional PCR method, which consists of a succession of two PCRs, the second PCR uses the product of the first PCR as sample. Indeed, two sets of primers are used in the nested PCR protocol. The first set of primers (first PCR) amplifies large fragments by binding outside of the target DNA. The second set of primers (second PCR) binds specifically at the target DNA. It is intended to reduce non-specific binding by reducing the amplification of unexpected primer binding sites. Different methods have been described for purifying the PCR products from the first universal PCR (using 16S rRNA gene universal primers). One option is the Denaturing Gradient Gel Electrophoresis (DGGE) technique, which involves using a gel extraction kit after the amplified products have migrated on an agarose gel. Alternative options include using PCR purification kits or incubation with an enzyme that removes unincorporated primers and dNTPs. Use of the Illustra ExoProStar® (Dutcher) product for removing unincorporated primers and dNTPs is easier to implement compared to other methods, and was highly effective in our study.
The implementation of this protocol based on nested PCR has increased the sensitivity, without losing specificity (). Extra cost and time were moderate compared to the gain.
Figure 3. Images of agarose gel showing the migration of amplicons from an amplification by nested PCR (conventional PCR as the first step and real-time PCR as the second step) using primers designed for Porphromonas gingivalis. (Lane 1 and 9:) Ladder. (Lane 2:) Negative control. (Lane 3:) Positive control. (Lanes 4–8 and 10–15:) Samples. Samples from (lanes 4, 7, 11, 13, and 15) had an amplicon with the same molecular weight as the positive control. They were confirmed to be positive by comparison of the melting curves and by sequencing
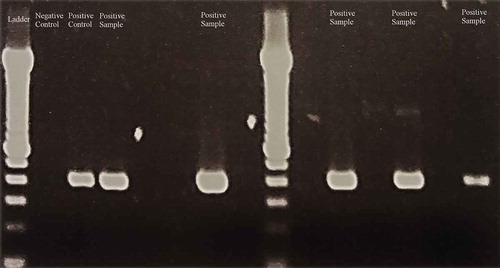
Based on our experience, nested PCR is a good approach for identifying periodontal pathogens in highly challenging samples, like atherothrombotic plaques, preventing false negative by increasing the sensitivity via a double amplification and false positive by reducing non-specific binding.
Quality control considerations
Evaluation of the migration of PCR products on agarose gels is a widely used method [Citation45]. However, this approach is inaccurate and is not reliable for identification of periodontal pathogens. Therefore, recent studies have added a sequencing phase to confirm their results (). Admittedly, this step represents additional cost and preparation time. An alternative option for greater quality control is using a comparative analysis of the melting curve. However, this technique may introduce error, since a slight variation in the profile of the melting curve may correspond to an aberrant PCR product (Figures S2 and S3). In our study, the 3 approaches were used to strengthen the identification of the bacteria. First, we compared the melting curves, then positive samples were loaded on agarose gel and, finally, positive samples were sequenced, preventing false positive, which is not the case for most of the reported studies. In summary, for reliable identification of periodontal pathogens in atherothrombotic plaques, PCR products must be sequenced.
Conclusion
Identification of periodontal microbiota in human atherothrombotic plaques is very important. Indeed, pathogens are central to the relationships that bind periodontitis to atherothrombosis [Citation46]. The detection of the 16 rRNA gene is a signature of the pathogen that colonized the atheromatous plaque at some point in the patient’s life, which means that the pathogen had been alive at one point and may be still be alive. This may result in oxidation and proteolysis, which further leads to plaque vulnerability [Citation2]. However, it remains highly challenging despite technical improvements. Heterogeneity in the published data may be linked to differences in sample characteristics, in sample collection and preparation, and the molecular-based techniques used for identification. False negatives and false positives may occur when inadequate methodological approaches and quality control measures are implemented, leading to an inacturate estimation of the presence of periodontal bacteria in atherothrombotic plaques. Identification of periodontal bacteria in human atherothrombotic samples should be performed carefully to avoid significant pitfalls. Standardization of the molecular techniques and protocols across laboratories and clinical teams are needed to improve the quality of reported data in the field of periodontitis and atherothrombotic cardiovascular disease research. Furthermore, WGS may not be suitable for species identification of periodontal bacteria in atherothrombotic samples. The PCR protocol described in this report may be a first step in standardizing these approaches, and thereby contributing to a consensus in the identification of periodontal pathogens in atherothrombotic samples, especially in highly calcified ones, preventing false positive and false negative biais.
Supplemental Material
Download Zip (5.5 MB)Acknowledgments
The authors thank Martine Bonnaure-Mallet for her advice and support.
Disclosure statement
No potential conflict of interest was reported by the authors.
Supplemental Material
Supplemental data for this article can be accessed here.
Correction Statement
*These authors contributed equally to this work and are considered as joint first authors.
This article has been republished with minor changes. These changes do not impact the academic content of the article.
Additional information
Funding
References
- Lockhart PB, Bolger AF, Papapanou PN, et al. Periodontal disease and atherosclerotic vascular disease: does the evidence support an independent association? Circulation. 2012;125(20):2520–10.
- Kozarov EV, Dorn BR, Shelburne CE, et al. Human Atherosclerotic Plaque Contains Viable Invasive Human Atherosclerotic Plaque Contains Viable Invasive Actinobacillus actinomycetemcomitans and Porphyromonas gingivalis. Arter Thromb Vasc Biol. 2005;25:17–18.
- Huck O, Elkaim R, Davideau J-L, et al. Porphyromonas gingivalis-impaired innate immune response via NLRP3 proteolysis in endothelial cells. Innate Immun. 2015;21:65–72.
- Rangé H, Labreuche J, Louedec L, et al. Periodontal bacteria in human carotid atherothrombosis as a potential trigger for neutrophil activation. Atherosclerosis. 2014;236:448–455.
- Reyes L, Herrera D, Kozarov E, et al. Periodontal bacterial invasion and infection: Contribution to atherosclerotic pathology. J Clin Periodontol. 2013;84:S30–S50.
- Sanz M, Lau L, Herrera D, et al. Methods of detection of Actinobacillus actinomycetemcomitans, Porphyromonas gingivalis and Tannerella forsythensis in periodontal microbiology, with special emphasis on advanced molecular techniques: A review. J Clin Periodontol. 2004;31:1034–1047.
- Petti CA, Polage CR, Schreckenberger P. The role of 16S rRNA gene sequencing in identification of microorganisms misidentified by conventional methods. J Clin Microbiol. 2005;43:6123–6125.
- Padilla C, Lobos O, Hubert E, et al. Periodontal pathogens in atheromatous plaques isolated from patients with chronic periodontitis. J Periodontal Res. 2006;41:350–353.
- Toyofuku T, Inoue Y, Kurihara N, et al. Differential detection rate of periodontopathic bacteria in atherosclerosis. Surg Today. 2011;41:1395–1400.
- Figuero E, Lindahl C, Marín MJ, et al. Quantification of Periodontal Pathogens in Vascular, Blood and Subgingival Samples from Patients with Peripheral Arterial Disease or Abdominal Aortic Aneurysms. J Periodontol. 2014;85:1–19.
- Haraszthy VI, Zambon JJ, Trevisan M, et al. Identification of periodontal pathogens in atheromatous plaques. J Periodontol. 2000;71:1554–1560.
- Cairo F, Gaeta C, Dorigo W, et al. Periodontal pathogens in atheromatous plaques. A controlled clinical and laboratory trial. J Periodontal Res. 2004;39:442–446.
- Fiehn NE, Larsen T, Christiansen N, et al. Identification of periodontal pathogens in atherosclerotic vessels. J Periodontal Res. 2005;76:731–736.
- Aimetti M, Romano F, Nessi F. Microbiologic analysis of periodontal pockets and carotid atheromatous plaques in advanced chronic periodontitis patients. J Periodontol. 2007;78:1718–1723.
- Figuero E, Sánchez-Beltrán M, Cuesta-Frechoso S, et al. Detection of Periodontal Bacteria in Atheromatous Plaque by Nested Polymerase Chain Reaction. J Periodontol. 2011;82:1469–1477.
- Fernandes CP, Oliveira FAF, Silva PGDB, et al. Molecular analysis of oral bacteria in dental biofilm and atherosclerotic plaques of patients with vascular disease. Int J Cardiol. 2014;174:710–712.
- Kozarov E, Sweier D, Shelburne C, et al. Detection of bacterial DNA in atheromatous plaques by quantitative PCR. Microbes Infect. 2006;8:687–693.
- Aquino ARL, Lima KC, Paiva MS, et al.. Molecular survey of atheromatous plaques for the presence of DNA from periodontal bacterial pathogens, archaea and fungi. J Periodontal Res. Blackwell Publishing Ltd; 2011;46:303–309.
- Ishihara K, Nabuchi A, Ito R, et al. Correlation between Detection Rates of Periodontopathic Bacterial DNA in Carotid Coronary Stenotic Artery Plaque and in Dental Plaque Samples. J Clin Microbiol. American Society for Microbiology; 2004;42:1313–1315.
- Pucar A, Milasin J, Lekovic V, et al. Correlation between atherosclerosis and periodontal putative pathogenic bacterial infections in coronary and internal mammary arteries. J Periodontol. 2007;78:677–682.
- Gaetti-Jardim E, Marcelino SL, Feitosa ACR, et al. Quantitative detection of periodontopathic bacteria in atherosclerotic plaques from coronary arteries. J Med Microbiol. Microbiology Society; 2009;58:1568–1575.
- Mahendra J, Mahendra L, Kurian VM, et al. Prevalence of periodontal pathogens in coronary atherosclerotic plaque of patients undergoing coronary artery bypass graft surgery. J Maxillofac Oral Surg. 2009;8:108–113.
- Rath S, Mukherjee M, Kaushik R, et al. Periodontal pathogens in atheromatous plaque. Indian J Pathol Microbiol. Medknow Publications and Media Pvt. Ltd.; 2014;57:259.
- Mahalakshmi K, Krishnan P, Krishna Baba MG, et al. “Association of periodontopathic anaerobic bacterial co-occurrence to atherosclerosis” – A cross-sectional study. Anaerobe. Elsevier Ltd; 2017;44:66–72.
- Atarbashi-Moghadam F, Havaei SR, Havaei SA, et al. Periopathogens in atherosclerotic plaques of patients with both cardiovascular disease and chronic periodontitis. ARYA Atheroscler. 2018;14:53–57.
- Kurihara N, Inoue Y, Iwai T, et al. Detection and localization of periodontopathic bacteria in abdominal aortic aneurysms. Eur J Vasc Endovasc Surg. 2004;28:553–558.
- Marques da Silva R, Caugant DA, Lingaas PS, et al. Detection of Actinobacillus actinomycetemcomitans But Not Bacteria of the Red Complex in Aortic Aneurysms by Multiplex Polymerase Chain Reaction. J Periodontol. 2005;76:590–594.
- Nakano K, Nemoto H, Nomura R, et al. Detection of oral bacteria in cardiovascular specimens. Oral Microbiol Immunol. 2009;24:64–68.
- Delbosc S, Alsac J-M, Journe C, et al. Porphyromonas gingivalis participates in pathogenesis of human abdominal aortic aneurysm by neutrophil activation. Proof of concept in rats. PLoS One. 2011;6.
- Pyysalo MJ, Pyysalo LM, Pessi T, et al. The connection between ruptured cerebral aneurysms and odontogenic bacteria. J Neurol Neurosurg Psychiatry. 2013;84:1214–1218.
- Elkaïm R, Dahan M, Kocgozlu L, et al. Prevalence of periodontal pathogens in subgingival lesions, atherosclerotic plaques and healthy blood vessels: A preliminary study. J Periodontal Res. 2008;43:224–231.
- Okuda K, Ishihara K, Nakagawa T, et al. Detection of Treponema denticola in Atherosclerotic Lesions. 2001;39:1114–1117.
- Taylor-Robinson D, Aduse-Opoku J, Sayed P, et al. Oro-dental bacteria in various atherosclerotic arteries. Eur J Clin Microbiol Infect Dis. 2002;21:755–757.
- Koren O, Spor A, Felin J, et al. Human oral, gut, and plaque microbiota in patients with atherosclerosis. Proc Natl Acad Sci U S A. 2011;108(Suppl):4592–4598. DOI:10.1073/pnas.1011383107
- Armingohar Z, Jørgensen JJ, Kristoffersen AK, et al. Bacteria and bacterial DNA in atherosclerotic plaque and aneurysmal wall biopsies from patients with and without periodontitis. J Oral Microbiol. 2014;6:23408.
- Gemmell MR, Berry S, Mukhopadhya I, et al. Comparative genomics of Campylobacter concisus: Analysis of clinical strains reveals genome diversity and pathogenic potential. Emerg Microbes Infect. 2018;7:116.
- Kelsen JR, Wu GD. The gut microbiota, environment and diseases of modern society. Gut Microbes. 2012;3:374–382.
- Galan M, Razzauti M, Bard E, et al. 16S rRNA amplicon sequencing for epidemiological surveys of bacteria in wildlife : the importance of cleaning post-sequencing data before estimating positivity, prevalence and co-infection. mSystems. 2016;1:e00032–16.
- Fujimoto S, Nakagami Y, Kojima F. Optimal Bacterial DNA Isolation Method Using Bead-Beating Technique. Med Sch. 2004;3:33–38.
- Coscas R, Bensussan M, Jacob M-P, et al. Free DNA precipitates calcium phosphate apatite crystals in the arterial wall in vivo. Atherosclerosis. 2017;259:60–67.
- Feehery GR, Yigit E, Oyola SO, et al. A method for selectively enriching microbial DNA from contaminating vertebrate host DNA. Highlander SK, ed. PLoS One. 2013;8:e76096.
- Marin MJ, Figuero E, Herrera D, et al. Quantitative Analysis of Periodontal Pathogens Using Real-Time Polymerase Chain Reaction (PCR). Methods Mol Biol. 2017;191–202.
- Kennedy NA, Walker AW, Berry SH, et al. The impact of different DNA extraction kits and laboratories upon the assessment of human gut microbiota composition by 16S rRNA gene sequencing. PLoS One. Public Library of Science; 2014;9:e88982. DOI: 10.1371/journal.pone.0088982
- Cole JR, Wang Q, Fish JA, et al. Ribosomal Database Project: data and tools for high throughput rRNA analysis. Nucleic Acids Res. 2014;42:D633–D642.
- Lee PY, Costumbrado J, Hsu C-Y, et al. Agarose Gel Electrophoresis for the Separation of DNA Fragments. J Vis Exp. 2012 ;3923.
- Hajishengallis G. Periodontitis: from microbial immune subversion to systemic inflammation. Nat Rev Immunol. Nature Publishing Group; 2014;15:30–44.