ABSTRACT
Objective Treponema denticola is involved in ‘chronic’ periodontitis pathogenesis. The mechanism underlying the regulation of the expression of its virulence factors, such as major surface protein (Msp) and prolyl-phenylalanine specific protease (dentilisin) is yet to be clarified. We determined the gene expression profiles of Msp- and dentilisin-deficient mutants of T. denticola to identify the regulation network of gene expression concomitant with the inactivation of these virulence genes.
Methods Gene expression profiles of T. denticola ATCC 35405 (wild type), dentilisin-deficient mutant K1, and msp-deficient mutant DMSP3 were determined using DNA microarray analysis and quantitative real-time reverse transcription PCR (qRT-PCR). Msp and dentilisin protein levels were determined by immunoblotting and proteolytic activity assays.
Results In addition to several differentially expressed genes, dentilisin expression was reduced in DMSP3; msp expression was significantly reduced in K1 (p < 0.05), both at the gene and protein levels. To identify the regulatory system involved, the expression levels of the potential regulators whose expression showed changes in the mutants were evaluated using qRT-PCR. Transcriptional regulators TDE_0127 and TDE_0814 were upregulated in K1, and the potential repressor, TDE_0344, was elevated in DMSP3.
Conclusions Dentilisin and Msp expression were interrelated, and gene expression regulators, such as TDE_0127, may be involved in their regulation.
Introduction
Chronic periodontitis is an infectious disease that leads to the destruction of the periodontal tissues and represents a major cause of tooth loss in adults [Citation1]. Dysbiosis in the subgingival microbiome is a major cause of periodontitis [Citation2]. Along with Porphyromonas gingivalis and Tannerella forsythia, the prevalence of Treponema denticola reportedly increases in the subgingival microbiome during the dysbiotic shift associated with the development of chronic periodontitis [Citation3,Citation4]. This shift is believed to play an important role in the onset and progression of the disease [Citation5]. Further, T. denticola was also detected in lesions of apical periodontitis [Citation6]. These reports suggest that T. denticola is involved in the dysbiotic shift of the microbiome at the site of inflammation. In addition, T. denticola was also detected in systemic diseases such as atherosclerotic lesions [Citation7].
Major surface protein (Msp) and prolyl-phenylalanine-specific protease (dentilisin) are the major virulence factors of T. denticola [Citation8]. Dentilisin and Msp are located in the outer sheath of T. denticola [Citation9]. Msp is a highly immunogenic 53 kDa protein [Citation10] and organizes a heat modifiable high molecular-weight complex [Citation11]. The structure of Msp, predicted from its amino acid sequence, was reported to be similar to those of other bacterial porins, in which several membrane-spanning amphipathic β sheets are arranged in a pore-forming β barrel [Citation12]. Msp has been reported to possess cytopathic activity owing to its pore-forming activity against epithelial cells [Citation9]. It also mediates the extracellular matrix binding activity of T. denticola via adherence to fibronectin and laminin [Citation13].
Dentilisin is a prolyl-phenylalanine-specific protease [Citation14]. It consists of four proteins coded by three genes (prcB, prcA, and prtP) [Citation14,Citation15]. Among them, prtP codes for a 72 kDa protease domain, which degrades components of the extracellular matrix such as fibronectin, activates C3, and is involved in adherence to fibrinogen [Citation14,Citation16,Citation17]. Additionally, dentilisin is also involved in adherence to fibronectin, and immunomodulation [Citation8]. Further, the coaggregation activity between T. denticola and T. forsythia was reported to be reduced in a dentilisin-dependent manner in the dentilisin-deficient mutant, K1 [Citation18]. Additionally, K1 showed attenuated invasiveness to gingival epithelial cells compared to the wild type strain [Citation19]. Moreover, the gingival epithelial cells infected by T. denticola wild type strain ATCC 35405 showed attenuated migration activity, while a lower attenuation was observed with K1 and the Msp-deficient mutant, DMSP3.
Msp and dentilisin have been co-isolated from the outer sheath of T. denticola [Citation9], and an oligomeric form of Msp, which was observed in the wild type strain, was dissociated in strain K1 [Citation20]. Furthermore, msp expression is affected by the inactivation of dentilisin [Citation21]; however, the mechanism underlying this phenomenon is yet to be clarified. These reports were indicative of interactions between the pathways regulating the expression of dentilisin and Msp, although the mechanisms regulating the expression of each individual gene remain unknown. In this study, we investigated the gene expression profile of Msp-deficient and dentilisin-deficient T. denticola mutants to identify the regulation system involved with the inactivation of these virulence genes, focusing on the regulator proteins.
Materials and methods
Strains and culture conditions
T. denticola ATCC 35405 [Citation22], msp-deficient mutant, T. denticola DMSP3 [Citation18], and a prtP-deficient mutant, T. denticola K1 [Citation20], were maintained in TYGVS medium [Citation23] at 37°C under anaerobic conditions (N2: 80%, H2: 10%, CO2: 10%).
DNA microarray analysis
RNA was extracted from T. denticola ATCC 35405, K1, and DMSP3, as described previously [Citation24]. Briefly, mid-log phase-grown T. denticola cells were harvested via centrifugation, and total RNA was extracted using the Trizol reagent (Invitrogen, Carlsbad, CA) according to the manufacturer’s instructions. The resultant samples were treated with Turbo DNase I (Life Technologies Japan Ltd, Tokyo, Japan) twice at 37°C for 30 min to remove genomic DNA contamination. The quality of the RNA was evaluated by TapeStation (Agilent Technologies, Santa Clara, CA).
Microarray analysis was performed using the T. denticola 8 × 15 K 1 color system (Hokkaido System Science, Sapporo, Japan). Briefly, the labeled cRNA was synthesized using cDNA as the template with cyanine 3′ CTP using the Low Input Quick-Amp WT labeling kit (Agilent Technologies). The labeled cRNA was hybridized to microarray slides. Microarray image acquisition was performed using the Agilent Microarray Scanner and Agilent Feature Extraction 10. 7. 3. 1. (Agilent Technologies). Differentially expressed genes were determined using a fold-change of at least 2.0, with a significance level of p < 0.05. The microarray data have been deposited in NCBI’s Gene Expression Omnibus [Citation25].
Real-time reverse transcription-PCR (qRT-PCR) analysis
Total RNA was isolated as described above. cDNA was synthesized using ReverTra Ace (Toyobo, Osaka, Japan). To remove RNA contamination, the cDNA samples were treated with RNaseH (New England Biolabs, Tokyo, Japan) at 37°C for 20 min and then at 65 °C for 20 min. The quantity of the DNA was measured using a Quantus fluorometer (Promega, Tokyo, Japan). For qRT-PCR analysis, the cDNA samples were diluted in RNase-free water. For each well, 1 µl of the cDNA samples was added to a solution containing 10 µl TaqMan Fast Universal PCR master mix (Life Technologies Japan Ltd., Tokyo, Japan), 1 µl gene-specific primers mix (), and 8 µl RNase-free water. The qRT-PCR analysis was performed in triplicates with the StepOnePlus real-time PCR system (Life Technologies Japan Ltd.). The expression of each gene was normalized to that of the 16S rRNA, an internal control.
Table 1. Primers for PCR and qRT-PCR.
Measurement of dentilisin activity
T. denticola ATCC 35405 and DMSP3 grown in TYGVS medium were collected at mid-log (OD660 of 0.5) and stationary phase (OD660 of 1.0). The aliquots (0.5 ml) were separated into the bacterial pellet and supernatant via centrifugation at 3,000 × g for 10 min. The cells were then washed with phosphate-buffered saline (PBS, pH 7.2) and were resuspended in double the volume (1 ml) of 50 mM Tris-HCl buffer (pH 8.0). The supernatant was filtered to remove the remaining cells and was mixed with the same volume of 100 mM Tris-HCl buffer (pH 8.0). The samples (120 µl) were mixed with 30 µl 5 mM synthetic chromogenic substrate for chymotrypsin [N-Succinyl-Ala-Ala-Pro-Phe p-nitroanilide (SAAPFNA)] (Sigma, St. Louis, MO) and incubated for 30 min at 37°C. The reaction was then stopped by the addition of 50 µl 20% acetic acid. The release of p-nitroanilide was determined by measuring optical density (OD) at 405 nm using a microplate reader (SpecroraMax M5e, Molecular device, Sunnyvale, CA). The OD of the blank sample was subtracted from the OD of each sample.
Protein profile of the mutant
Protein profiles of the T. denticola wild type strain, K1, and DMSP3 were compared using sodium dodecyl sulfate-polyacrylamide gel electrophoresis (SDS-PAGE) and immunoblotting. T. denticola strains were washed with PBS twice and disrupted with a sonicator (Branson, Danbury, CT) at 100 W for 5 min on ice. Insoluble material was removed by centrifugation at 6,000 g for 30 min. Ten µg of sonicates were loaded on 10% SDS-polyacrylamide gel and electrophoresed at 30 mA for 60 min. After electrophoresis, the gel was stained with 2D-silver stain reagent II (Cosmobio, Tokyo, Japan). For immunoblotting, 10 µg of sonicates were electrophorased on the gel, as described above. The electrophoresed protein was transferred to apolyvinylidene fluoride (PVDF) membrane (Immobiline, Merck Millipore, Darmstadt, Germany). The membrane was blocked with PBS containing 3% bovine serum albumin at room temperature for 60 min. After washing with PBS containing Tween 20 (PBS-T), the membrane was incubated with 1/20,000 diluted rabbit anti-T.denticola Msp serum [Citation20] at room temperature for 60 min and 1/100,000 diluted horseradish peroxidase-conjugated goat anti-rabbit IgG thereafter. The membrane was washed with PBS-T, developed with enhanced chemiluminescence (ECL) western blotting detection reagents (GE Healthcare UK Ltd., Little Chalfont, England), and photographed using LAS-4000 (GE Healthcare UK Ltd.).
Statistical analyses
All experiments were repeated at least twice. The results were expressed as mean values with standard deviations. Student’s t-test and one-way analysis of variance (ANOVA) with Dunnett’s multiple comparison test were used to determine the differences between groups using GraphPad Prism 7.0d (GraphPad Software, La Jolla, CA). The level of significance for all statistical tests was set at p < 0.05.
Results
DNA microarray analysis
To investigate the effect of inactivation of prtP and msp on the gene expression profile of T. denticola, DNA microarray analysis of wild type, K1, and DMSP3 strains were performed. In K1, the expression levels of ParB-like nuclease, DNA-binding protein (TDE_0127), several ABC transporters, peptidase, silent mating-type information regulation (Sir) 2 family of transcriptional regulators, and membrane proteins such as OmpA and lipoprotein were increased (). On the other hand, the expression of several ABC transporters, MATE family transporters, acetyltransferases, lipoproteins, and Msp was reduced in K1 (). In DMSP3, the number of genes with increased expression was relatively lower than that in K1. The expression levels of alpha/beta fold family hydrolase, AbrB family transcriptional regulator (TDE_0344), purine nucleoside phosphorylase, and lipoprotein were increased in DMSP3 (). The expression of the surface antigen BspA was significantly reduced in DMSP3. In addition, genes encoding methyltransferase, methylthioribose kinase, several transporters, tetratricopeptide repeats (TPR), and methylthioribose kinase were downregulated (). The expression of msp in strain K1 was significantly lower than that in the wild type strain (). In addition, the expression of prtP in strain DMSP3 was 0.68-fold lower than that in the wild type strain, although it was not statistically significant ().
Table 2. Upregulated genes in prtP-deficient mutant.
Table 3. Genes downregulated in prtP-deficient mutant.
Table 4. Genes upregulated in msp-deficient mutant.
Table 5. Genes downregulated in msp-deficient mutant.
Expression analysis of msp and prtP in the mutants
qRT-PCR was performed to confirm the above observations. ) shows the expression of msp in the wild type and mutant strains. In agreement with the −2.35-fold reduction in msp expression observed in the microarray assay, msp expression was significantly reduced to 8.7% of that in the wild type strain in the dentilisin mutant. Although the expression of prtP was somewhat lower in the microarray assay, it was not statistically significant. However, the qRT-PCR assay showed a significant, albeit slight reduction in prtP expression in the msp mutant (39.3%, )).
Figure 1. Expression of msp and prtP determined using qRT-PCR. (a) Expression of msp. (b) Expression of prtP (dentilisin); 35405: T. denticola ATCC 35405 (wild type), K1: dentilisin mutant, DMSP3: msp mutant. The expression of each gene is presented as fold-change over that in the wild type strain. Data are presented as means ± SD (n = 6), and statistically significant differences are indicated using asterisks (one-way ANOVA with Dunnett’s multiple comparison test; *p < 0.05 compared to the wild type).
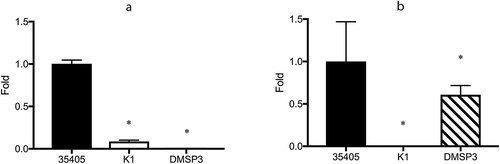
The reduction in expression was also confirmed at the protein level. The results of the SDS-PAGE analysis of DMSP3 and K1 are shown in ). In strain DMSP3, a 53-kDa band disappeared, while in K1, 100 kDa and 130 kDa bands, which were not detected in the wild type, were observed, along with a smeared band at around 60 kDa. These bands in K1 were detected in the PMSF-treated wild type strain (data not shown), suggesting that these bands were degraded by dentilisin in the process of sample preparation for SDS-PAGE. The results of immunoblotting using an anti-Msp antibody revealed that the density of the Msp band was significantly lower in K1 compared to that in the wild type ()). These results indicated that the inactivation of prtP affects the expression of msp.
Figure 2. SDS-PAGE and immunoblot analysis of sonicates of T. denticola ATCC 35405, DMSP3, and K1. SDS-PAGE analysis (a) and immunoblot analysis (b).
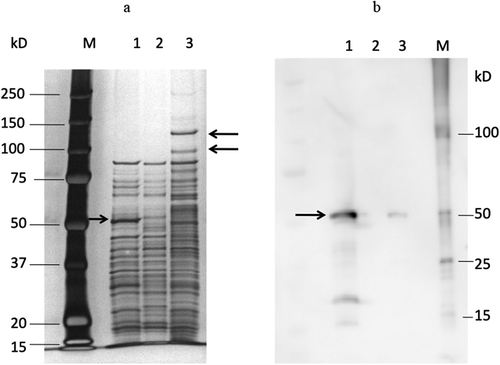
Although the expression of dentilisin in the msp mutant showed a statistically significant reduction, it was not biologically significant. The dentilisin activity in the msp mutant was assayed to clarify its expression. The dentilisin activity assay indicated that the cell-associated dentilisin activity of the msp mutant was almost similar in the wild type and mutant strains; however, the activity in the culture supernatant of the mutant was significantly lower than that of the wild type (). These results indicated that the expression of the virulence factors was interrelated, as the expression of one was affected by the inactivation of the other.
Figure 3. Dentilisin activity of wild type (35405) and msp-deficient mutant (DMSP3). Data are presented as means ± SD, and statistically significant differences are indicated by asterisks (student t-test; *p < 0.05 compared to the wild type). 35405: T. denticola ATCC 35405, DMSP3: T. denticola DMSP3, cell: cells of T. denticola, sup: culture supernatant of T. denticola, log: log phase, stationary: stationary phase.
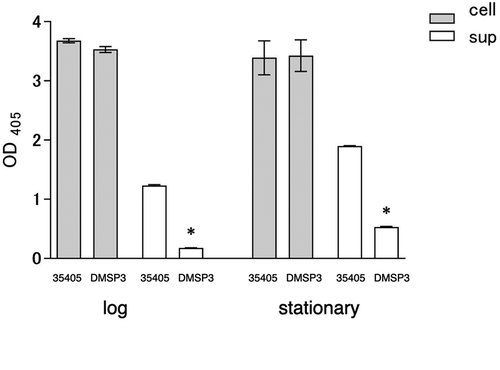
Expression of potential transcriptional regulators in the mutants
The results of microarray and qRT-PCR analyses showed that the inactivation of msp affected the expression of prtP and vice versa. To clarify this further, the expression of several potential transcriptional regulators, whose expression levels were high in the microarray analysis, were investigated using qRT-PCR. In K1, the expression levels of DNA-binding protein (TDE_0127) and transcriptional regulator (TDE_0814) were significantly higher than those in the wild type strain ( and )). In the genome sequence of T. denticola ATCC 35405, two open reading frames (TDE_0037 and TDE_0344) are annotated as the AbrB family of transcriptional regulators. In DMSP3, the expression of the AbrB family of transcriptional regulators (TDE_0344) was 3.2 times higher than that in the wild type strain, while that of the DNA-binding protein (TDE_0127) was reduced to 28.4% of that in the wild type strain ( and )).
Figure 4. Gene expression of the potential regulator proteins in wild type (35405), msp-deficient mutant (DMSP3), and prtP-deficient mutant (K1). Gene expression levels were evaluated using qRT-PCR with a Taqman probe. The expression of TDE_0127 (a), TDE_0344 (b), and TDE_0814 (c) are presented as fold-change with respect to that of the wild type strain. Data are presented as means ± SD (n = 6), and statistically significant differences are indicated by asterisks (one-way ANOVA with Dunnett’s multiple comparison test; *p < 0.05 compared to wild type).
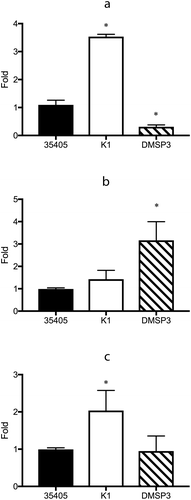
Discussion
In the present study, the inactivation of prtP affected the expression of msp and vice versa. The reduction of msp expression in the dentilisin mutant was in agreement with the results of Bian et al. [Citation21], who reported that compared to that in the wild type, the level of total Msp was considerably reduced and that the Msp oligomers were barely detected in the prtP-deficient mutant. In the present study, the results of qRT-PCR and immunoblotting indicated that the msp expression at both mRNA and protein levels, respectively, was reduced in the prtP-deficient mutant. Interestingly, mRNA expression of prtP was also reduced in the msp mutant. Cell-associated dentilisin activity in T. denticola wild type and the msp mutant was almost similar, as determined by the activity assay; however, the activity of the msp mutant in the culture supernatant was significantly lower than that of the wild type supernatant. Vesicle release has been reported in T. denticola, and Msp and dentilisin are located in the vesicle [Citation26]. A recent report indicated that PrtP, PrcA1, and PrcA2 from PacA, and PrcB were enriched in the outer membrane vesicle [Citation27]. The inactivation of Msp or the reduction of prtP expression may affect the formation of vesicles containing dentilisin. These results suggested the existence of a regulatory system, which acts in response to the inactivation of prtP or msp to regulate their expression.
Dentilisin and msp mutations altered the mRNA expression profiles of a number of genes with various functions, suggesting the involvement of a gene repressor or activator in regulating gene expression in the mutants. Sigma factors, the two-component system, and the one-component system control bacterial gene expression [Citation28–Citation30]. The two-component system has been studied in the adaptation of T. denticola [Citation31]. Regulation by the AtcR LytTR domain-containing response regulator has been reported, and these proteins regulated gene expression in response to environmental conditions such as oxygen levels [Citation32,Citation33]. The regulation by the sigma factors has not been clarified yet. In the one-component system, TroR, which regulates the troABCDR operon as a repressor under iron- and manganese-limited conditions, is the only regulatory protein [Citation34,Citation35]. The regulator proteins identified in the previous studies are not reported to regulate the expression of Msp or dentilisin. In the present study, changes in the expression of potential sigma factors and response regulators were not observed. In contrast, the expression level of the DNA-binding protein (TDE_0127) in K1 was 3.54 times higher than that in the wild type. The predicted structure of this protein is similar to that of helix-turn-helix DNA-binding proteins (cd00093, NCBI), which include the HipB protein of Escherichia coli, a protein belonging to the xenobiotic response element family of transcriptional regulators. Interestingly, the TDE_0127 mRNA level was reduced in DMSP3. It indicated that TDE_0127 regulates gene expression in response to both msp and prtP inactivation. TDE_0127 level has been reported to increase under oxidative stress [Citation36]. TDE_0127 may be involved in gene regulation under multiple stress conditions.
In strain DMSP3, a large number of genes were downregulated compared to that in the wild type, while a few genes were upregulated in the strain. The expression of the surface antigen BspA showed a − 20.49-fold reduction. This protein is reported to be involved in coaggregation with T. forsythia and adhesion to HEp-2 cells [Citation37]. As Msp is the major surface protein, a regulation system concerning the surface molecule may be involved in this change. Among the genes with increased expression in the msp mutant, the AbrB family transcriptional regulator (TDE_0344) showed a 3.16-fold higher expression than that in the wild type strain. On the other hand, the expression of another AbrB family transcriptional regulator, TDE_0037, was not changed in the msp mutant. This gene was co-transcribed with ActR-ActS, a member of the two-component system, and the expression of the operon increased during late-stage growth [Citation38]; however, the function was not clear. The function of the proteins coded by TDE_0037 and TDE_0344 might be different, although both have similarities with the AbrB family transcriptional regulator. In Bacillus subtilis, AbrB is involved in the control of over 40 different genes nominally expressed or repressed in suboptimal environmental conditions characterized by limited nutrient resources [Citation39,Citation40]. AbrB acts as a repressor and activator in the regulation of post-exponential gene expression in B. subtilis and regulates subtilisin expression in B. subtilis [Citation40]. In the present study, TDE_0344 was increased in the msp-deficient mutant. Dentilisin and Msp organize a complex in T. denticola [Citation11], and Msp and PrcA2 interact with the outer sheath [Citation41]. TDE_0344 might be activated by a stimulus sensed by the outer membrane proteins. The amino acid sequence of dentilisin is similar to that of subtilisin [Citation14]. It is possible that the AbrB family transcriptional regulator (TDE_0344) controls the recovery of the inactivated or downregulated genes, including prtP, although further analysis is required to verify this hypothesis.
The transcriptional regulator, TDE_0814, showed similarity to HxlR-like helix-turn-helix (pfam01638, DDBJ), which is a DNA-binding protein that acts as a positive regulator of the formaldehyde-inducible hxlAB operon in B. subtilis [Citation42]. In the present study, compared to that in the wild type, the expression of several genes increased in strain K1. Possibly, this increased expression reflected the increase in the expression of TDE_0814. The expression of the Sir2 family of transcriptional regulators was increased in K1. Sir proteins are conserved across eukaryotes and are involved in deacetylating several substrates. Sirtuins are an evolutionarily conserved family of NAD (+)-dependent protein deacetylases that function in the regulation of gene transcription, cellular metabolism, and aging [Citation43]. However, their role in the regulation of prokaryotic gene expression is unclear. The Sir2 transcriptional regulator is unlikely to regulate the expression of T. denticola genes, including msp although further analysis is required.
Taken together, the inactivation of prtP or msp induced changes in the expression of several genes, including prtP and msp, indicating that the expression of these virulence factors was interrelated. Several potential DNA-binding proteins or transcriptional regulators, such as TDE_0127, are suggested to be involved in the regulation system, which acts concomitantly with the inactivation of Msp and dentilisin. However, further analysis is required to clarify the DNA-binding activity and the binding site on the DNA.
Disclosure statement
The authors reported no potential conflict of interest.
Correction Statement
This article has been republished with minor changes. These changes do not impact the academic content of the article.
Additional information
Funding
References
- Kinane DF, Stathopoulou PG, Papapanou PN. Periodontal diseases. Nat Rev Dis Primers. 2017;3:17038.
- Lamont RJ, Koo H, Hajishengallis G. The oral microbiota: dynamic communities and host interactions. Nat Rev Microbiol. 2018;16(12):745–9.
- Griffen AL, Beall CJ, Campbell JH, et al. Distinct and complex bacterial profiles in human periodontitis and health revealed by 16S pyrosequencing. Isme J. 2012;6(6):1176–1185.
- Socransky SS, Haffajee AD, Cugini MA, et al. Microbial complexes in subgingival plaque. J Clin Periodontol. 1998;25(2):134–144.
- Ellen RP, Galimanas VB. Spirochetes at the forefront of periodontal infections. Periodontol. 2005;38:13–32.
- Cogulu D, Oncag O, Kutukculer N, et al. The correlation between serum immunoglobulin A and immunoglobulin G levels and the presence of Treponema denticola in human periapical lesions. J Endod. 2007;33(12):1413–1416.
- Ishihara K, Nabuchi A, Ito R, et al. Correlation between detection rates of periodontopathic bacterial DNA in carotid coronary stenotic artery plaque and in dental plaque samples. J Clin Microbiol. 2004;42(3):1313–1315.
- Ishihara K. Virulence factors of Treponema denticola. Periodontol. 2010;54(1):117–135.
- Fenno JC, Hannam PM, Leung WK, et al. Cytopathic effects of the major surface protein and the chymotrypsinlike protease of Treponema denticola. Infect Immun. 1998;66(5):1869–1877.
- Umemoto T, Namikawa I, Suido H, et al. A major antigen on the outer envelope of a human oral spirochete, Treponema denticola. Infect Immun. 1989;57(8):2470–2474.
- Haapasalo M, Muller KH, Uitto VJ, et al. Characterization, cloning, and binding properties of the major 53-kilodalton Treponema denticola surface antigen. Infect Immun. 1992;60(5):2058–2065.
- Godovikova V, Goetting-Minesky MP, Timm JC, et al. Immunotopological analysis of the Treponema denticola major surface protein (Msp). J Bacteriol. 2019;201(2):e00528–18.
- Fenno JC, Muller KH, McBride BC. Sequence analysis, expression, and binding activity of recombinant major outer sheath protein (Msp) of Treponema denticola. J Bacteriol. 1996;178(9):2489–2497.
- Ishihara K, Miura T, Kuramitsu HK, et al. Characterization of the Treponema denticola prtP gene encoding a prolyl-phenylalanine-specific protease (dentilisin). Infect Immun. 1996;64(12):5178–5186.
- Godovikova V, Wang HT, Goetting-Minesky MP, et al. Treponema denticola PrcB is required for expression and activity of the PrcA-PrtP (dentilisin) complex. J Bacteriol. 2010;192(13):3337–3344.
- Bamford CV, Fenno JC, Jenkinson HF, et al. The chymotrypsin-like protease complex of Treponema denticola ATCC 35405 mediates fibrinogen adherence and degradation. Infect Immun. 2007;75(9):4364–4372.
- Yamazaki T, Miyamoto M, Yamada S, et al. Surface protease of Treponema denticola hydrolyzes C3 and influences function of polymorphonuclear leukocytes. Microbes Infect. 2006;8(7):1758–1763.
- Sano Y, Okamoto-Shibayama K, Tanaka K, et al. Dentilisin involvement in coaggregation between Treponema denticola and Tannerella forsythia. Anaerobe. 2014;30:45–50.
- Inagaki S, Kimizuka R, Kokubu E, et al. Treponema denticola invasion into human gingival epithelial cells. Microb Pathog. 2016;94:104–111.
- Ishihara K, Kuramitsu HK, Miura T, et al. Dentilisin activity affects the organization of the outer sheath of Treponema denticola. J Bacteriol. 1998;180(15):3837–3844.
- Bian XL, Wang HT, Ning Y, et al. Mutagenesis of a novel gene in the prcA-prtP protease locus affects expression of Treponema denticola membrane complexes. Infect Immun. 2005;73(2):1252–1255.
- Chan EC, Siboo R, Keng T, et al. Treponema denticola (ex Brumpt 1925) sp. nov., nom. rev., and identification of new spirochete isolates from periodontal pockets. Int J Syst Bacteriol. 1993;43(2):196–203.
- Ohta K, Makinen KK, Loesche WJ. Purification and characterization of an enzyme produced by Treponema denticola capable of hydrolyzing synthetic trypsin substrates. Infect Immun. 1986;53(1):213–220.
- Tanaka-Kumazawa K, Kikuchi Y, Sano-Kokubun Y, et al. Characterization of a potential ABC-type bacteriocin exporter protein from Treponema denticola. BMC Oral Health. 2016;17(1):18.
- Edgar R, Domrachev M, Lash AE. Gene Expression Omnibus: NCBI gene expression and hybridization array data repository. Nucleic Acids Res. 2002;30(1):207–210.
- Rosen G, Naor R, Rahamim E, et al. Proteases of Treponema denticola outer sheath and extracellular vesicles. Infect Immun. 1995;63(10):3973–3979.
- Veith PD, Glew MD, Gorasia DG, et al. Localization of outer membrane proteins in Treponema denticola by quantitative proteome analyses of outer membrane vesicles and cellular fractions. J Proteome Res. 2019;18(4):1567–1581.
- Browning DF, Busby SJ. Local and global regulation of transcription initiation in bacteria. Nat Rev Microbiol. 2016;14(10):638–650.
- Cuthbertson L, Nodwell JR. The TetR family of regulators. Microbiol Mol Biol Rev. 2013;77(3):440–475.
- Galperin MY. Structural classification of bacterial response regulators: diversity of output domains and domain combinations. J Bacteriol. 2006;188(12):4169–4182.
- Frederick JR, Sarkar J, McDowell JV, et al. Molecular signaling mechanisms of the periopathogen, Treponema denticola. J Dent Res. 2011;90(10):1155–1163.
- Miller DP, Frederick JR, Sarkar J, et al. The Treponema denticola AtcR LytTR domain-containing response regulator interacts with three architecturally distinct promoter elements: implications for understanding the molecular signaling mechanisms that drive the progression of periodontal disease. Mol Oral Microbiol. 2014;29(5):219–232.
- Sarkar J, Miller DP, Oliver LD Jr., et al. The Treponema denticola PAS domain-containing histidine kinase Hpk2 is a heme binding sensor of oxygen levels. J Bacteriol. 2018;200(18):e00116–00118.
- Brett PJ, Burtnick MN, Fenno JC, et al. Treponema denticola TroR is a manganese- and iron-dependent transcriptional repressor. Mol Microbiol. 2008;70(2):396–409.
- Saraithong P, Goetting-Minesky MP, Durbin PM, et al. Roles of TroA and TroR in metalloregulated growth and gene expression in Treponema denticola. J Bacteriol. 2020;202(7):e00770–00719.
- McHardy I, Keegan C, Sim JH, et al. Transcriptional profiles of Treponema denticola in response to environmental conditions. PLoS One. 2010;5(10):e13655.
- Ikegami A, Honma K, Sharma A, et al. Multiple functions of the leucine-rich repeat protein LrrA of Treponema denticola. Infect Immun. 2004;72(8):4619–4627.
- Frederick JR, Rogers EA, Marconi RT. Analysis of a growth-phase-regulated two-component regulatory system in the periodontal pathogen Treponema denticola. J Bacteriol. 2008;190(18):6162–6169.
- Strauch MA. Regulation of Bacillus subtilis gene expression during the transition from exponential growth to stationary phase. Prog Nucleic Acid Res Mol Biol. 1993;46:121–153.
- Strauch MA, Hoch JA. Transition-state regulators: sentinels of Bacillus subtilis post-exponential gene expression. Mol Microbiol. 1993;7(3):337–342.
- Godovikova V, Goetting-Minesky MP, Fenno JC. Composition and localization of Treponema denticola outer membrane complexes. Infect Immun. 2011;79(12):4868–4875.
- Yurimoto H, Hirai R, Matsuno N, et al. HxlR, a member of the DUF24 protein family, is a DNA-binding protein that acts as a positive regulator of the formaldehyde-inducible hxlAB operon in Bacillus subtilis. Mol Microbiol. 2005;57(2):511–519.
- McClure JM, Wierman MB, Maqani N, et al. Isonicotinamide enhances Sir2 protein-mediated silencing and longevity in yeast by raising intracellular NAD+ concentration. J Biol Chem. 2012;287(25):20957–20966.