ABSTRACT
Background: Intake of probiotic bacteria may prevent oral Candida infection.
Objective: To screen the antifungal activity of 14 Lactobacillus candidate strains of human origin, against six opportunistic C. albicans and non-albicans species. A second aim was to study the acid production of the four strains showing the strongest antifungal activity.
Methods: We used an agar overlay growth inhibition assay to the assess the antifungal activity of the lactobacilli. The acid-producing capacity was measured with pH micro-sensors.
Results: All 14 Lactobacillus candidates inhibited the growth of the Candida spp. The four best-performing strains were L. rhamnosus DSM 32992 (oral origin), L. rhamnosus DSM 32991 (oral), L. jensenii 22B42 (vaginal), and L. rhamnosus PB01 (vaginal). The difference between L. rhamnosus DSM 32992 and the other three strains was statistically significant (p < 0.001). The Candida spp. differed in susceptibility; C. parapsilosis was highly inhibited, while C. krusei was not or slightly inhibited. The oral L. rhamnosus DSM 32992 and DSM 32991 strains showed the lowest pH-values.
Conclusion: Screening of probiotic lactobacilli showed significant strain-dependent variations in their antifungal capacity in a pH-dependent mode. Two strains of oral origin were most effective. A further characterization seems justified to elaborate on their probiotic properties.
Introduction
Candida species (spp.) are ubiquitous commensals taking part of a healthy oral microbiota. C. albicans is the predominant specie and is believed to be present in its natural blastospore form in around 50% of the healthy population [Citation1,Citation2]. In healthy individuals, the oral microbiota appears to prevent Candida spp. to shift from harmless commensals to disease-causing pathogens. However, certain conditions may disrupt the homeostatic bacteriome–mycobiome relationship and predispose the Candida spp. to become pathogenic and cause disease in the oral mucosa, termed oral candidosis [Citation3]. While C. albicans is the main contributor to infection, the incidence of oral infection due to non-albicans spp. have increased over the last decades, and the most frequently isolated strains associated with disease are C. glabrata, C. krusei, C. tropicalis, C. dubliniensis, and C. parapsilosis [Citation4,Citation5].
Currently, treatment options of oral candidosis include topical and systemic antimycotics. However, the American Centers for Disease Control and Prevention has reported that about 7% of all Candida bloodstream isolates are resistant to fluconazole [Citation6]. Although C. albicans is the most common cause of severe Candida infections, resistance is most common in other species, particularly C. glabrata and C. parapsilosis [Citation6]. According to the World Health Organization, Candida infection is a significant concern for human health in vulnerable populations due to the emergence of new resistance mechanisms in the microorganisms. In most countries where data are available, drug resistance appears to be higher among non-albicans species than among C. albicans [Citation7]. Hence, the search of alternative antimycotic treatments to prevent and combat oral mucosal infections of both C. albicans and non-albicans spp. is highly relevant.
Probiotic bacteria have been proposed as a supplement to conventional therapies to prevent and combat oral Candida-infection [Citation8–10]. Probiotic bacteria are defined as ‘live microorganism that, when administered in adequate amounts, confer a health benefit on the host’ [Citation11]. The most investigated probiotics for anti-Candida activity belong to the genera Lactobacillus and Bifidobacterium, but also Streptococcus has been proposed [Citation12]. Laboratory studies have shown that probiotic lactobacilli can inhibit C. albicans growth [Citation13,Citation14], interfere with the C. albicans biofilm formation on dentures [Citation15], and to interfere in hyphae formation [Citation16]. In addition, Tan and coworkers [Citation17] showed that sterile spent media of L. gasseri BF and L. rhamnosus BF could disrupt biofilm formation of C. tropicalis BF, C. krusei BF, and C. parapsilosis BF and reduce their metabolic activity. It has also been demonstrated that cell-free supernatants of the probiotic L. pentosus strain LAP1 could inhibit the growth of C. albicans, C. tropicalis, and C. krusei in vitro [Citation18].
Several clinical trials have confirmed that regular probiotic administration can decrease the Candida cell counts in samples from the oral cavity, in particular among elderly individuals prone to infections [Citation8,Citation19–22] and be a possible supplement to conventional antimycotic treatment [Citation23,Citation24]. However, the genera of probiotics used in the trials are wide-spread, and the choice of an appropriate type of probiotic for this specific target is challenging. Recently, we showed significant differences in the antifungal properties of two L. reuteri strains (ATCC PTA 5289 and DSM 17938) [Citation9]. This finding emphasizes that the probiotic strain-specificity cannot be disregarded, and that the search for appropriate probiotic strains with strong anti-Candida properties for oral application is warranted. The primary aim of this study was, therefore, to screen the antifungal activity of 14 selected probiotic Lactobacillus candidate strains, isolated from the human oral cavity or vagina, against six opportunistic Candida spp. in vitro. A secondary aim was to study the acid production abilities of the four best-performing lactobacilli in the growth inhibition assay with aid of pH microsensor measurements through Candida and Lactobacillus co-cultures.
Materials and methods
Strains and culture conditions
Fourteen selected Lactobacillus spp. (Deerland Probiotics & Enzymes A/S, Hundested, Denmark) were used in this study together with six clinical Candida spp. from the Department of Clinical Microbiology, Copenhagen University Hospital, Copenhagen, Denmark, and six control spp. from the Culture Collection, University of Gothenburg, Sweden (). The lactobacilli were initially cultured on de Man Rogosa Sharpe (MRS) agar (Oxoid Ltd., Basingstoke, Hampshire, UK) for 24 hours (h) in an anaerobic chamber at 37°C (10% H2, 5% CO2 and 85% N2). The Candida strains were cultured on BD Difco™ Sabouraud Dextrose (SD) agar (Becton, Dickinson and Company, Sparks, MD, USA) for 24 h in ambient air at 37°C.
Table 1. List of Lactobacillus spp. and Candida spp. used in the study
Growth inhibition assay
The growth inhibition assay was performed as described earlier [Citation9]. In brief, one distinct colony of overnight cultured lactobacilli was transferred to 5 mL MRS broth (Oxoid Ltd., Basingstoke, Hampshire, UK) and incubated at 37°C for 24 h under anaerobic conditions. The following day the lactobacilli were harvested by centrifugation at 2,000 rpm for 10 min at room temperature. The supernatants of the Lactobacillus strains were obtained after centrifugation and destroyed. The pellets were washed three times in phosphate-buffered saline (PBS), and the OD was adjusted to 1.8 at 630 nm (Genesys™ 10S UV-Vis Spectrophotometer, Thermo Fisher Scientific, Waltham, MA, USA), corresponding to approximately 109 cfu/mL. The cultures were then serially diluted in MRS broth in sixfold steps. One mL of the supernatants, undiluted suspensions and cell suspensions corresponding to approximately 107 and 105 CFU/mL were added to 24 mL sterilized molten MRS agar (~45°C) in Petri dishes and the agar could solidify. The plates were incubated overnight at 37°C under anaerobic conditions. One single colony of each of the overnight cultured Candida strains was added to 5 mL broth and aerobically incubated at 37°C for 24 h. The following day, one additional layer of 25 mL of molten sterile SD agar was poured on top of the MRS agar with grown lactobacilli and could solidify and air-dry for 3 h in room temperature. The overnight cultured Candida strains were diluted in SD broth to a final OD of 0.2 at 500 nm. The Candida suspensions were stamped on the plates with a Steers steel-pin replicator (CMI-Promex ICN, Pedricktown, NJ, USA) and left to dry for 2 h at room temperature. The plates were subsequently aerobically incubated overnight at 37°C. As controls, the Candida strains were stamped on the top of plates with no lactobacilli within the bottom MRS agar layer.
The assays were carried out in duplicates and repeated three times on different occasions. The results were evaluated with a 4-step score, modified from Simark-Mattson et al. [Citation25]: Score 0 = complete growth inhibition (no visible colonies), Score 1 = almost total growth inhibition (colonies slightly visible), Score 2 = slight growth inhibition (colonies are clearly visible but smaller than at the control plate), and Score 3 = no growth inhibition (colonies equal to those at the control plate). Two observers (MRJ and PTR) scored the plates independently, and in case of disagreement, consensus was reached through discussion.
Microsensor measurement of pH
Acid production (measured by pH) of the four best-performing lactobacilli in the growth inhibition assay and the effect of pH on Candida growth was measured with pH microelectrodes (pH-100 and ref-100; Unisense A/S, Århus, Denmark) with a linear response between pH 4–9 and a 90% response time of <10 s and a detection limit of 0.01 pH units. Measurements were performed using a modification of a previously described procedure [Citation9] in selected plates from the growth inhibition test. In brief, the electrodes were mounted in a motorized PC-controlled profiling setup (MM33 and MC-232, Unisense A/S), and positioning and data acquisition were controlled by dedicated software (SensorTrace Profiling, Unisense A/S). The pH microsensor was calibrated against buffers of pH 4 and 7 at room temperature with a linear slope of −56.1 mV/pH unit. The pH was measured on the final day of the growth inhibition test. Before measuring, the pH and reference electrodes were placed approximately 2 mm above the surface of the Candida colonies. The pH was measured from the surface of the colonies in 100 µm increments until the depth of maximum 4.5 mm into the agar to make sure the sensors had reached the bottom agar layer containing the lactobacilli. Selection of the plates was based on the results from the interference test; the plates with the highest inhibition scores for each Lactobacillus spp. were selected regardless of concentration (CK and PØJ). The pH was measured through dense, slightly inhibited colonies of Candida (C. krusei and C. tropicalis) and through vague, almost completely inhibited colonies (C. albicans, C. glabrata, C. dubliniensis, and C. parapsilosis) incubated on plates with L. rhamnosus DSM 32992, L. rhamnosus DSM 32991, L. jensenii 22B42, and L. rhamnosus PB01, respectively. In addition, pH was measured in control plates containing only the lactobacilli in the bottom agar layer, in a control plate with only Candida strains, and lastly in a control agar plate without lactobacilli or Candida strains.
Statistical analyses
All data were processed with SAS Enterprise guide software (version 7.1, SAS Institute Inc., Cary, NC, USA). A p-value <0.05 (two-sided) was considered statistically significant. For descriptive statistics, the growth inhibition scores for the lactobacilli at different doses are presented as the median score. The frequency of growth inhibition scores 0–3 for each Lactobacillus strain, for each dose, and for each Candida strain is presented in percentage distribution. A Poisson regression model was employed to test the influence of the Lactobacillus strains, Candida strains and dose variables on the growth inhibition score. Based on the results from the model, the four lactobacilli displaying the lowest growth inhibition scores were subjected to Chi-square tests.
Results
Growth inhibition
All the 14 Lactobacillus strains proved to inhibit the growth of the Candida spp., but some were more effective than others, with a substantial Candida ssp. inhibition variation (, Suppl. Table S1). The four best-performing lactobacilli were L. rhamnosus DSM 32992, which demonstrated the highest number of score 0 and 1, followed by L. rhamnosus DSM 32991, L. jensenii 22B42, and L. rhamnosus PB01. The difference between L. rhamnosus DSM 32992 and the other three strains was statistically significant (p < 0.001). In general, the growth inhibition was almost equal for the cell concentrations 109 and 107 CFU/mL, while the lowest concentration (105 CFU/mL) appeared to be least effective (Suppl. Table S1, Suppl. Figure S1). The various Candida spp. displayed contrasting susceptibility; the growth of the clinical and control strains of C. parapsilosis was highly inhibited, while the two C. krusei strains presented no growth inhibition or only slight growth inhibition (, Suppl. Table S1). The clinical Candida isolates and the control strains of the same species presented a similar susceptibility in the assays except for C. tropicalis for which the clinical isolate seemed to be more inhibited than the control strain.
Figure 1. Frequency distribution (%) of growth inhibition score 0–3 for each Lactobacillus spp. (all concentrations and all Candida spp.). Results are based on duplicate assays, repeated at three separate occasions
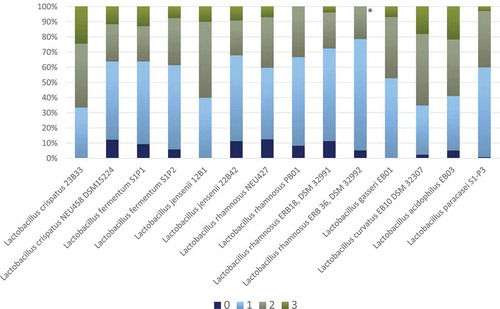
Figure 2. Frequency distribution (%) of growth inhibition score 0–3 for each Candida spp. based on agar overlay interference tests with all 14 Lactobacillus spp. (all concentrations). Results are based on duplicate assays, repeated at three separate occasions
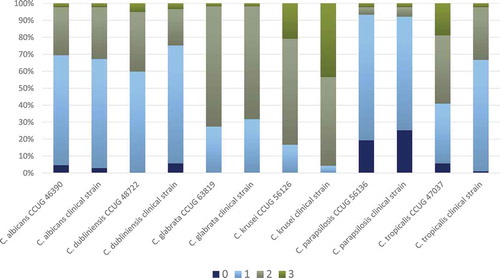
Microsensor pH measurements
Microsensor measurements of pH were performed for the four above-mentioned best-performing Lactobacillus strains: L. rhamnosus DSM 32992, L. rhamnosus DSM 32991, L. jensenii 22B42, and L. rhamnosus PB01, of which the two former were isolated from the oral cavity and the two latter had vaginal origin. The pH of the plain double-layered agar was 5.8, and pH measurements from L. rhamnosus DSM 32992, L. rhamnosus DSM 32991, and L. rhamnosus PB01 generally showed lower pH values than L. jensenii 22B42 ranging from 3.5 to 3.8 (Suppl. Figure S2). In plates with only Candida spp. incubated, pH measured through dense colonies of C. krusei and C. tropicalis was approximately 6.8 at the surface of the colonies, reaching 5.8 around 700–1,000 µm down in the agar and remained stable. A similar picture was obtained when the pH-value was measured through C. albicans colonies, but the pH drop seemed to be more rapid, reaching 5.8 approximately 500 µm inside the agar. The measurements for L. rhamnosus DSM 32992 (107 CFU/mL) through C. albicans and non-C. albicans colonies are presented in . The surface pH was substantially lower compared with the Candida spp. incubated plates and the pH dropped rapidly through the first 400 µm. However, for the C. krusei and C. tropicalis reference strains, this drop was delayed and less dramatic (). The pH profiles of the remaining three Lactobacillus strains inoculated with Candida spp. at the top agar layer showed very similar patterns (data not shown).
Figure 3. Micro-sensor measurement of pH with selected Candida strains with L. rhamnosus DSM 32992 (107 CFU/mL) in the bottom agar layer of the co-cultured agar overlay assay. Zero on the vertical axis represents the first measurement from the sensor of either a Candida colony or agar. No changes in pH values appeared for any of the strains at deeper levels in the agar
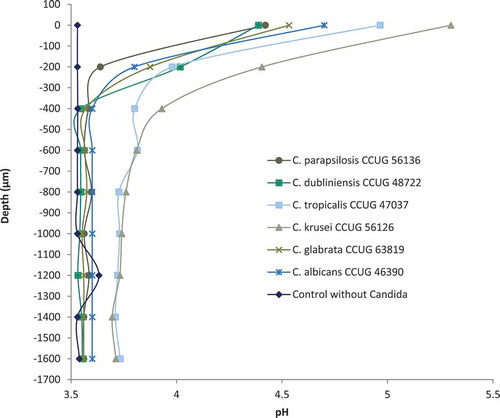
Discussion
During recent years, the use of probiotic bacteria to control oral Candida colonization has been investigated as an adjunct therapeutic strategy to prevent infections in susceptible individuals [Citation24,Citation26,Citation27]. In this study, we investigated the growth inhibition of 14 selected Lactobacillus strains isolated from the human oral cavity or vagina. We found a clear but strain-dependent effect on Candida growth which was in harmony with several previous in vitro experiments [Citation9,Citation17,Citation26,Citation28,Citation29]. Interestingly, three of the best performing strains were L. rhamnosus strains, and the two best growth inhibitors, L. rhamnosus DSM 32992 and L. rhamnosus DSM 32991, were isolated from the oral cavity. L. rhamnosus is one of the most investigated probiotic Lactobacillus spp. and especially, the strain L. rhamnosus GG ATCC 53103 (LGG), isolated from a healthy human gastrointestinal tract, has shown remarkable probiotic properties [Citation30,Citation31]. Oral candidosis is a result of bacterial-fungal dysbiosis appearing when the microbial homeostasis is disrupted due to antibiotic treatment, local or systemic immune disturbances, smoking, hyposalivation, use of dentures, and poor oral hygiene [Citation32]. To our knowledge, only one randomized clinical trial (RCT) has investigated the antifungal effect of a single strain of L. rhamnosus in the oral cavity [Citation22]. When added to milk, L. rhamnosus SP1 provided a significant reduction in the severity of denture stomatitis and significantly reduced Candida counts in the probiotic group after 6 months’ intervention [Citation22]. Four RCT’s [Citation19–21,Citation33] have shown decreased Candida counts after intervention with different L. rhamnosus strains; however, in these trials the strains were combined with non-L. rhamnosus probiotic species, and the antifungal effect can therefore not completely be ascribed to one probiotic strain. The beneficial clinical effects are however supported by laboratory studies showing that L. rhamnosus strains can protect oral epithelial tissue from damage, caused by C. albicans, by impacting its major virulence factors, including adhesion, competition for nutrients, invasion and hyphae formation [Citation26,Citation30,Citation34].
The probiotic mechanisms of action against C. albicans are not fully clear [Citation27] but our present results suggest that some of the inhibiting effects are pH-driven. Similar observations have been made before [Citation9,Citation28]. It is thought that colonization of C. glabrata and C. albicans depends on their ability to cope with the presence of lactic and acetic acids produced by commensal microbiota [Citation35]. Lactobacilli produce different weak organic acids (WOAs), mainly lactic and acetic acids, but the capability and rate of acid production are strain-specific [Citation36]. In our study, L. rhamnosus DSM 32992 and L. rhamnosus DSM 32991 generally showed the lowest pH values indicating the highest acid production of the selected lactobacilli. The findings correspond well with the results from the growth inhibition test, in which these two strains exhibited the best inhibitory effect. This indicates that acid production plays an important role in the inhibition of Candida growth in agar overlay interference assay and indicates that the acids produced by the lactobacilli diffuse into the upper agar layer and interfere with Candida growth and survival. Cottier and co-workers [Citation37] investigated the transcriptional stress response of C. albicans to WOAs in vitro and found the response to be a significant enrichment of genes involved in iron homeostasis, and down-regulation of RNA synthesis and ribosome biogenesis genes. The findings were mostly apparent upon chronic exposure to the acids and suggested that exposure of C. albicans to WOAs over time might shift the cells into a ‘starvation-like’ metabolic state with low transcription, translation and growth of the cell [Citation37]. In a study by Köhler et al. [Citation14], C. albicans growth was suppressed at low pH by the supernatant of the lactobacilli; however, pH neutralization of the culture filtrate completely abrogated the inhibitory effects of the supernatants. It is likely that low pH inhibits the transition between the blastospore form and the more invasive hyphae form of C. albicans and increases the intracellular concentration of protons in the yeasts. This may lead to an increased activity of an energy-consuming plasma membrane H+-ATPase that exhausts the available energy for growth and metabolism, leading to growth inhibition and, finally, cell death of the yeast [Citation38]. Our experiments revealed that the tested C. krusei and C. tropicalis strains were able to resist and neutralize the acids produced by the lactobacilli. This may be understood in view of an in vitro study by Halm et al. [Citation39] who found that lactic acid only induced a short-term (few seconds) pH change intracellularly in C. krusei. Fungal resistance to organic acids could be caused by extracellular production of ammonia [Citation40,Citation41], less permeable plasma membranes to lactic acid, higher buffer capacity inside the fungal cells, or higher H+-ATPase capacity [Citation38].
Results from in vitro inference studies with single bacteria or multi-species biofilms must be considered with great caution taken the complex environment of the oral cavity into account. The agar overlay interference assay allows screening of multiple strains at a time on a single plate, with different Lactobacillus strains in the bottom agar laying at different cell concentrations. The assay is a relatively simple, yet a robust and well-proven method for screening purposes of probiotic bacteria against oral pathogens [Citation9,Citation13,Citation25]. In our experiments, we modified the growth inhibition scoring system to allow for a more sensitive screening. Based on our previous experience [Citation9], we separated the original score 1 (‘slight inhibition’) into score 1 ‘almost total growth inhibition (colonies slightly visible)’ and score 2 ‘slight growth inhibition (colonies are clearly visible but smaller than at the control plate)’. The advantage of this modified system was that we were able to distinguish the growth inhibition capacity of the 14 probiotic candidates but the downside was that the results were harder to compare with the results found in comparable studies using the same inhibition assay [Citation9,Citation13,Citation42].
There is evidence to support the hypothesis that the efficacy of probiotics is both strain-specific and disease-specific [Citation43]. Therefore, our present the finding of two probiotic L. rhamnosus candidates (strain-specificity) with excellent antifungal capabilities (disease-specificity) isolated from the oral cavity, the environment where they are intended to exhibit their probiotic action (target site-specificity), was promising. Future studies on the characteristics of the four candidates, or combinations thereof, seems motivated to further unveil the probiotic and physiological properties including the risk of transmission of antibiotic resistance genes. In addition to clinically demonstrated health benefits and safety for human use, the persistence of cell viability and probiotic activities throughout the processing, handling and storage needs to be investigated [Citation31].
Conclusion
All 14 Lactobacillus strains employed in this in vitro screening demonstrated antifungal activity and inhibited the growth of six Candida spp. to varying degrees. The effect seemed pH-driven and two L. rhamnosus strains isolated from the oral cavity showed the strongest growth inhibition and acid production ability among the selected strains. The findings are promising for future clinical employment of these strains in the prevention of oral C. albicans and non-C. albicans infections; however, further investigations and characterization of the strains are needed to elaborate on their probiotic properties.
Supplemental Material
Download MS Word (58.5 KB)Acknowledgments
The authors would like to thank Mr Erik Brandsborg and Mr Lasse Sommer Mikkelsen, Deerland Probiotics & Enzymes A/S for providing the Lactobacillus spp., for valued support and fruitful discussions during the studies. Furthermore, we thank laboratory technician Camilla Gündüz for her valuable assistance in the laboratory. The experimental products were generously provided by Deerland Probiotics & Enzymes A/S, Hundested, Denmark.
Disclosure statement
The study was partly funded by Deerland Probiotics & Enzymes A/S, Hundested, Denmark. However, the study was solely performed of scientific interest, and the authors had no financial or political conflicts of interest in the study. Mette Rose Jørgensen is currently employed at Novozymes A/S, Bagsværd, Denmark.
Supplementary material
Supplemental data for this article can be accessed here.
Additional information
Funding
References
- Rindum JL, Stenderup A, Holmstrup P. Identification of Candida albicans types related to healthy and pathological oral mucosa. J Oral Pathol Med. 1994;23:406–8.
- Kragelund C. Exploiting new knowledge of Candida infection for future antifungal combat. Oral Dis. 2017;23:543–547.
- Oever JT, Netea MG. The bacteriome-mycobiome interaction and antifungal host defense. Eur J Immunol. 2014;44:3182–3191.
- Sardi JC, Scorzoni L, Bernardi T, et al. Candida species: current epidemiology, pathogenicity, biofilm formation, natural antifungal products and new therapeutic options. J Med Microbiol. 2013;62:10–24.
- Pfaller MA, Andes DR, Diekema DJ, et al. Epidemiology and outcomes of invasive candidiasis due to non-albicans species of Candida in 2,496 patients: data from the Prospective Antifungal Therapy (PATH) registry 2004-2008. PLoS One. 2014;9(7):e101510. .
- U.S. Department of Health and Human Services, Centers for Disease Control and Prevention. Antibiotic resistance threats in the USA 2019. [cited 2020 Apr 14]. Available from: https://www.cdc.gov/drugresistance/pdf/threats-report/2019-ar-threats-report-508.pdf
- World Health Organization. Antimicrobial resistance global report on surveillance. 2014 [cited 2020 Apr 14]. Available from: https://apps.who.int/iris/bitstream/handle/10665/112642/9789241564748_eng.pdf;jsessionid=3DFA76135096412FF7041954E144562E?sequence=1
- Kraft-Bodi E, Jørgensen MR, Keller MK, et al. Effect of probiotic bacteria on oral Candida in frail elderly. J Dent Res. 2015;94(9 Suppl):181–186. .
- Jørgensen MR, Kragelund C, Jensen PØ, et al. Probiotic Lactobacillus reuteri has antifungal effects on oral Candida species in vitro. J Oral Microbiol. 2017;9:1274582.
- Hu L, Mao Q, Zhou P, et al. Effects of Streptococcus salivarius K12 with nystatin on oral candidiasis-RCT. Oral Dis. 2019;25:1573–1580.
- Sanders ME. Probiotics: definition, sources, selection, and uses. Clin Infect Dis. 2008;46(Suppl 2):S58–61. discussion S144-51. .
- Matsubara VH, Bandara HM, Mayer MP, et al. Probiotics as antifungals in mucosal candidiasis. Clin Infect Dis. 2016;62:1143–1153.
- Hasslöf P, Hedberg M, Twetman S, et al. Growth inhibition of oral mutans streptococci and candida by commercial probiotic lactobacilli - an in vitro study. BMC Oral Health. 2010;10:18.
- Köhler GA, Assefa S, Reid G. Probiotic interference of Lactobacillus rhamnosus GR-1 and Lactobacillus reuteri RC-14 with the opportunistic fungal pathogen Candida albicans. Infect Dis Obstet Gynecol. 2012. DOI:10.1155/2012/636474
- Ujaoney S, Chandra J, Faddoul F, et al. In vitro effect of over-the-counter probiotics on the ability of Candida albicans to form biofilm on denture strips. J Dent Hyg. 2014;88:183–189.
- Murzyn A, Krasowska A, Stefanowicz P, et al. Capric acid secreted by S. boulardii inhibits C. albicans filamentous growth, adhesion and biofilm formation. PLoS One. 2010;5:e12050.
- Tan Y, Leonhard M, Moser D, et al. Inhibitory effect of probiotic lactobacilli supernatants on single and mixed non-albicans Candida species biofilm. Arch Oral Biol. 2018;85:40–45.
- Aarti C, Khusro A, Varghese R, et al. In vitro investigation on probiotic, anti-Candida, and antibiofilm properties of Lactobacillus pentosus strain LAP1. Arch Oral Biol. 2018;89:99–106.
- Hatakka K, Ahola AJ, Yli-Knuuttila H, et al. Probiotics reduce the prevalence of oral Candida in the elderly–a randomized controlled trial. J Dent Res. 2007;86:125–130.
- Ishikawa KH, Mayer MP, Miyazima TY, et al. A multispecies probiotic reduces oral Candida colonization in denture wearers. J Prosthodont. 2015;24:194–199.
- Miyazima TY, Ishikawa KH, Mayer M, et al. Cheese supplemented with probiotics reduced the Candida levels in denture wearers-RCT. Oral Dis. 2017;23:919–925.
- Lee X, Vergara C, Lozano CP. Severity of Candida-associated denture stomatitis is improved in institutionalized elders who consume Lactobacillus rhamnosus SP1. Aust Dent J. 2019;64:229–236.
- Li D, Li Q, Liu C, et al. Efficacy and safety of probiotics in the treatment of Candida-associated stomatitis. Mycoses. 2014;57:141–146.
- Hu L, Zhou M, Young A, et al. In vivo effectiveness and safety of probiotics on prophylaxis and treatment of oral candidiasis: a systematic review and meta-analysis. BMC Oral Health. 2019;19:140.
- Simark-Mattsson C, Emilson CG, Hakansson EG, et al. Lactobacillus-mediated interference of mutans streptococci in caries-free vs. caries-active subjects. Eur J Oral Sci. 2007;115:308–314.
- Rossoni RD, de Barros PP, de Alvarenga JA, et al. Antifungal activity of clinical Lactobacillus strains against Candida albicans biofilms: identification of potential probiotic candidates to prevent oral candidiasis. Biofouling. 2018;34:212–225.
- Ribeiro FC, Rossoni RD, de Barros PP, et al. Action mechanisms on Candida spp. and candidiasis prevention: an update. J Appl Microbiol. 2019. DOI:10.1111/jam.14511.
- Jiang Q, Stamatova I, Kari K, et al. Inhibitory activity in vitro of probiotic lactobacilli against oral Candida under different fermentation conditions. Benef Microbes. 2015;6:361–368.
- Salari S, Almani PGN. Antifungal effects of Lactobacillus acidophilus and Lactobacillus plantarum against different oral Candida species isolated from HIV/AIDS patients: an in vitro study. J Oral Microbiol. 2020;12(1):1769386.
- Allonsius CN, van den Broek MFL, De Boeck I, et al. Interplay between Lactobacillus rhamnosus GG and Candida and the involvement of exopolysaccharides. Microb Biotechnol. 2017;10:1753–1763.
- Capurso L. Thirty years of Lactobacillus rhamnosus GG: A review. J Clin Gastroenterol. 2019;53(Suppl 1):S1–S41.
- Pankhurst CL. Candidiasis (oropharyngeal). BMJ Clin Evid. 2012;2012:1304.
- Ahola AJ, Yli-Knuuttila H, Suomalainen T, et al. Short-term consumption of probiotic-containing cheese and its effect on dental caries risk factors. Arch Oral Biol. 2002;47:799–804.
- Mailänder-Sánchez D, Braunsdorf C, Grumaz C, et al. Antifungal defense of probiotic Lactobacillus rhamnosus GG is mediated by blocking adhesion and nutrient depletion. PLoS One. 2017;12:e0184438.
- Lourenco A, Pedro NA, Salazar SB, et al. Effect of acetic acid and lactic acid at low pH in growth and azole resistance of Candida albicans and Candida glabrata. Front Microbiol. 2018;9:3265.
- Zangl I, Pap I-J, Aspöck C, et al. The role of Lactobacillus species in the control of Candida via biotrophic interactions. Microb Cell. 2020;7:1–14.
- Cottier F, Tan AS, Chen J, et al. The transcriptional stress response of Candida albicans to weak organic acids. G3 (Bethesda). 2015;5:497–505.
- Holyoak CD, Stratfor M, McMullin Z, et al. Activity of the plasma membrane H+-ATPase and optimal glycolytic flux are required for rapid adaptation and growth of Saccharomyces cerevisiae in the presence of the weak-acid preservative sorbic acid. Appl Environ Microbiol. 1996;62:3158–3164.
- Halm M, Hornbaek T, Arneborg N, et al. Lactic acid tolerance determined by measurement of intracellular pH of single cells of Candida krusei and Saccharomyces cerevisiae isolated from fermented maize dough. Int J Food Microbiol. 2004;94:97–103.
- Zikanova B, Kuthan M, Ricicova M, et al. Amino acids control ammonia pulses in yeast colonies. Biochem Biophys Res Commun. 2002;294:962–967.
- Vylkova S, Carman AJ, Danhof HA, et al. The fungal pathogen Candida albicans autoinduces hyphal morphogenesis by raising extracellular pH. MBio. 2011;2:e00055–11.
- Keller MK, Hasslof P, Stecksen-Blicks C, et al. Co-aggregation and growth inhibition of probiotic lactobacilli and clinical isolates of mutans streptococci: an in vitro study. Acta Odontol Scand. 2011;69:263–268.
- McFarland LV, Evans CT, Goldstein EJC. Strain-specificity and disease-specificity of probiotic efficacy: A systematic review and meta-analysis. Front Med. 2018;5:124.