ABSTRACT
Background
Poor oral hygiene and the increased incidence and severity of periodontitis may exacerbate SARS-CoV-2 infection. The aim was to evaluate the oral microbiota of 60 participants divided into groups: COVID-19 convalescents who received antibiotics during hospitalization (I), COVID-19 convalescents without antibiotic therapy (II) and healthy individuals (III).
Materials and Methods
Dental examination was conducted, and oral health status was evaluated using selected dental indexes. Clinical samples (saliva, dorsal swabs, supragingival and subgingival plaque) were collected and used for metagenomic library to the next-generation sequencing (NGS) preparation.
Results
Each of the clinical materials in particular groups of patients showed a statistically significant and quantitatively different bacterial composition. Patients from group I showed significantly worse oral health, reflected by higher average values of dental indexes and also a higher percentage of Veillonella, Tannerella, Capnocytophaga and Selenomonas genera in comparison to other groups. Additionally, a statistically significant decrease in the amount of Akkermansia type in both groups with COVID-19 was observed for all materials.
Conclusions
The primary factor affecting the composition of oral microbiota was not the SARS-CoV-2 infection itself, but the use of antibiotic therapy. The increased percentage of pro-inflammatory pathogens observed in COVID-19 patients underscores the importance of preventing periodontal disease and improving oral hygiene in the future.
Introduction
Oral microbiome consists of approximately 800 species of bacteria [Citation1] and is the second largest (after the large intestine) community of microorganisms [Citation2]. In healthy individuals, numerous species of aerobic and anaerobic bacteria form a multispecies biofilm whose composition varies depending on the area of the oral cavity. This means that the tongue, palate, cheeks, teeth and periodontal pockets have their own unique microbiota [Citation2].
Introduction of the next-generation sequencing (NGS) allows for a qualitative and semi-quantitative determination of the diversity of microorganisms depending on the analysed factors (e.g. diet, hygiene [Citation3]) diseases (e.g. coeliac disease, diabetes, SARS-CoV-2 infection) and treatment [Citation4–6]. One of the best-known therapies that disrupt the composition of the microbiota is antibiotic therapy [Citation7]. Antibiotic-induced changes in the microbial composition may have a negative impact on the health of the host, for example by reducing the diversity of microbes, changing the functional characteristics of the microbiota, creating antibiotic-resistant strains, and making the host more susceptible to infection [Citation8–10]. While numerous reports on the impact of antibiotic therapy on the microbiota of the gastrointestinal tract, mainly the intestines, are available [Citation11], its impact on the oral microbiota remains poorly understood [Citation7].
Oral diseases, especially periodontitis, are associated with the release of pro-inflammatory cytokines and mediators of tissue destruction, which may influence systemic inflammation [Citation12]. It has been reported that the increased incidence and severity of periodontitis and the related poor oral hygiene may contribute to the exacerbation of SARS-CoV-2 infection [Citation12]. However, there are no reports on their possible impact on potential changes in the microbiota in the course of the disease and therapy used in COVID-19.
Therefore, the aim of the present study was to assess the oral microbiota, using the next-generation sequencing method, in patients after hospitalisation for COVID-19, treated and not treated with antibiotics, considering the impact of oral health based on dental indices.
Materials and methods
Study population and settings
The study included 60 people (36 men and 24 women), aged 28–87. Participants were divided into three groups: group I – COVID-19 convalescents who received antibiotics during hospitalisation (n = 17), group II – COVID-19 convalescents who did not receive antibiotics during hospitalisation (n = 23) and group III – healthy patients who have not been infected with the SARS-CoV-2 virus and not treated with antibiotics (control group, n = 20).
Patients without systemic diseases or patients whose only disease was hypertension were qualified for the study. Patients from groups I and II were previously treated in hospital conditions due to COVID-19 disease at the Temporary Hospital for COVID-19 patients at the Trauma Center of Emergency Medicine and Disasters of the University Hospital in Krakow. The patients were treated in accordance with the recommendations of the Polish Ministry of Health, based on the recommendations of the World Health Organization [Citation13]. Patients from group I were mainly treated with ceftriaxonum (i.v. 2 g/12 h). Four patients from I group were treated in different schemes: two of them with levofloxacin alone (i.v. 500 mg/12 h), third with ceftriaxone (i.v. 2 g/12 h) and ciprofloxacin (i.v. 400 mg/12 h), fourth with ceftriaxonum (i.v. 2 g/12 h) and levofloxacin (i.v. 500 mg/12 h). The treatment lasted 6–7 days and depended on clinical symptoms and inflammatory markers based on complete blood count and CRP. Biological material was collected on the day of discharge, when the patients were considered convalescents (negative result of the PCR test for SARS-CoV-2) and provided written consent to participate in the study.
Patients of the University Dental Clinic in Kraków were recruited to the third group.
The dental inclusion criterion for the study was as follows: having at least six teeth, not performing oral hygiene activities and not eating for 12 h prior to sampling. For the control group, as for group II, an additional inclusion criterion was not taking antibiotics for at least 3 months before the study. In addition, each of the participants declared that in the period preceding the study (at least 3 months) they did not take probiotics. Lack of any of the criteria resulted in exclusion from the study.
Dental examination
Each participant underwent a dental examination. On its basis, the selected indices were determined: DMFT (Decayed, Missing and Filled Teeth index) [Citation14], D number (Decayed Teeth number) [Citation14] and PI (Plaque Index simplified index) [Citation15]. DMFT Index reflects individual caries exposition in the lifespan. According to the definition is the sum of teeth: with caries (D), teeth extracted due to caries (M) and teeth with fillings (F) [Citation14]. The number of carious lesions (D) is the first component of the DMFT index [Citation14]. Plaque Index is the periodontal index of oral hygiene. In order to calculate the PI index, the tooth is divided into four surfaces (mesial, distal, lingual, buccal). The presence of bacterial dental and gingival plaque is tested on each surface. The value of the index is calculated according to the formula PI = (sum of tooth surfaces with plaque/number of all examined surfaces) × 100% [Citation15].
Collection of biological material
Four samples were collected from each subject: unstimulated saliva, dorsal swabs, supragingival and subgingival plaque. Biological material was collected according to the protocol of Caselli E et al. [Citation2]. Dental examination, material collection, its protection and transport were performed by a dentist, after prior standardisation of the method.
Samples preparation, nucleic acid isolation and 16S rRNA sequencing
Collected samples were placed in cool condition (−20°C) and delivered within 1 h to the Department of Molecular Medical Microbiology, Chair of Microbiology of the Jagiellonian University Medical College and then frozen at −80°C until the time of nucleic acid extraction. The samples were used for bacterial DNA isolation and for the next-generation sequencing preparation as described previously [Citation16].
Bioinformatics analysis and statistical analysis
Raw sequencing reads were controlled for quality (FastQC software; Babraham Bioinformatics) and filtered to remove poor quality read ends using FASTQ Toolkit (Illumina). The filtered reads were further analysed using BaseSpace (Illumina) 16S Metagenomics application which is a high-performance implementation of the Ribosomal Database Project (RDP) Classifier described by Wang Q. et al. [Citation17]. In brief, the analysis included matching the reads to primer sequences, excluding non-target reads, filtering the low quality reads and merging the paired end reads. Then, chimeric reads were detected using UCHIME and further assigned to the taxonomic classes using RDP algorithm which is based on Bayesian approach [Citation17]. Classification was made with respect to the RefSeq RDP 16S v3 database [Citation18]. Counts of reads classified into specific taxonomic units were filtered for frequency and used to assess Alpha and Beta diversity using MicrobiomeAnalyst software [Citation19]. Alpha diversity, expressed in the Shannon index, was tested for significance using Kruskal–Wallis test, and the beta diversity, using the Shannon index, was subsequently tested for significance using PERMANOVA. An EdgeR [Citation20] software was used to normalise read counts and to perform differential analysis with exact tests. Obtained p-values were corrected for multiple testing using false discovery rate (FDR) procedure [Citation21]. When evaluating the statistical power of this analysis, we found that with our 240 samples we fully achieve statistical power exceeding 90% [Citation22].
Quantitative variables were compared between study groups using one-way analysis of variance (ANOVA) with Welch adjustment if required. For statistically significant variables with respect to DMFT indices, Tukey post hoc test was performed, while for the D number and PI, Games-Howell post hoc test was performed due to the lack of homogeneity of variance. On the other hand, for categorised variables, the χ2 test was performed. Statistical analysis was prepared using the IBM SPSS Statistics 27 software. The significance level was α = 0.05.
Correlation between dental indices and selected microbiota abundances was calculated using JASP (https://jasp-stats.org/) statistical software, following data distribution evaluation using Shapiro–Wilk test. Spearman rank correlation coefficient was used for this analysis.
Ethics consideration
Informed consent was obtained from all participants involved in the study. This research has been approved by the Jagiellonian University Ethical Committee (no. 1072.6120.333.2020 of 7 December 2020).
Results
Characteristics of the study groups in terms of demographics and the condition of the oral cavity
There were no statistically significant differences between the study groups (I vs. II vs. III) in terms of gender (P = 0.069) and age (P = 0.580). However, for dental indices: D (P < 0.001), DMFT (P < 0.001) and PI (P = 0.011), statistically significant differences were observed. Groups I and II were statistically significantly different from group III in terms of DMFT (P < 0.001 and P < 0.001, respectively) and D number (P < 0.001 and P < 0.001, respectively). In groups I and II, these indices were higher than in group III. In the case of PI, only groups I and III were statistically significantly different (P = 0.008). The PI was the highest in group I ().
Table 1. The result of one-way analysis of variance (ANOVA) for age and seed dental indices between the study groups.
Taxonomic and biodiversity analysis of the mouth microbiota in the studied groups
Briefly, sequencing of 240 samples generated more than 13.8 M raw paired end reads, resulting in 56.5 K PE reads per sample on average. After filtering, from 11,530 to 127,756 reads per sample were used for matching against the taxonomic database. From 92.6 to 99.82% of those reads were successfully classified to genus level.
Alpha and beta biodiversity
Alpha biodiversity for each of the clinical materials and statistically significant differences were demonstrated (P < 0.05) (). The alpha biodiversity expressed by the Simpson index was not statistically significant only for the samples of saliva (P < 0.06). Beta biodiversity, expressed by the Bray-Curtis, Jensen-Shannon and Jaccard indices, was also statistically significant (P < 0.05) ().
Table 2. Alpha diversity (assessed by richness Chao1, Shannon and Simpson), and beta diversity indices (estimated by the Bray-Curtis, Jensen-Shannon and Jaccard indices) in the studied groups (group I vs. Group II vs. Group III).
Profiles with the percentage distribution of bacteria at the L2 level
At the L2 level, the obtained operational taxonomic units (OTUs) were assigned to a total of 14 phyla, comprising the so-called core microbiome for each biological material (saliva, tongue, supragingival and subgingival plaque) in groups I, II and III, which included Firmicutes, Bacteroidetes, Proteobacteria, Fusobacteria, Actinobact-eria and Saccharibacteria. The most numerous phyla were Firmicutes and Bacteroidetes (, Table S1).
Figure 1. Relative percentage distribution of bacteria from the mouth on COVID-19 infection and taking antimicrobials at the phylum level (L2) depending on clinical material: saliva (), tongue ridge (), subgingival plaque () and supragingival plaque (), respectively.
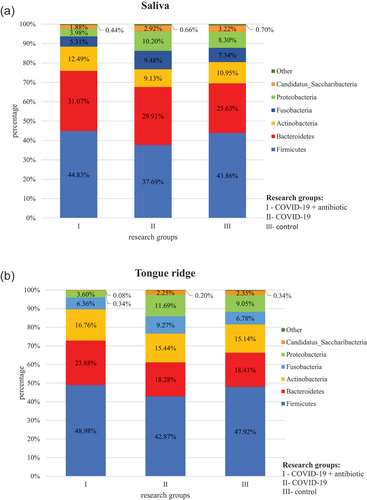
Comparison of results at the L2 level for the group of COVID-19 patients administered antibiotics (I) in relation to COVID-19 without antibiotics (II) and the control group (III)
In group I, as compared to group II, bacteria belonging to the following phyla were present in a significantly higher percentage: Firmicutes (P = 0.001 in saliva and P < 0.001 on the tongue, in subgingival plaque and supragingival plaque), Bacteroidetes (P = 0.018in saliva and P < 0.001 on the tongue, in subgingival plaque and supragingival plaque) and Actinobacteria (P = 0.035 in saliva, P = 0.001 on the tongue). On the other hand, in group I, as compared to group II, bacteria belonging to the following phyla were present in a significantly lower percentage: Proteobacteria (P = 0.036 in saliva) and Saccharibacteria (P = 0.009 on the tongue, P = 0.016 in subgingival plaque).
Profiles with the percentage distribution of bacteria at the L6 level
At the L6 level, the obtained OTUs were assigned to a total of 106 genera of bacteria. The core microbiome for all tested materials was bacteria belonging to the following genera: Streptococcus and Prevotella. Bacteria of the Prevotella genus were significantly more numerous in group I as compared to group II (P < 0.01 on the tongue and in subgingival plaque, P = 0.034 in supragingival plaque) and group III (P < 0.01 for all materials) as well as in group II as compared to group III (P < 0.01 in saliva and supragingival plaque) (, Table S2). In turn, bacteria of Streptococcus genus were present in a statistically significantly lower percentage in group I as compared to group III (P = 0.024 in saliva). In the case of other materials and study groups, no statistically significant differences were observed in relation to the above-mentioned genera. Detailed results of the differential analysis are included in the supplement (S3).
Figure 2. Relative percentage distribution of bacteria from the mouth on COVID-19 infection and taking antimicrobials at the genus level (L6) depending on clinical material: saliva (), tongue ridge (), subgingival plaque () and supragingival plaque (), respectively.
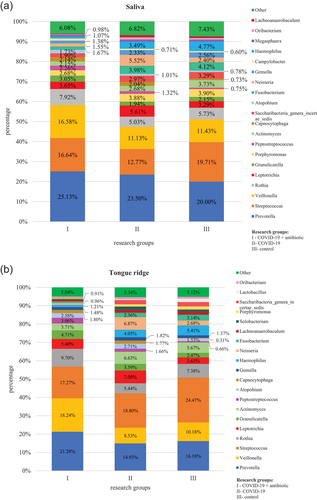
Comparison of results at the L6 level for the group of COVID-19 patients administered antibiotics (I) in relation to COVID-19 without antibiotics (II) and the control group (III)
In group I, as compared to group II, bacteria belonging to the following genera were present in a significantly higher percentage: Veillonella (P = 0.045 in saliva and P < 0.01 on the tongue, in subgingival plaque and in supragingival plaque), Fusobacterium (P = 0.021 on the tongue), Cardiobacterium (P = 0.011 in saliva, P < 0.01 on the tongue), Selenomonas (P = 0.038 in saliva), Rothia (P = 0.021 on the tongue), Tanerella (P = 0.011 in saliva) and Capnocytophaga (P < 0.01 in subgingival plaque and in supragingival plaque). On the other hand, in group I, as compared to group II, bacteria belonging to the Cardiobacterium (P = 0.036 in subgingival plaque) and Corynebacterium (P < 0.01 on the tongue) genera were present in a significantly lower percentage. Comparisons of results at the L6 level for the group of COVID-19 patients administered antibiotics (I) in relation to the control group (III) and for the group of COVID-19 patients without antibiotics (II) in relation to and the control group (III) are placed in and supplementary materials (S4, S5), respectively. Moreover, shows the increase in the share of bacteria in a given niche of the oral cavity that affects inflammatory processes.
Figure 3. Increase and decrease the number of studied bacteria at the phylum (L2) and genus (L6) level in COVID-19 convalescents who received antibiotics during hospitalization compared to the control (left side of figure) and COVID-19 infection without antimicrobial treatment compared to the control group (right side of the figure) taking antimicrobials depending on clinical material: saliva, tongue ridge, subgingival plaque, supragingival plaque, respectively.
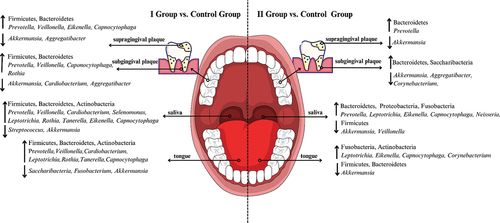
Correlations between the oral microbiota and clinical indices
The choice of the analysed types of bacteria at the L6 level was made on the basis of a literature study [Citation23–26] and then correlated with selected dental indices (DMFT, D, PI). The Spearman’s correlation was used in the analysis.
In group I, statistically significant, strong positive correlations were observed between the D number and bacteria of the Fusobacterium and Leptotrichia genera (P = 0.011 and P = 0.034 for saliva materials, respectively) and between the PI index and bacteria of the Selenomonas genus (P = 0.018, for subgingival plaque materials). Other statistically significant, strong correlations were negative and occurred between the D number and the Cardiobacterium genus (P = 0.030 for supragingival plaque materials), PI index and Eikenella genus (P = 0.020 for dorsal material) and between the DMFT index and Corynebacterium and Fusobacterium genera (P = 0.005 and P = 0.029 for subgingival and supragingival plaque materials, respectively). In all tested clinical materials, strong negative correlations between the DMFT index and bacteria of the Leptotrichia genus were found ().
Table 3. Correlations between selected dental indices and types of bacteria in the examined clinical materials for the groups of patients included in the study.
In group II, statistically significant, strong positive correlations occurred only between the D number and bacteria of the Prevotella genus (P = 0.002 for supragingival plaque materials). In turn, statistically significant strong negative correlations were observed between the D number and the Cardiobacterium genus (P = 0.012 for dorsal materials) as well as the DMFT index and the Veillonella genus (P = 0.002 for subgingival plaque materials).
In group III, statistically significant, strong positive correlations were observed between the D number and bacteria of the Eikenella and Tannerella genera (P = 0.017 and P = 0.019 for saliva materials, respectively) as well as between the DMFT index and bacteria of the Akkermansia genus (P = 0.011, for subgingival plaque materials). In turn, statistically significant strong negative correlations occurred between the D number and the Rothia genus (P = 0.029 for dorsal materials), the PI index and the Corynebacterium genus (P = 0.009 for saliva materials) as well as the DMFT index and bacteria of the following genera: Aggregatibacter (P = 0.027 for dorsal materials), Corynebacterium i Fusobacterium (P = 0.017 and P = 0.023 for saliva and dorsal materials, respectively) (). Other correlations, although statistically significant, showed low strength and are presented in .
Discussion
The present study focused on a comprehensive comparison of the oral microbiota composition and its correlation with selected dental indices in selected groups of patients after hospitalisation for COVID-19. To the best knowledge of the authors, this is currently the first study to analyze and correlate these parameters. In the present study, based on selected dental indices, we noticed that the oral hygiene in COVID-19 patients was neglected. PI index was statistically significantly higher in the I and II group, as compared to the control group (). It is consistent with the results of Anand PS et al. who examined periodontal conditions and hygiene in patients with COVID-19, proving that they had significantly higher average values of dental plaque, which is the main factor exacerbating periodontal disease [Citation12].
According to the WHO, as early as before the SARS-CoV-2 pandemic, severe periodontal diseases affected approximately 19% of the world adult population, which corresponds to over 1 billion cases worldwide [Citation27]. Anand PS et al. examined periodontal condition and hygiene in patients with COVID-19, proving that they had significantly higher average values of dental plaque, which is the main factor exacerbating periodontal disease [Citation12].
Poor oral hygiene may contribute to the exacerbation of COVID-19 symptoms, as the aspiration of periodontal disease-causing bacteria induces the expression of angiotensin-converting enzyme 2, a receptor for SARS-CoV-2, and the production of inflammatory cytokines in the lower respiratory tract [Citation28]. In turn, improving oral health may reduce the risk of COVID-19 complications [Citation29]. For this reason, the condition of the oral cavity, which is the main portal of entry for infection [Citation30], deserves special attention.
In the group of COVID-19 patients receiving antibiotics an increase in the population of genera associated with poor oral hygiene and periodontitis, such as Prevotella, Capnocytophaga, Eikenella, Tanerella and Selenomonas was observed. Additionally, a significantly higher percentage was represented by bacteria from the Firmicutes phylum. This may possibly be related to the deterioration of oral health.
The core microbiome, which included the Streptococcus, Prevotella and Veillonella genera, was distinguished for all study groups and in all tested materials. Streptococcus bacteria were presented in each group of patients in a similar percentage, which indicates that Streptococcus bacteria are one of the most stable elements of the oral microbiota [Citation31].
The genera Prevotella and Veillonella were present in a significantly higher percentage in group I than in the remaining groups in all the study materials. The Prevotella genus demonstrated a strong positive correlation with the number of carious lesions in the supragingival plaque in group II. Yang F et al. observed an increase in the population of the Prevotella genus in people with active caries, which is consistent with the relationship observed in our study [Citation32].
Bacteria of the Prevotella and Veillonella genera are capable of producing lipopolysaccharides and inducing inflammatory responses [Citation33]. Species of Veillonella genus reveal a strong ability to induce interleukin-6 (IL-6) [Citation34], while Prevotella primarily activates the TLR-2 receptor and enhances the expression of inflammatory cytokines, including IL-23 and IL-1 [Citation35]. According to the results presented by Khan AA et al., the proteins of the species of the Prevotella genus, and not the viral proteins, are involved in many interactions with NF-kB, which increases the clinical severity of COVID-2019 [Citation35], proving that the Prevotella genus plays an important role in the progression of COVID-19. In other studies, it was observed that bacteria of the Prevotella genus are present in a higher percentage in COVID-19 patients with prolonged symptoms of infection [Citation33].
In turn, in COVID-19 convalescent patients who were subjected to antibiotic therapy, the balance between the inflammatory microbiota and the inflammation-reducing microbiota is slowly restored [Citation23,Citation36]. Studies by Cui GY et al. indicate that the oral microbiota of one-year convalescents, determined on the basis of swabs collected from the tongue, was largely restored, but did not completely return to normal.
In the regeneration process, the population of microorganisms producing butyric acid gradually increased, while the number of microorganisms producing lipopolysaccharides gradually decreased [Citation37]. The results of our study confirm this phenomenon. A statistically significant decrease in bacteria of the Fusobacterium genus (producing butyric acid) in group I as compared to group III in the material from the tongue was observed, while the bacterial count of the Leptotrichia and Selenomonas genera (producing lipopolysaccharides – LPS) was significantly higher in both groups of patients with COVID-19, as compared to healthy individuals. Similar results were described in the work of Ren Z et al. in which a decrease in the population of bacteria of the Porphyromonas and Fusobacterium genera and an increase in the population of bacteria of the Leptotrichia and Selenomonas genera was recorded [Citation23].
Butyric acid reduces inflammation as well as oxidative stress under various pathological conditions, including viral respiratory infections. Therefore, the reduction of butyric acid-producing bacteria may lead to increased inflammation and, consequently, to an increased risk of developing pneumonia [Citation38]. In turn, lipopolysaccharides stimulate lymphocytes, fibroblasts and macrophages to produce a wide range of antibodies with different specificities, a number of cytokines and other effector molecules with inflammatory, immunomodulatory and tissue-destroying properties, which leads to an exacerbation of the inflammatory response [Citation25].
Taking under consideration poor oral health of COVID-19 patients receiving antibiotics, a strong positive correlation between Fusobacterium producing butyric acid in saliva and carious lesions is worth noting. It is consistent with the results of Yang F. et al., who pointed out positive correlation between active caries and concentration of Fusobacterium [Citation32].
A positive correlation of the Leptotrichia genus producing lipopolysaccharides in saliva with the number of carious foci (D number) and a strong positive correlation of bacteria of the Selenomonas genus with the PI index were observed. The Selenomonas genus includes pathogens that inhabit the gingival sulcus and periodontal pocket, and the Leptotrichia genus ferment carbohydrates and produce lactic acid, similarly to S. mutans [Citation39]. This suggests their involvement in the formation of caries.
Bacteria of the Capnocytophaga and Eikenella genera are characterised by a high destructive potential in relation to the periodontium and a potential effect on the exacerbation of periodontitis in adults [Citation26]. In this study, the Eikenella genus was present in a significantly higher percentage in group I (saliva, supragingival plaque) and in group II, as compared to group III (saliva, tongue). Scientific reports indicate participation of Eikenella in deltoid muscle abscess, pericarditis, brain abscess, perirenal abscess, head and neck infections, liver abscess, and hip arthritis [Citation40].
Capnocytophaga genus in the subgingival and supragingival plaque material was significantly more than doubled in group I (8–10%), as compared to groups II and III (3–4%). In another study, bacteria of the Capnocytophaga genus were detected in the bronchoalveolar lavage fluid of COVID-19 patients [Citation41]. This is confirmed by the fact that the oral cavity may be a source of pathogens infecting the lungs [Citation41] and causing secondary bacterial infections in the course of SARS-CoV-2 infection [Citation42].
It is also worth paying attention to the Akkermansia genus. In our study, a statistically significant decrease in the amount of these bacteria in both groups with COVID-19, as compared to the group of healthy people, was observed for all materials. Bacteria of the Akkermansia genus are well characterised in the context of the intestinal microbiota, but their role in the oral cavity is still not well understood [Citation43]. It is likely that Akkermansia genus in the oral cavity may reduce the production of inflammatory cytokines as well as other inflammatory biomarkers associated with periodontitis caused by periodontal pathogens [Citation44].
In the present study, bacteria of the Cardiobacterium genus which have been so far poorly understood in COVID-19 patients showed a strong negative correlation with the number of carious lesions. Therefore, results different from those of the team of Espinoza JL et al., who analysed the interactions of microorganisms present in the supragingival plaque with other organisms and metabolic pathways in the oral cavity, were obtained [Citation45]. The team observed the coexistence of Cardiobacterium with other oral bacteria and the impact of this genus on their metabolism, which favoured the development of caries [Citation45].
Based on the presented results, it has been observed that the group of patients treated with antibiotics is distinguished by a different percentage distribution of individual taxa as compared to the group without antibiotics and the control group, which are similar to each other. This indicates that infection with the SARS-CoV-2 virus itself does not significantly change the oral microbiota. Other researchers observed that the oral microbiota of COVID-19 patients treated with antibiotics showed less diversity compared to healthy individuals [Citation37,Citation46,Citation47]. This might suggest that treatment with ceftriaxone, which is in vitro activity against Gram-positive and Gram-negative aerobic and some anaerobic bacteria, probably might contribute to changing the biodiversity of the microbiota, promoting an increase in the number of anaerobic and relatively anaerobic bacteria participating in inflammatory processes inside the oral cavity. Therefore, it should be emphasised that the introduction of antibiotic therapy in the course of viral diseases, including COVID-19, should be considered individually and used only in a situation justified by the patient’s clinical condition. On average, 75% of COVID-19 cases worldwide were treated with antibiotics, while only 18% of patients who received antibiotics had secondary bacterial infections. On average, approximately half of the COVID-19 patients who received antibiotics were neither seriously nor critically ill [Citation48]. In this context, it should be emphasised that as early as before the SARS-CoV-2 pandemic, the excessive use of antibiotics, inducing the phenomenon of resistance, was mentioned as the main priority of global health care [Citation49].
Our study has numerous strengths; nevertheless, we should also acknowledge several limitations. Firstly, the inability to collect clinical materials from patients before the start of antibiotic therapy makes it difficult to unambiguously assess the real impact of antibiotics on the oral microbiota. This limitation may interfere with the test results. However, we found statistically significant differences between the study groups. Further studies are needed to compare the composition of the oral microbiome before and after antibiotic therapy. The second limitation is the lack of microbiota analysis depending on the severity of COVID-19 in the study. Another limitation of the study is that the analysis was carried out at the genus level, without taking into account the species. At the genus level, the correspondence of the tested sequences with the sequences deposited in databases is in the range of 95–97%, which enables obtaining the most reliable information. In turn, at the species level, these percentages are much lower and there is a high probability of drawing incorrect conclusions as to the statistical significance of a given bacterial species. These limitations result directly from the sequencing of short (about 550 bp) fragments. In future research, it would be advisable to use sequencing of longer fragments, which, unfortunately, is associated with much higher research costs.
Conclusions
It has been found that the main factor interfering the composition of the oral microbiota was not the course of the SARS-CoV-2 infection itself, but primarily the use of antibiotic therapy.
In the group of COVID-19 patients treated with antibiotics, the qualitative depletion of the microbiota increased LPS-producing bacteria, and a reduced number of butyric acid-producing bacteria were found.
A significant reduction in the number of the Akkermansia genus in both convalescents with COVID-19 probably may have a negative impact on the reduction of inflammatory cytokines and other inflammatory biomarkers associated with periodontitis caused by periodontal pathogens. Worse oral health in the group of patients with COVID-19 treated with antibiotics and the observed increased number of pro-inflammatory types of bacteria in the oral cavity may affect the course of COVID-19.
Author’s contribution
Conceptualization: JZ, MBW; Collecting material: BBS, JZ, EZF, WO, JK; Statistical analysis and methodology: BBS, KTĆ, ASO, AG; Data interpretation: BBS, KTĆ, ASO, MBW; Writing – review and editing: BBS, KTĆ, ASO; Supervision: JZ, MBW.
Supplemental Material
Download Zip (609.9 KB)Disclosure statement
No potential conflict of interest was reported by the author(s).
Supplementary material
Supplemental data for this article can be accessed online at https://doi.org/10.1080/20002297.2023.2264591.
Additional information
Funding
References
- Dewhirst FE, Chen T, Izard J, et al. The human oral microbiome. J Bacteriol. 2010;192(19):5002–13. doi: 10.1128/JB.00542-10
- Caselli E, Fabbri C, D’Accolti M, et al. Defining the oral microbiome by whole-genome sequencing and resistome analysis: the complexity of the healthy picture. BMC Microbiol. 2020;20(1):120. doi: 10.1186/s12866-020-01801-y
- Mosaddad SA, Tahmasebi E, Yazdanian A, et al. Oral microbial biofilms: an update. Eur J Clin Microbiol Infect Dis. 2019;38(11):2005–2019. doi: 10.1007/s10096-019-03641-9
- Kowalska-Duplaga K, Kapusta P, Gosiewski T. Changes in the Intestinal Microbiota Are Seen Following Treatment with Infliximab in Children with Crohn's Disease. J Clin Med. 2020;9:687. doi: 10.3390/jcm9030687
- Salamon D, Sroka-Oleksiak A, Gurgul A, et al. Analysis of the gut mycobiome in adult patients with type 1 and type 2 diabetes using next-generation sequencing (NGS) with increased sensitivity—Pilot study. Nutrients. 2021;13(4):1066. doi: 10.3390/nu13041066
- Chen X, Kang Y, Luo J, et al. Next-generation sequencing reveals the progression of COVID-19. Front Cell Infect Microbiol. 2021;11:632490. doi: 10.3389/fcimb.2021.632490
- Ferrer M, Méndez-García C, Rojo D, et al. Antibiotic use and microbiome function. Biochem Pharmacol. 2017;134:114–126. doi: 10.1016/j.bcp.2016.09.007
- Patangia DV, Anthony Ryan C, Dempsey E, et al. Impact of antibiotics on the human microbiome and consequences for host health. Microbiologyopen. 2022;11(1):1260. doi: 10.1002/mbo3.1260
- Konstantinidis T, Tsigalou C, Karvelas A, et al. Effects of antibiotics upon the gut microbiome: a review of the literature. Biomedicines. 2020;8(11):502. doi: 10.3390/biomedicines8110502
- Ready D, Lancaster H, Qureshi F, et al. Effect of amoxicillin use on oral microbiota in young children. Antimicrob Agents Chemother. 2004;48(8):2883–2887. doi: 10.1128/AAC.48.8.2883-2887.2004
- Ladirat SE, Schols HA, Nauta A, et al. High-throughput analysis of the impact of antibiotics on the human intestinal microbiota composition. J Microbiol Methods. 2013;92(3):387–397. doi: 10.1016/j.mimet.2012.12.011
- Anand PS, Jadhav P, Kamath KP, et al. A case-control study on the association between periodontitis and coronavirus disease (COVID-19). J Periodontol. 2022;93(4):584–590. doi: 10.1002/JPER.21-0272
- Therapeutics and COVID-19: Living guideline. [Internet]. World Health Organization; 2023 [cited 2023 January 13]. Available from: https://www.who.int/publications/i/item/WHO-2019-nCoV-therapeutics-2022.5
- Murererehe J, Malele-Kolisa Y, Niragire F, et al. Prevalence of dental caries and associated risk factors among people living with HIV/AIDS and HIV uninfected adults at an HIV clinic in Kigali, Rwanda. PLoS One. 2023;18(4):0276245. doi: 10.1371/journal.pone.0276245
- O’Leary TJ, Drake RB, Naylor JE. The plaque control record. J Periodontol. 1972;43(1):38. doi: 10.1902/jop.1972.43.1.38
- Talaga-Ćwiertnia K, Sroka-Oleksiak A, Zapała B, et al. New insights into diversity of the upper respiratory tract microbiota and its relationship with SARS-CoV-2 viral load in the nasopharyngeal epithelial cells in patients with COVID-19. Pol Arch Intern Med. 2023; 133:16442.
- Wang Q, Garrity GM, Tiedje JM, et al. Naive bayesian classifier for rapid assignment of rRNA sequences into the New bacterial taxonomy. Appl Environ Microbiol. 2007;73(16):5261–5267. doi: 10.1128/AEM.00062-07
- Taxonomic reference data [Internet]. DADA2 documentation. 2021. Available from: https://benjjneb.github.io/dada2/training.html
- Chong J, Liu P, Zhou G, et al. Using MicrobiomeAnalyst for comprehensive statistical, functional, and meta-analysis of microbiome data. Nat Protoc. 2020;15(3):799–821. doi: 10.1038/s41596-019-0264-1
- Robinson MD, McCarthy DJ, Smyth GK. edgeR: a Bioconductor package for differential expression analysis of digital gene expression data. Bioinformatics. 2010;26:139–140. doi: 10.1093/bioinformatics/btp616
- Benjamini Y, Hochberg Y. Controlling the false discovery rate: a practical and powerful approach to multiple testing. J Royal Stat Soc Ser B (Methodological). 1995;57(1):289–300. doi: 10.1111/j.2517-6161.1995.tb02031.x
- Mattiello F, Verbist B, Faust K, et al. A web application for sample size and power calculation in case-control microbiome studies. Bioinformatics. 2016;32(13):2038–2040. doi: 10.1093/bioinformatics/btw099
- Ren Z, Wang H, Cui G, et al. Alterations in the human oral and gut microbiomes and lipidomics in COVID-19. Gut. 2021;70(7):1253–1265. doi: 10.1136/gutjnl-2020-323826
- Khaledi M, Sameni F, Afkhami H, et al. Infective endocarditis by HACEK: a review. J Cardiothorac Surg. 2022;17(1):185. doi: 10.1186/s13019-022-01932-5
- Wilson M. Biological activities of lipopolysaccharides from oral bacteria and their relevance to the pathogenesis of chronic periodontitis. Sci Prog. 1995;78(Pt 1):19–34.
- Socransky SS, Haffajee AD, Cugini MA, et al. Microbial complexes in subgingival plaque. J Clin Periodontol. 1998;25(2):134–144. doi: 10.1111/j.1600-051X.1998.tb02419.x
- Global oral health status report: towards universal health coverage for oral health by 2030 [Internet]. World Health Organization. 2022. Available from: https://www.who.int/publications/i/item/9789240061484
- Takahashi Y, Watanabe N, Kamio N, et al. Aspiration of periodontopathic bacteria due to poor oral hygiene potentially contributes to the aggravation of COVID-19. J Oral Sci. 2020;63(1):1–3. doi: 10.2334/josnusd.20-0388
- Sampson V, Kamona N, Sampson A. Could there be a link between oral hygiene and the severity of SARS-CoV-2 infections? Br Dent J. 2020;228(12):971–975. doi: 10.1038/s41415-020-1747-8
- Nitin P, Nandhakumar R, Vidhya B, et al. COVID-19: invasion, pathogenesis and possible cure - a review. J Virol Methods. 2022;300:114434. doi: 10.1016/j.jviromet.2021.114434
- Lenartova M, Tesinska B, Janatova T, et al. The oral microbiome in periodontal health. Front Cell Infect Microbiol. 2021;11:629723. doi: 10.3389/fcimb.2021.629723
- Yang F, Zeng X, Ning K, et al. Saliva microbiomes distinguish caries-active from healthy human populations. Isme J. 2012;6(1):1–10. doi: 10.1038/ismej.2011.71
- Haran JP, Bradley E, Zeamer AL, et al. Inflammation-type dysbiosis of the oral microbiome associates with the duration of COVID-19 symptoms and long COVID. JCI Insight. 2021;6(20):152346. doi: 10.1172/jci.insight.152346
- Segal LN, Clemente JC, Tsay JC, et al. Enrichment of the lung microbiome with oral taxa is associated with lung inflammation of a Th17 phenotype. Nat Microbiol. 2016;1(5):16031. doi: 10.1038/nmicrobiol.2016.31
- Khan AA, Khan Z, Elofsson A. COVID-2019-associated overexpressed Prevotella proteins mediated host–pathogen interactions and their role in coronavirus outbreak. Bioinformatics. 2020;36(13):4065–4069. doi: 10.1093/bioinformatics/btaa285
- Gao M, Wang H, Luo H, et al. Characterization of the human oropharyngeal microbiomes in SARS-CoV-2 infection and recovery patients. Adv Sci. 2021;8(20):2102785. doi: 10.1002/advs.202102785
- Cui GY, Rao BC, Zeng ZH, et al. Characterization of oral and gut microbiome and plasma metabolomics in COVID-19 patients after 1-year follow-up. Mil Med Res. 2022;9(1):32. doi: 10.1186/s40779-022-00387-y
- NK K, Patil P, Bhandary SK, et al. Is butyrate a natural alternative to dexamethasone in the management of CoVID-19? F1000Res. 2021;10:273. doi: 10.12688/f1000research.51786.1
- Eribe ERK, Olsen I. Leptotrichia species in human infections II. J Oral Microbiol. 2017;9(1):1368848. doi: 10.1080/20002297.2017.1368848
- Li L, Shi YB, Weng XB. Eikenella corrodens infections in human: reports of six cases and review of literatures. J Clin Lab Anal. 2022;36(2):24230. doi: 10.1002/jcla.24230
- Chen L, Liu W, Zhang Q, et al. RNA based mNGS approach identifies a novel human coronavirus from two individual pneumonia cases in 2019 Wuhan outbreak. Emerg Microbes Infect. 2020;9(1):313–319. doi: 10.1080/22221751.2020.1725399
- Zhou F, Yu T, Du R, et al. Clinical course and risk factors for mortality of adult inpatients with COVID-19 in Wuhan, China: a retrospective cohort study. Lancet. 2020;395(10229):1054–1062. doi: 10.1016/S0140-6736(20)30566-3
- Karamzin AM, Ropot AV, Sergeyev OV, et al. Akkermansia muciniphila and host interaction within the intestinal tract. Anaerobe. 2021;72:102472. doi: 10.1016/j.anaerobe.2021.102472
- Huck O, Mulhall H, Rubin G, et al. Akkermansia muciniphila reduces Porphyromonas gingivalis-induced inflammation and periodontal bone destruction. J Clin Periodontol. 2020;47(2):202–212. doi: 10.1111/jcpe.13214
- Espinoza JL, Torralba M, Leong P, et al. Differential network analysis of oral microbiome metatranscriptomes identifies community scale metabolic restructuring in dental caries. PNAS Nexus. 2022;1(5):239. doi: 10.1093/pnasnexus/pgac239
- Wu Y, Cheng X, Jiang G, et al. Altered oral and gut microbiota and its association with SARS-CoV-2 viral load in COVID-19 patients during hospitalization. NPJ Biofilms Microbiomes. 2021;7(1):61. doi: 10.1038/s41522-021-00232-5
- Rafiqul Islam SM, Foysal MJ, Hoque MN, et al. Dysbiosis of oral and gut microbiomes in SARS-CoV-2 infected patients in Bangladesh: elucidating the role of opportunistic gut microbes. Front Med. 2022;9:821777. doi: 10.3389/fmed.2022.821777
- Chedid M, Waked R, Haddad E, et al. Antibiotics in treatment of COVID-19 complications: a review of frequency, indications, and efficacy. J Infect Public Health. 2021;14(5):570–576. doi: 10.1016/j.jiph.2021.02.001
- Gow NAR, Johnson C, Berman J, et al. The importance of antimicrobial resistance in medical mycology. Nat Commun. 2022;13(1):5352. doi: 10.1038/s41467-022-32249-5