ABSTRACT
Aim
To determine the antimicrobial activity of the bacteriocin-producing probiotic strains Streptococcus salivarius K12 and Streptococcus salivarius M18 alone or in combination against caries-associated Streptococcus mutans.
Methods
Antimicrobial activity of S. salivarius K12 and/or S. salivarius M18 against S. mutans ATCC 25175 growth and biofilm formation on hydroxyapatite (HA) discs was determined in a flow chamber model by recording the colony forming units (CFU/ml) after 48 h of co-cultivation. The biofilm was analyzed by scanning electron microscopy (SEM) and by confocal laser scanning microscopy (CLSM). Additionally, the simultaneous antagonism assay was used to assess the inhibitory effect of S. salivarius K12 and/or S. salivarius M18 against S. mutans ATCC 25175 and 21 clinical isolates of S. mutans.
Results
Co-cultivation of S. mutans and S. salivarius K12 and/or S. salivarius M18 led to the inhibition of S. mutans viability, thereby, preventing its biofilm formation on HA discs. Furthermore, S. salivarius K12 and S. salivarius M18 exhibited antimicrobial activity against most clinical isolates of S. mutans.
Conclusion
The in vitro flow chamber system used in this study allows the simulation of time-dependent administration of S. salivarius probiotic strains, either alone or in combination, to investigate the prevention of S. mutans biofilm formation in a standardized model.
Introduction
The oral cavity is inhabited by complex microbial communities that can form biofilms which may subsequently lead to caries and periodontitis [Citation1]. Caries remains a major public health problem and is characterized by a multifactorial etiology. Under favorable conditions, the cariogenic oral microbiota in the biofilm metabolize dietary carbohydrates resulting in prolonged periods of local demineralization of the enamel, i.e. white spot lesions or cavitated caries of the dentin [Citation2]. Various models exist to explain the development of cariogenic biofilms as well as important influencing factors such as genetic predisposition and the individual composition of the oral microbiome [Citation2]. The formation of a cariogenic biofilm is described by a shift from dynamic stability with early colonizers to a dysbiotic state characterized by a multispecies cariogenic community including mutans streptococci, Lactobacillus species, bifidobacteria and opportunistic Actinomyces species [Citation2].
Orthodontic fixed appliances promote the accumulation of plaque around brackets and wires. Particularly, predilection sites with an increased risk for gingivitis, demineralization of the enamel, and the proliferation of pathogenic species i.e. S. mutans are found between brackets and gingiva [Citation3–5]. Specialized orthodontic brushes, and pharmaceutical products such as antimicrobial mouth rinses and toothpastes appears to be not sufficient to prevent the buildup of pathogenic biofilms [Citation6,Citation7]. Therefore, additional therapeutic measures might be necessary to maintain a good oral hygiene during orthodontic treatment.
One promising treatment strategy includes the use of beneficial bacteria. S salivarius K12 and S. salivarius M18 are commercially produced probiotics available as single strain lozenges or as strain mixtures. Both strains are producing bacteriocin-like inhibitory substances (BLIS), called salivaricins [Citation8,Citation9]. Salivaricins belong to the large group of lantibiotics, ribosomally synthesized antimicrobial peptides containing the amino acid lanthionine, which show an antibiotic effect against specific bacterial strains [Citation10,Citation11]. However, there is no consensus on how salivaricins affect dental health in detail [Citation12]. Moreover, although a product containing both probiotic strains is commercially available, the antimicrobial effect of S. salivarius strains combination has not yet been investigated in detail [Citation13]. The goal of this study is to determine the antibacterial effect of S. salivarius K12 and S. salivarius M18 alone or in combination on S. mutans biofilm development in a dynamic model mimicking real-life clinical biofilm formation on hydroxyapatite discs and metal brackets. The hypothesis of the present study is that the single administration of S. salivarius K12 and/or S. salivarius M18 inhibits S. mutans under these conditions.
Materials and methods
Bacterial strains and growth conditions
S. salivarius K12 (Burgerstein Biotics-O, Fa. Burgerstein, Switzerland) and S. salivarius M18 Throat Guard® (BLIS Technologies Limited, Wellington, New Zealand) from lozenges were suspended in 3 ml THB (Todd Hewitt Broth, BD, Allschwil, Switzerland) for 1.5 h under aerobic conditions at 37°C. S. mutans ATCC 25175, 21 clinical S. mutans isolates, Bacteroides fragilis ATCC 25285, Micrococcus luteus ATCC 6575, M. luteus ATCC 6592 and S. salivarius MU (a non salivaricin-producer, kindly provided by Prof. J. Tagg, Department of Microbiology and Immunology, University of Otago, Dunedin, New Zealand) were grown on Columbia blood agar plates (BBLTM, Becton Dickinson, Allschwil, Switzerland) supplemented with 0.1% calcium carbonate (CaCO3) and 5% human blood (Blood donor center, Basel, Switzerland) under anaerobic conditions (80% N2, 10% H2 and 10% CO2) at 37°C for either 24 h or 48 h.
Anaerobic flow chamber model
The in vitro flow chamber model used in this study has been previously described in detail [Citation14]. Briefly, a flow chamber was connected to the bacterial culture dispenser and the peristaltic pump by polyvinylchloride tubes. Sterile hydroxyapatite discs with a diameter of 12.1 mm and a thickness of 2.1 to 2.4 mm (ENAMEL LIKE HA discs, Fa. Himed N.Y.) as substrate for biofilm formation were placed in the flow chamber together with artificial saliva (AS) to simulate human oral conditions [Citation15]. The flow rate was set at 0.8 ml/min to simulate physiological low shear conditions found in oral cavity. The system was incubated under anaerobic conditions (80% N2, 10% H2 and 10% CO2) at 37°C for 48 h on a shaker (260 rev/min) [Citation14]. To mimic the different surfaces (teeth and metal) present during orthodontic treatment, lower incisor brackets (Victory, 3 M Unitek Monrovia, CA) were fixed with dental composite (GC Ortho Connect™, Fa. GC, Tokyo, JPN) on hydroxyapatite discs. Discs without fixed appliances served as control.
A co-cultivation model in the anaerobic flow chamber was implemented to assess the antimicrobial activity of S. salivarius K12 and/or S. salivarius M18 on S. mutans in a biofilm (). For this, 10 single colonies of S. mutans grown on Columbia blood agar plates (CBA) were transferred to 30 ml THB containing 0.5% sucrose. After 24 h of anaerobic incubation at 37°C, 3 ml of suspension was diluted in 27 ml of 0.9% NaCl. S. salivarius K12 resp. S. salivarius M18 were extracted from lozenges as described above and 100 µl of the respective suspensions were plated on CBA and incubated anaerobically at 37°C. After 24 h, single colonies of either S. salivarius K12 or S. salivarius M18 were resuspended in 30 ml 0.9% NaCl, ultrasonicated for 30 s (22.5 W, Vibracell, Sonics & Materials, Newtown, CT) and centrifuged at 9200 rpm for 5 min at room temperature. The bacterial pellets were resuspended in 30 ml AS containing 0.5% sucrose and incubated in the flow chamber with one direction circulation mimicking oral saliva flow. Free-floating bacteria present in the tubing and dispenser represented the planktonic population, while bacteria attached to the HA discs were considered as biofilm-associated.
Figure 1. Experimental scheme of the anaerobic incubation of (a) S. mutans ATCC 25175 (control) and (b) S. mutans and S. salivarius co-cultivation in a flow chamber. S. mutans ATCC 25175 and S. salivarius K12/S. salivarius M18 were suspended in 30 ml AS containing 0.5% sucrose (T0). After 24 h (T1), the medium was replaced with 30 ml AS containing 0.5% sucrose (a) or 30 ml AS containing 0.5% sucrose and the probiotic bacteria S. salivarius K12 and/or S. salivarius M18 (b). Bacteria were incubated for additional 24 h (T2). Planktonic bacteria were quantified at the beginning of the experiment (T0) and after 48 h of incubation (T2). The biofilm formed on the HA discs after 48 h (T2) was analyzed by determining the CFU/ml, SEM, and CLSM.
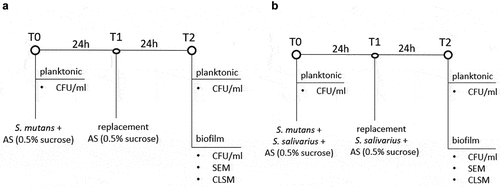
For the co-cultivation, S. mutans ATCC 25175 was mixed with a single strain of S. salivarius K12 or S. salivarius M18 or with S. salivarius K12 and S. salivarius M18 combination in 1:1 or 1:1:1 ratio, respectively (T0). The biofilms were then allowed to form on the HA discs by placing them for 48 h in the flow chamber. The incubation time of 48 h was chosen to enable one medium exchange and re-administration of the probiotic strains. Therefore, after 24 h (T1), the medium was replaced with 30 ml AS supplemented with 0.5% sucrose and S. salivarius K12 and/or S. salivarius M18. After additional incubation for 24 h, the flow chamber system was stopped and the planktonic and the biofilm-associated bacteria were analyzed (T2). A flow chamber system with S. mutans only was used as a positive control ().
Quantification of bacteria
To quantify the number of viable bacteria present in the biofilm formed on the HA discs, the discs were removed from the flow chamber system, dipped gently in 0.9% NaCl to remove any non-adherent cells and vortexed in 5 ml 0.9% NaCl for 1 min. For each suspension, serial dilutions were made in 0.9% NaCl and 100 µl of appropriate dilutions were plated on Mitis Salivarius Agar (Difco™ Mitis Salivarius Agar, BD, Allschwil, Switzerland), a medium used for the selective isolation of Streptococcal species [Citation16]. To determine the number of viable bacteria in the planktonic suspensions, serial dilutions were made in 0.9% NaCl and 100 µl of appropriate dilutions were plated on Mitis Salivarius Agar.
Planktonic and biofilm-associated bacteria were quantified at the start of the experiment (T0) and after 48 h of incubation (T2) ().
Fluorescence in situ hybridization (FISH) and CLSM
High-pressure liquid chromatography (HPLC)-purified oligonucleotide probes for Streptococcus spp. and S. mutans were commercially synthesized and 5’-end modified with Cy3 and Cy5 fluorophores, respectively (Microsynth, Balgach, Switzerland) (). Appropriate probe sequences for the specific detection of each bacterial strain in the biofilm have been described previously [Citation17]. Three biofilm-coated discs with S. salivarius K12 resp. S. salivarius M18 and S. mutans ATCC 25175 biofilms were fixed in 4% paraformaldehyde in physiological saline (NaCl 0.9%) for 1 h at 4°C and washed twice with 0.9% NaCl with RNase inhibitor (Guard® RNase Inhibitor, Sigma-Aldrich, Switzerland). Thereafter, the samples were permeabilized by exposure to 7 mg/ml of lysozyme (Fluka, Buchs, Switzerland) in lysis buffer (0.1 M Tris-HCl [pH 7.5], 5 mM EDTA) for 10 min at 37°C in a humid chamber, and rinsed with physiological saline with RNase inhibitor. Non-specific hybridization was blocked with Denhardt’s solution for 1 h at 37°C. For hybridization, HA discs were placed in 96-well polystyrene plates and incubated in hybridization buffer (0.9 M NaCl, 20 mM Tris-HCl [pH 7.5], 25% (vol/vol) formamide, and 0.01% (wt/vol) sodium dodecyl sulfate) containing streptococci oligonucleotide probes (10 ng/ul) at 46°C for 3 h. After hybridization, the biofilms were immediately transferred into washing buffer (102 mM NaCl, 20 mM Tris-HCl [pH 7.5], 5 mM EDTA, 0.01% SDS) at 48°C for 30 min. Biofilms were examined using a Leica SP8® microscope (Leica, Wetzlar, Germany). The images were collected using 520–600 nm and 650–730 nm emission spectrum for Cy3 and Cy5, respectively. Confocal images were obtained using a ×63 oil immersion objective. Each biofilm was scanned at three arbitrary positions at the center of the disc. Z-direction series were generated by vertical optical sectioning at every position with the thickness of the slices set to 0.46 µm.
Table 1. Characteristics of 16S rRNA gene-directed oligonucleotide probes used for FISH.
Scanning electron microscopy (SEM)
Biofilm-coated HA discs with or without brackets were used for SEM. Bacteria were fixed overnight in 2% glutaraldehyde solution (Sigma, Buchs, Switzerland), washed once with phosphate buffered saline (PBS, ThermoFisher, Waltham, MA), and dehydrated in stepwise increasing concentrations of ethanol: 30%, 50%, 70%, 90% and 100% for 10 min each. The samples were then critical-point-dried and coated with 10 nm of gold and examined by scanning electron microscopy (Fei Nova NanoSEM 230®, Eindhoven, the Netherlands) [Citation18].
Antimicrobial activity of S. salivarius K12 and/or S. salivarius M18 - simultaneous antagonism method
Antimicrobial activity of S. salivarius K12 and S. salivarius M18 was determined using the simultaneous antagonism method as previously described [Citation9]. Briefly, overnight cultures of the respective indicator strains were prepared in 3 ml Todd-Hewitt Broth (THB; BD, Allschwil, Switzerland), adjusted to a turbidity equivalent of a McFarland 0.5 standard and 100 µl of the respective suspensions were plated on CaCO3 (CBA) plates. Single colonies of S. salivarius K12 and/or S. salivarius M18, respectively, were picked and stabbed successively into each agar plate. The plates were subsequently incubated at 37°C for 48 h under anaerobic conditions. After incubation, the zones of inhibition surrounding the S. salivarius colonies were measured: No inhibition 0 mm (−); Slight inhibition 1–2.5 mm (+); Inhibition 2.5–4 mm (++); Strong inhibition 4–11.0 mm (+++).
Data analysis and statistics
All experiments were performed at least in biological triplicates. Data were analyzed using a two-way ANOVA, calculated and visualized with GraphPad Prism (Version 9.0.0 for Windows, GraphPad Software, San Diego, California, USA). Differences in data points were considered significant at p < 0.05 (*), p < 0.01 (**), p < 0.001 (***).
Results
S. mutans biofilm formation on oral surfaces in the flow chamber
Using an in vitro flow chamber system, which mimicked the oral cavity microenvironmental conditions, mature biofilm of S. mutans was formed on HA discs with or without orthodontic brackets after 48 h. The structure of the biofilms analyzed by SEM revealed abundant adherent clusters of S. mutans on both, HA discs surface and on bonded orthodontic brackets. There was a notable increase in number of S. mutans cells on HA discs with brackets compared to HA discs without brackets (Figure S1).
The co-cultivation of S. mutans with S. salivarius K12 and/or S. salivarius M18 significantly reduced the biofilm formation on both surfaces (). To display the composition of the biofilm after the co-cultivation, CLSM combined with FISH was used for selective detection of bacteria (). Thus, pan-reactive probe SRT450 was used to stain all streptococci in the biofilm, while MUT590 probe specifically distinguished S. mutans (no signal on S. salivarius, ). CLSM validated further S. mutans and S. salivarius M18 co-clustering, indicating a higher proportion of the probiotic strain in the co-cultivation biofilm (). Analogous results were found when S. salivarius K12 was used (data not shown). Interestingly, neither S. salivarius K12 nor S. salivarius M18 adhered well to HA discs or other material when grown as monoculture. However, both strains formed a solid biofilm in the presence of S. mutans ( and ). Additionally, S. salivarius formed noticeable longer streptococcal chains after co-cultivation with S. mutans ().
Figure 2. Representative SEM images of the biofilm-coated HA discs after 48 h of anaerobic incubation in the flow chamber. S. mutans monoculture biofilm (negative control) formed on HA discs with brackets (a) and without brackets (c). S. salivarius M18 and S. mutans mixed biofilm formed on HA discs with (b) and without bonded orthodontic brackets (d) after 48 h of co-cultivation. For biofilm on HA discs with brackets, images were taken at x20. For biofilm on HA discs without brackets, images were taken at x50 and x3000.
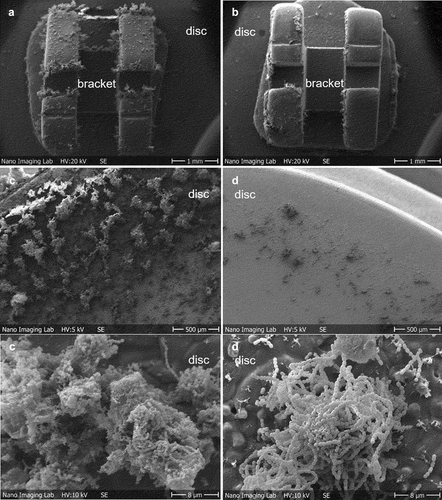
Figure 3. The composition of biofilm formed on HA discs after 48 h of incubation as monitored by FISH. Representative CLSM images of the biofilm formed by S. salivarius M18 (a) and S. mutans ATCC 25175 (b) monocultures, as well as S. mutans and S. salivarius M18 mixture after co-cultivation (c). Biofilms were labeled with MUT590 (green) and STR405 (red) probes.
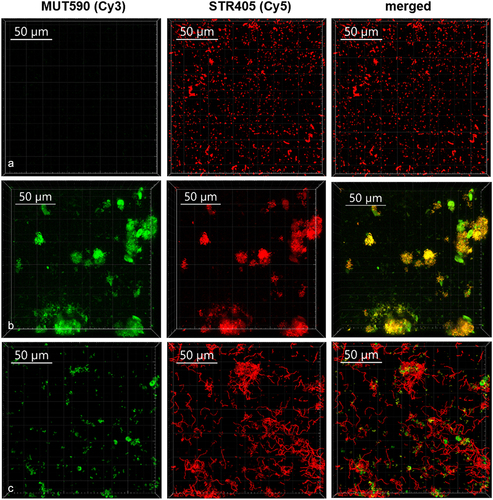
Antimicrobial activity of S. salivarius K12 and/or S. salivarius M18 in a co-cultivation model
The co-cultivation of S. mutans ATCC 25175 with S. salivarius K12 and/or S. salivarius M18 led to a complete inhibition of S. mutans viability in the planktonic suspension, i.e. the bacterial suspension present in the tubing of the flow chamber system after 48 h. In contrast, the cell numbers of either S. mutans grown as monoculture or the probiotic strains S. salivarius K12 and S. salivarius M18 when grown in the co-culture together with S. mutans, remained high but notably decreased over time (), presumably, because both were retained in the growing biofilms. Consequently, no viable S. mutans could be detected in the biofilm on HA discs after 48 h of co-cultivation ().
Figure 4. Antimicrobial activity of S. salivarius K12 and/or M18 against S. mutans in a co-cultivation model. (a) bacterial viability measured as CFU/ml for planktonic cells at the beginning (T0) and after 48 h (T2) of co-cultivation of S. mutans ATCC 25175 (light grey column) with S. salivarius K12 (grey column, left right diagonal hatch pattern), M18 (grey column, right left diagonal hatch pattern) resp. and K12/M18 mixture (dark grey column, crossed line hatch pattern). S. mutans monoculture was used as a control (light grey column). (b) bacterial viability measured as CFU/ml for biofilm-associated cells after 48 h (T2) of co-cultivation of S. mutans (light grey column) with S. salivarius K12 (grey column, left right diagonal hatch pattern), M18 (grey column, right left diagonal hatch pattern) and resp. K12/M18 (dark grey column, crossed line hatch pattern). S. mutans ATCC 25175 monoculture 48 h biofilm was used as a control (light grey column).
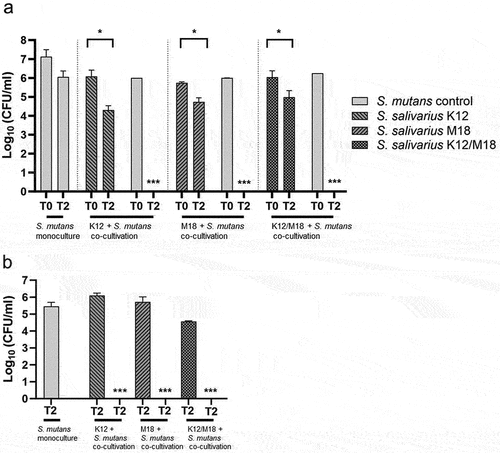
Antimicrobial activity of S. salivarius K12 and/or S. salivarius M18 - simultaneous antagonism method
Using a simultaneous antagonism assay, S. salivarius K12 and/or S. salivarius M18 exhibited antimicrobial activity against most of S. mutans isolates tested (). However, differences in sensitivity were noticed. Thus, S. mutans ZIB6095 was not inhibited by S. salivarius M18, but was sensitive against S. salivarius K12. Additionally, S. salivarius K12 and/or S. salivarius M18 were able to inhibit the known indicator strains M. luteus 6575, M. luteus 6592, and S. salivarius MU. When S. salivarius K12 and S. salivarius M18 were used simultaneously, no significant additive effects were found. Additionally, S. salivarius strains K12 and S. salivarius M18 inhibited each other ().
Table 2. Inhibition of bacterial growth by S. salivarius K12 and M18 as determined by the simultaneous antagonism test. (−) No inhibition; (+) slight inhibition (1–2.5 mm); (++) inhibition (2.5–4 mm); (+++) strong inhibition (4–11.0 mm).
Discussion
Despite being a preventable disease, dental caries remains a major public health problem for both children and adults [Citation19]. Especially, fixed orthodontic therapy enhances the risk for oral diseases due to increased plaque accumulation and white spot lesions [Citation3,Citation5–7]. Recently, probiotic approaches using S. salivarius lozenges have been developed to modulate the bacterial community in dental plaque by administering beneficial organisms that belong to the natural healthy plaque community [Citation10].
Approximately 700 bacterial species are known to be part of the oral microbiome. In the oral cavity, bacteria find various sites for attachment, especially on hard tissues such as teeth, where they can accumulate to form biofilms [Citation20]. They may coexist with their host; however, dysbiosis may occur, leading to oral diseases such as caries or periodontitis. Streptococcus species belong to the first colonizers of the oral cavity and are also ubiquitously present, making them the predominant genus in the oral cavity [Citation21]. S. salivarius belongs to the commensal oral flora and may even be beneficial to the host by producing molecules that inhibit pathogenic species. S. mutans is considered a cariogenic bacterium due to its ability to acidify the oral environment. In contrast, certain streptococcal species, such as S. gordonii or S. salivarius, are known to produce large amounts of alkali or generate hydrogen peroxide that can inhibit the growth of S. mutans [Citation21].
S. salivarius, in particular strains S. salivarius K12 and S. salivarius M18, are of interest as they offer numerous potential benefits for the host such as prevention and treatment of pharyngitis, caries and halitosis [Citation4,Citation22–24]. However, to date, the effect of S. salivarius isolates S. salivarius K12 and S. salivarius M18 on dental plaque formation reported in controlled clinical trials remains controversial [Citation4,Citation25]. Moreover, no standardized in vitro model, that would allow the analysis of different conditions, is available yet. Such a model could assist in analyzing the effect of individual S. salivarius probiotics or their use in combination and facilitate the comparison of these in vitro results with the varying results of clinical studies. In a randomized double-blind, placebo-controlled trial, Burton et al. reported that regular consumption of S. salivarius M18 for 3 months reduced dental plaque scores in children [Citation26]. Similarly, a significant decrease in plaque accumulation was reported in a pilot randomized clinical trial after four weeks of daily consumption of S. salivarius K12 lozenges within 31 patients [Citation27]. In contrast, a prospective, randomized, triple-blind, placebo-controlled trial with 64 patients undergoing fixed orthodontic treatment revealed that daily administration of S. salivarius M18 reduced the level of halitosis, but had minimal effects on plaque index (PI), gingival index (GI), and dental biofilm microflora [Citation4]. The reported differences might be attributed to the different treatment regimes, cultivation mode, or probiotics concentration. Therefore, the establishment of an adjustable in vitro model mimicking the dynamic condition found in the oral cavity is essential for investigating the effect of probiotics administration on dental biofilm formation. The dynamic flow chamber system used in this study allows the investigation of different parameters of biofilm formation in a low-shear environment with regular medium exchange, flexible cultivation mode, and offers the possibility to employ different oral surfaces with subsequent structural and functional analysis of the biofilms formed.
The cultivation of S. mutans in our flow chamber system with fixed orthodontic material showed a significant increase in S. mutans biofilm formation especially on orthodontic brackets and HA discs. Potentially, the larger surface area and niches of orthodontic brackets serve as colonization promoters for S. mutans biofilm formation on HA discs with bonded brackets in comparison to HA alone. These findings agree with previous observations, where orthodontic materials enhanced the presence of S. mutans in patients [Citation3,Citation28].
The reduction of dental plaque and decrease in the viable number of planktonic and biofilm-associated S. mutans, as shown in this study, might therefore be an effective measure for caries control. Both, S. salivarius K12 and/or S. salivarius M18 were able to reduce S. mutans biofilm formation on HA discs with or without brackets under anaerobic conditions. Closer examination of bacterial biofilm after co-cultivation revealed that, first, S. salivarius K12 and/or S. salivarius M18 adsorbed noticeable better on oral surfaces only in the presence of S. mutans. Second, in biofilm S. salivarius co-clustered with S. mutans micro-colonies adhering on top and around these colonies. The term coaggregation was previously described as a clumping phenomenon that occurred when sucrose-grown streptococci were co-cultivated with actinomyces species [Citation20]. Interestingly, the mechanism of coaggregation is essential for the development of complex biofilms and seems to be a prerequisite for antibacterial activities of colonizers such as S. salivarius. Our results are in accordance with the view that the structure and/or components of an existing biofilm provide a basis for the inhibition and even elimination of viability of caries-associated bacteria by S. salivarius. However, further studies concerning the mechanisms of S. salivarius adherence to dental biofilm are necessary.
The administration of a single strain and a combination of S. salivarius K12 and S. salivarius M18 fully inhibited the viability of S. mutans in planktonic culture and, therefore, prevented the formation of viable cariogenic biofilm. The selective antibacterial effect of S. salivarius on S. mutans including its various clinical isolates was also confirmed in the simultaneous antagonism assay. Notably, while being ineffective against the control strain B. fragilis, S. salivarius strains exhibited an antagonistic effect against each other. Moreover, the combination of both strains did not indicate any synergistic effect on S. mutans viability, which was claimed by the manufacturers. This might be explained by the diversity of lantibiotics gene profiles encoded by different S. salivarius strains [Citation10]. Both, S. salivarius K12 and S. salivarius M18 are producing multiple bacteriocins, each with its specific antimicrobial activity. S. salivarius K12 is known to produce the lantibiotics SalA and SalB, while whole-genome sequencing of S. salivarius M18 revealed a novel bacteriocin, salivaricin M, in addition to the already described lantibiotics SalA2 and Sal9 [Citation10]. Although the antibacterial activity of S. salivarius is most likely caused by these lantibiotics, its bacteriocin-deficiency variants need to be further tested to dissect the exact mechanism of strong S. mutans inhibition. Moreover, S. salivarius strains could produce other metabolites and enzymes such as urease with potentially modulatory activity on salivaricins and overall probiotic’s competitiveness [Citation21,Citation24].
Probiotics from oral S. salivarius species seem to have no negative impact on the salivary microbiome. Daily administration of S. salivarius K12 in a chewing gum did not significantly alter the salivary microbiome and did not increase inflammatory markers in the oral cavity of healthy patients [Citation11]. Furthermore, investigation of S. salivarius K12 effect on host epithelial cells revealed that probiotic bacteria downregulated inflammatory response while stimulating anti-inflammatory reactions and adhesive properties of the host cell [Citation11]. This makes S. salivarius a prominent probiotic for selective treatment of pathogenic dental biofilms.
While the in vitro model system used in this study is designed to simulate dynamic environment conditions during the dental biofilm formation, only a few parameters were tested so far. However, the flow chamber model would allow to further investigate time- and concentration-dependent application of probiotics on biofilm formed on various dental surfaces. Moreover, the effect of probiotics on pre-formed S. mutans biofilm is of particular interest for future studies. Finally, to optimize the effect of S. salivarius K12 and/or S. salivarius M18 application on dental biofilm, multispecies dental biofilm formed on various surface could be also tested with the current model.
Conclusions
The oral microbiome is a dynamic, complex system comprising multiple bacterial species that contribute to the pathogenic biofilms and subsequently cause caries and gingival inflammation of the surrounding tissues. Despite multispecies etiology, S. mutans remains one of the most common and, hence, most important cariogenic pathogen among oral streptococci. Natural antimicrobial compounds produced by oral probiotic streptococci can be utilized as a highly promising treatment option to eliminate S. mutans. In the present study, the in vitro flow chamber system allows the accurate simulation of daily administration of oral probiotics which was used to show antibacterial effect of S. salivarius K12 and S. salivarius M18 on S. mutans biofilm. Furthermore, our flow chamber system turned out to be especially useful for deciphering the complex dynamic behavior of the oral microbiome under the special caries-promoting conditions during orthodontic treatment.
Author contributions
Conception and design, E.R.; data curation E.R., V.S., L.A.; data analysis and interpretation, E.R., V.S., E.K.; original draft preparation, review and editing, E.R., V.S., E.K., C.V. and M.M.B. All authors have read and agreed to the published version of the manuscript.
Ethical approval
Clinical isolates used in this study were collected from human volunteers and stored anonymously, in accordance with the ethical standards of the institutional and national research committee and with the 1964 Helsinki declaration. All volunteers were informed about the study and upon agreeing to participate, their consent was registered. Based on the Swiss Federal Law on Research Involving Humans (Swiss Human Research Act Regulations 810.30, HRA: https://www.fedlex.admin.ch/eli/cc/2013/617/de), formal ethical approval is not applicable for the research, which involves anonymised biological material.
Bio_Author.docx
Download MS Word (18.6 KB)Acknowledgments
Elisabeth Reichardt would like to thank Prof. Jeremy Burton (Western University, Michigan, US), Prof. Werner Reichardt (Ernst-Abbe-University of Applied Sciences, Jena, Germany) and Dr. Maximilian Ole Bahls (ETH Zürich, Switzerland) for substantial support during developing the project and writing the manuscript. The authors would also like to express their gratitude to the UZB laboratory team including Prof. Tuomas Waltimo, Dr. Irmgard Hauser-Gerspach, Dr. Monika Astasov-Frauenhoffer, Elisabeth Filipuzzi-Jenny, Hedwig Wariwoda and to Susanne Erpel from the Nano Imaging Lab (University of Basel).
Disclosure statement
No potential conflict of interest was reported by the author(s).
Supplementary material
Supplemental data for this article can be accessed online at https://doi.org/10.1080/20002297.2024.2304971
Additional information
Funding
References
- Ximénez-Fyvie LA, Haffajee AD, Socransky SS. Microbial composition of supra- and subgingival plaque in subjects with adult periodontitis. J Clin Periodontol. 2000;27(10):722–10. doi: 10.1034/j.1600-051x.2000.027010722.x
- Astasov-Frauenhoffer M, Kulik EM. Cariogenic biofilms and caries from birth to old age. Monogr Oral Sci. 2021;29:53–64.
- Reichardt E, Geraci J, Sachse S, et al. Qualitative and quantitative changes in the oral bacterial flora occur shortly after implementation of fixed orthodontic appliances. Am J Orthod Dentofacial Orthop. 2019;156(6):735–44. doi: 10.1016/j.ajodo.2018.12.018
- Benic GZ, Farella M, Morgan XC, et al. Oral probiotics reduce halitosis in patients wearing orthodontic braces: a randomized, triple-blind, placebo-controlled trial. J Breath Res. 2019;31(13):036010. doi: 10.1088/1752-7163/ab1c81
- Höchli D, Hersberger-Zurfluh M, Papageorgiou SN, et al. Interventions for orthodontically induced white spot lesions: a systematic review and meta-analysis. Eur J Orthod. 2017;39:122–133. doi: 10.1093/ejo/cjw065
- Rafe Z, Vardimon A, Ashkenazi M. Comparative study of 3 types of toothbrushes in patients with fixed orthodontic appliances. Am J Orthod Dentofacial Orthop. 2006;130(1):92–5. doi: 10.1016/j.ajodo.2006.01.018
- Yaacob M, Worthington HV, Deacon SA, et al. Powered versus manual toothbrushing for oral health. Cochrane Database Syst Rev. 2014;6:CD002281. doi: 10.1002/14651858.CD002281.pub3
- Burton JP, Wescombe PA, Macklaim JM, et al. Persistence of the oral probiotic streptococcus salivarius M18 is dose dependent and megaplasmid transfer can augment their bacteriocin production and adhesion characteristics. PLoS One. 2013;13(8):e65991. doi: 10.1371/journal.pone.0065991
- Wescombe PA, Heng NC, Burton JP, et al. Streptococcal bacteriocins and the case for Streptococcus salivarius as model oral probiotics. Future Microbiol. 2009;4(7):819–835. doi: 10.2217/fmb.09.61
- Barbour A, Wescombe P, Smith PL. Evolution of Lantibiotic Salivaricins: new weapons to fight infectious diseases. Trends Microbiol. 2020;28(7):578–93. doi: 10.1016/j.tim.2020.03.001
- MacDonald KW, Chanyi RM, Macklaim JM, et al. Streptococcus salivarius inhibits immune activation by periodontal disease pathogens. BMC Oral Health. 2021;7(21):245. doi: 10.1186/s12903-021-01606-z
- Willey JM, van der Donk WA. Lantibiotics: peptides of diverse structure and function. Annu Rev Microbiol. 2007;61(1):477–501. doi: 10.1146/annurev.micro.61.080706.093501
- Jansen PM, Abdelbary MMH, Conrads G, et al. A concerted probiotic activity to inhibit periodontitis-associated bacteria. PLoS One. 2021;16(3):e0248308. doi: 10.1371/journal.pone.0248308
- Astasov-Frauenhoffer M, Braissant O, Hauser-Gerspach I, et al. Quantification of vital adherent Streptococcus sanguinis cells on protein-coated titanium after disinfectant treatment. J Mater Sci Mater Med. 2011;22(9):2045–51. doi: 10.1007/s10856-011-4377-5
- Millhouse E, Jose A, Sherry L, et al. Development of an in vitro periodontal biofilm model for assessing antimicrobial and host modulatory effects of bioactive molecules. BMC Oral Health. 2014;14(1):80. doi: 10.1186/1472-6831-14-80
- Gold OG, Jordan HV, Van Houte J. A selective medium for streptococcus mutans. Arch Oral Biol. 1973;18(11):1357–64. doi: 10.1016/0003-9969(73)90109-X
- Thurnheer T, Gmür R, Giertsen E, et al. Automated fluorescent in situ hybridization for the specific detection and quantification of oral streptococci in dental plaque. J Microbiol Methods. 2001;44(1):39–47. doi: 10.1016/S0167-7012(00)00226-8
- Astasov-Frauenhoffer M, Braissant O, Hauser-Gerspach I, et al. Isothermal microcalorimetry provides new insights into biofilm variability and dynamics. FEMS Microbiol Lett. 2012;337(1):31–7. doi: 10.1111/1574-6968.12007
- Selwitz RH, Ismail AI, Pitts NB. Dental caries. Lancet. 2007;369(9555):51–59. doi: 10.1016/S0140-6736(07)60031-2
- Kolenbrander PE, Palmer RJ Jr, Rickard AH, et al. Bacterial interactions and successions during plaque development. Periodontol 2000. 2006;42(1):47–79. doi: 10.1111/j.1600-0757.2006.00187.x
- Abranches J, Zeng L, Kajfasz JK, et al. Biology of oral streptococci. Microbiol Spectr. 2018;6(5):10.1128. doi: 10.1128/microbiolspec.GPP3-0042-2018
- Tagg JR, Harold LK, Jain R, et al. Beneficial modulation of human health in the oral cavity and beyond using bacteriocin-like inhibitory substance-producing streptococcal probiotics. Front Microbiol. 2023;14:1161155. doi: 10.3389/fmicb.2023.1161155
- Di Pierro F, Zanvit A, Nobili P, et al. Cariogram outcome after 90 days of oral treatment with streptococcus salivarius M18 in children at high risk for dental caries: results of a randomized, controlled study. Clin Cosmet Investig Dent. 2015;7:107–113. doi: 10.2147/CCIDE.S93066
- Burton JP, Chilcott CN, Moore CJ, et al. A preliminary study of the effect of probiotic Streptococcus salivarius K12 on oral malodour parameters. J Appl Microbiol. 2006;100(4):754–64. doi: 10.1111/j.1365-2672.2006.02837.x
- Ebrahim F, Malek S, James K, et al. Effectiveness of the lorodent probiotic lozenge in reducing plaque and streptococcus mutans levels in orthodontic patients: a double-blind randomized control trial. Front Oral Health. 2022;27:884683. doi: 10.3389/froh.2022.884683
- Burton JP, Drummond BK, Chilcott CN, et al. Influence of the probiotic streptococcus salivarius strain M18 on indices of dental health in children: a randomized double-blind, placebo-controlled trial. J Med Microbiol. 2013;62(6):875–84. doi: 10.1099/jmm.0.056663-0
- Babina K, Salikhova D, Polyakova M, et al. The effect of oral probiotics (streptococcus salivarius k12) on the salivary level of secretory Immunoglobulin A, salivation rate, and oral biofilm: a pilot randomized clinical trial. Nutrients. 2022;7(14):1124. doi: 10.3390/nu14051124
- Rosenbloom RG, Tinanoff N. Salivary streptococcus mutans levels in patients before, during, and after orthodontic treatment. Am J Orthod Dentofacial Orthop. 1991;100(1):35–7. doi: 10.1016/0889-5406(91)70046-Y