ABSTRACT
Background: Posttraumatic stress disorder (PTSD) related to exposure to abuse and neglect during childhood is associated with particularly severe and persistent deleterious outcomes. Amygdala hyperreactivity has been observed in childhood trauma survivors and implicated in symptoms of PTSD.
Objective: The neuropeptide oxytocin holds promise as a potential treatment for PTSD due to its ability to attenuate amygdala response to threat cues. However, the effect of oxytocin on amygdala reactivity in individuals with childhood trauma-related PTSD has not been investigated.
Method: We employed a double-blind, randomized, placebo-controlled crossover design to examine the effects of intranasal oxytocin (24 IU) versus placebo on amygdala reactivity to fearful faces among childhood-trauma exposed individuals with PTSD (n = 17) and without PTSD (control group; n = 16).
Results: Region-of-interest based amygdala fMRI signal magnitude did not differ by group, drug, or group x drug interaction. Self-report of childhood trauma exposure severity was negatively associated with the oxytocin-related change in left amygdala response in the PTSD group, but not in the control group. Supplementary and exploratory whole-brain analyses conducted separately in each group revealed that left amygdala reactivity to fearful faces was absent on placebo but increased on oxytocin in the control group. The PTSD group showed right amygdala activation to fearful faces in both the oxytocin and placebo conditions, but the left amygdala response observed in the placebo condition was diminished on oxytocin.
Conclusions: Findings extend the literature pertaining to the potential for oxytocin to attenuate neural correlates of PTSD to a childhood trauma-related PTSD sample.
HIGHLIGHTS
• We examined intranasal oxytocin effects on amygdala reactivity to fearful faces.• Participants were childhood trauma-exposed individuals with PTSD compared to resilient controls.• No group, drug, or group x drug interaction effects emerged.• In PTSD, more severe childhood trauma predicted greater amygdala change under oxytocin.
Antecedentes: El trastorno de Estrés Postraumático (TEPT) relacionado con exposición a abuso y negligencia durante la infancia se asocia con consecuencias deletéreas particularmente persistentes y severas. Se ha observado una hiperreactividad de la amígdala en sobrevivientes de trauma infantil y también se ha implicado en los síntomas de TEPT.
Objetivo: El neuropéptido oxitocina es prometedor como un potencial tratamiento para el TEPT debido a su habilidad de atenuar la respuesta de la amígdala frente a señales de amenaza. Sin embargo, el efecto de la oxitocina en la reactividad de la amígdala en individuos con TEPT relacionado a trauma infantil no ha sido investigado.
Método: Empleamos un diseño doble ciego, aleatorizado, cruzado placebo-control para examinar los efectos de la oxitocina intranasal (24 IU) versus placebo en la reactividad de la amígdala a las caras de miedo entre individuos expuestos a trauma infantil con TEPT (n=17) y sin TEPT (grupo control; n=16).
Resultados: La magnitud de la señal por IRMf de la amígdala basada en la región de interés no difirió por grupo, droga, o interacción droga x grupo. El auto-reporte de la severidad de la exposición a trauma infantil se asoció negativamente con el cambio asociado a oxitocina en la respuesta de la amígdala izquierda en el grupo con TEPT, pero no en el grupo control. Los análisis suplementarios de todo el cerebro revelaron que la reactividad de la amígdala izquierda a caras de miedo estuvo ausente con placebo, pero aumentó con la oxitocina en el grupo control. El grupo con TEPT mostró una activación de la amígdala derecha a caras de miedo en ambas condiciones, con oxitocina y con placebo, pero la respuesta de la amígdala izquierda observada en la condición de placebo estuvo disminuida con la oxitocina.
Conclusiones: Los hallazgos amplían la literatura sobre el potencial de la oxitocina para atenuar los correlatos neurales del TEPT a una muestra de TEPT relacionado con trauma infantil.
背景:与儿童期虐待和忽视有关的创伤后应激障碍(PTSD)与特别严重和长期的有害后果相关。在儿童创伤幸存者中观察到杏仁核过度反应,并且与PTSD的症状有关。
目的:由于其能够减弱杏仁核对威胁线索的反应,因此神经肽催产素有望作为PTSD的潜在治疗方法。然而,催产素对童年创伤相关PTSD个体的杏仁核反应性的影响尚未研究。
方法:我们采用双盲随机安慰剂对照交叉设计,考察鼻内催产素(24 IU)与安慰剂对有PTSD(n = 17)和无PTSD(对照组; n= 16)的童年创伤暴露个体中杏仁核对恐惧面孔的反应的影响。
结果:基于兴趣区的杏仁核fMRI信号幅度没有因组别、药物或组*药物的相互作用而不同。自我报告D童年创伤暴露严重程度与PTSD组中催产素相关的左侧杏仁核反应变化呈负相关,但在对照组中无关。补充的全脑分析显示,在对照组中安慰剂条件没有影响左侧杏仁核对恐惧面孔的反应,但催产素条件下增强。PTSD组在催产素和安慰剂条件下均表现出右侧杏仁核激活,但在安慰剂条件下观察到的左侧杏仁核反应在催产素条件下减弱。
结论:研究结果扩展了有关催产素可能减弱PTSD与儿童创伤相关PTSD样本的神经相关性的文献北京。
1. Introduction
Posttraumatic stress disorder (PTSD) is a chronic, debilitating psychiatric disorder with a lifetime prevalence of approximately 8% in the U.S. general population (Grant et al., Citation2015a; Kessler, Petukhova, Sampson, Zaslavsky, & Wittchen, Citation2012). Exposure to traumatic events during childhood and adolescence is highly prevalent and among the most common causes of PTSD (Green et al., Citation2010; Kessler, Sonnega, Bromet, Hughes, & Nelson, Citation1995; Koenen, Moffitt, Poulton, Martin, & Caspi, Citation2007). PTSD resulting from childhood trauma is particularly deleterious due to its negative and persistent mental and physical health consequences, complicated course of mental health treatment, and poor treatment outcomes (Agorastos et al., Citation2014; Goodwin and Stein; Kelleher et al., Citation2013; Nanni, Uher, & Danese, Citation2012; Tunnard et al., Citation2014). Consequently, identifying neural factors that contribute to PTSD risk and resiliency, and developing pharmacological interventions to reduce PTSD symptoms or mitigate risk for PTSD in this population, are areas of important scientific inquiry.
Figure 1. Overview of within-subjects crossover study design. Participants were randomly assigned to the oxytocin or placebo condition prior to the first scanning day (Visit 2), then were assigned to the opposite condition for the second scanning day (Visit 3).
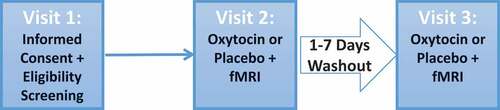
Dysregulation of limbic brain regions, such as the amygdala, is central to the pathophysiology of PTSD (Hayes, Hayes, & Mikedis, Citation2012; Rauch, Shin, & Phelps, Citation2006; Sripada et al., Citation2012). The amygdala is a critical mediator of compromised stress and fear reactivity and regulation, which are commonly considered to be hallmark features of PTSD symptomatology (Pitman et al., Citation2012). Neuroimaging studies have demonstrated hyperactivation of the amygdala during learning and emotional processing tasks among individuals with PTSD (Cisler, Citation2017; Geuze, Vermetten, Ruf, de Kloet, & Westenberg, Citation2008; Milad et al., Citation2009; Phelps et al., Citation2001; Sripada et al., Citation2012; Woodward, Neylan, Mellman, & Ross, Citation2006). Fearful face recognition tasks are well-established experimental paradigms commonly employed to probe amygdala reactivity in PTSD. Individuals with PTSD have been found to exhibit greater amygdala reactivity to both overt and implicit presentations of pictures depicting fearful faces as compared to individuals without PTSD (Rauch et al., Citation2000a; Shin et al., Citation2005).
Research also demonstrates evidence of long-term effects of childhood trauma on limbic brain regions with associated negative emotional processing biases (Dannlowski et al., Citation2013, Citation2012; Fonzo, Huemer, & Etkin, Citation2016; Grant et al., Citation2015b; Heim, Shugart, Craighead, & Nemeroff, Citation2010; Kaufman, Plotsky, Nemeroff, & Charney, Citation2000; Marusak, Martin, Etkin, & Thomason, Citation2015; Stevens et al., Citation2016; Teicher, Andersen, Polcari, Anderson, & Navalta, Citation2002; Veer et al., Citation2015). For example, there is evidence for strong positive associations between childhood trauma severity and right amygdala responsiveness to negatively valenced faces (Dannlowski et al., Citation2012). These findings hold significant clinical relevance: baseline amygdala hyperresponsivity to emotional stimuli including masked fearful faces has been found to be predictive of poorer PTSD treatment outcomes (Bryant et al., Citation2008; Fonzo et al., Citation2017; Norrholm et al., Citation2016). Moreover, improvement (e.g. restoring normative responsivity) in functioning of the amygdala and other limbic structures is associated with greater treatment gains (Cisler et al., Citation2015; Helpman et al., Citation2016; Laugharne et al., Citation2016). Identifying novel strategies for facilitating these neural changes in individuals who have experienced childhood trauma, such as pharmacotherapies that influence amygdala reactivity, could help to enhance response to PTSD treatment.
The neuropeptide oxytocin has been proposed to be involved in the pathophysiology of PTSD and thus might be a promising candidate to ameliorate its neural underpinnings (Olff, Langeland, Witteveen, & Denys, Citation2010). Central and systemic oxytocin administration exerts anxiolytic properties in animal models of stress (Chang, Barter, Ebitz, Watson, & Platt, Citation2012; Ring et al., Citation2006; Witt, Winslow, & Insel, Citation1992). Oxytocin has also been shown to ameliorate fear responses in humans (Labuschagne et al., Citation2010; Petrovic, Kalisch, Singer, & Dolan, Citation2008). Several studies in individuals without PTSD have found that oxytocin decreases the magnitude of amygdala responses to aversive stimuli and increases resting state connectivity among corticolimbic brain regions, suggesting that the anxiolytic properties of oxytocin may be mediated through a reduced amygdala response (Baumgartner, Heinrichs, Vonlanthen, Fischbacher, & Fehr, Citation2008; Dodhia et al., Citation2014; Domes et al., Citation2007; Kirsch et al., Citation2005; Kumar, Völlm, & Palaniyappan, Citation2015).
To date, there is only one published study of oxytocin effects on amygdala reactivity to facial affect cues in PTSD. Among police officers with PTSD, oxytocin attenuated left amygdala reactivity to emotional faces presented in an explicit face-matching task, regardless of valence, while increasing left amygdala reactivity to emotional faces in trauma-exposed police officers without PTSD (Koch et al., Citation2016b). Our objective is to extend this work to a sample of civilians with childhood-trauma related PTSD using briefly presented and masked fear faces based on evidence that automatic processing of threat cues is central to PTSD (Rabellino, Densmore, Frewen, Théberge, & Lanius, Citation2016). This work is critical because of the well-established complexity and longevity of symptomatology resulting from childhood trauma (Cloitre et al., Citation2009) and the emerging literature emphasizing the effects of individual differences, such as history of childhood maltreatment, on oxytocin response (Ebert et al., Citation2013; Flanagan, Baker, McRae, Brady, & Moran‐Santa Maria, Citation2015). Using a randomized, double-blind, placebo-controlled crossover design, we explored the effects of oxytocin (24 IU) on amygdala reactivity to briefly presented and masked fearful faces among individuals with PTSD resulting from childhood trauma exposure versus a control group comprised of individuals reporting childhood trauma exposure who did not develop PTSD. We expected to observe (1) greater amygdala reactivity to fearful faces in the PTSD group than in the control group and (2) that oxytocin would decrease amygdala reactivity to fearful faces in the PTSD group to approximate the reactivity of the control group.
2. Materials and methods
2.1. Participants
Thirty-eight individuals enrolled in the study. Five participants (two women in the PTSD group and two women and one man from the control group) were omitted from analyses due to poor quality of structural or functional images. Thus, 33 participants comprised the final sample: 17 participants (8 women; 9 men) in the PTSD group and 16 participants (10 women; 6 men) in the childhood trauma-exposed control group. Participants in the PTSD group and those in the control group were matched on sex, age, education, and smoking status (smoker vs. non-smoker). Women completed a menstrual cycle diary and were scheduled for their lab sessions during the luteal phase of their menstrual cycles to control for menstrual cycle variations in oxytocin response. Three participants in the PTSD group and three participants in the control group endorsed smoking.
Inclusion criteria for all study participants included: (1) scores of moderate to severe (>3) on a minimum of one item on at least one of the five trauma domains of the Childhood Trauma Questionnaire (CTQ; Bernstein et al., Citation2003) and (2) experiencing, witnessing, or confronting an event(s) that occurred prior to age 18 that involved actual or threatened death or serious injury, or a threat to the physical integrity of themselves or others and the person’s response involved intense fear, helplessness, and/or horror (i.e. meeting Diagnostic and Statistical Manual of Mental Disorders-IV [DSM-IV] Criterion A for PTSD).
General study exclusion criteria included (1) pregnancy, nursing, or ineffective means of birth control; (2) evidence of or a history of head trauma, neurological disorders, seizures, or unconsciousness; (3) current psychotic or bipolar affective disorder; (4) psychoactive substance use in the past 30 days as evidenced by participant report or urine drug screen; (5) unwillingness or inability to maintain abstinence from caffeine and alcohol for 24 h and drugs of abuse for 72 h prior to study visits; (6) ferrous metal implants/pacemaker; (7) claustrophobia; (8) history of or current significant haematological, endocrine, cardiovascular, pulmonary, renal, or gastrointestinal diseases.
Additionally, participants in the PTSD group were required to meet DSM-IV diagnostic criteria for current (i.e. past six months) PTSD. Participants were excluded from the PTSD group if they met DSM-IV diagnostic criteria for substance dependence in the past year (except for alcohol dependence). Exclusion criteria for the childhood trauma-exposed control group included (1) current or past (i.e. last 90 days) mood or anxiety disorders; (2) current/past substance dependence; and (3) psychotropic medications. Individuals who were free of psychotropic medications for the greater of the past 30 days or five half-lives prior to the study visits were eligible to participate. Current secondary psychiatric diagnoses were reported among eight participants in the PTSD group, including major depression (n = 2), ADHD (n = 1), alcohol use disorder (n = 1), panic disorder (n = 2), agoraphobia (n = 1), and dysthymia (n = 1).
2.2. Measures
Eligibility was established using the Mini-International Neuropsychiatric Interview (Sheehan et al., Citation1998). The Structured Clinical Interview for DSM-IV (SCID-I/PFirst, Frances, Pincus, Vettorello, & Davis, Citation1994) was used to assess current and lifetime substance use disorders diagnoses. Initially, the Clinician-Administered PTSD Scale (CAPS; Blake et al., Citation1995) was used to assess PTSD diagnosis and PTSD symptom severity. To minimize participant burden, the measure used for this purpose was changed to the Posttraumatic Diagnostic Scale (PDS; Foa, Cashman, Jaycox, & Perry, Citation1997). PTSD diagnosis assessments were linked to a childhood trauma index event. In this sample, six participants (three participants each in the PTSD and control groups, respectively) completed the CAPS while the remaining 27 participants completed the PDS. The Childhood Trauma Questionnaire (CTQ; Bernstein et al., Citation2003) was used to assess severity of exposure to potentially traumatic events such as abuse and neglect during childhood. Participants completed a medical history and physical examination to assess medical exclusions. The state anxiety subscale of the State-Trait Anxiety Inventory (STAI; Spielberger, Gorsuch, Lushene, Vagg, & Jacobs, Citation1983) was administered immediately before and after each fMRI scan in order to assess participants’ subjective anxiety.
2.3. Procedures
The overall study procedures are depicted in . Participants were recruited via local media advertisements over a 33-month period. All procedures were approved by the Medical University of South Carolina’s (MUSC) Institutional Review Board and conducted consistent with the Declaration of Helsinki. Written informed consent was obtained from each participant prior to any study procedures.
Participants who met eligibility requirements were scheduled to complete two fMRI sessions. Participants arrived at the laboratory at 9:30 a.m. each day. All participants were breathalysed and completed a urine drug screen; women also completed a urine pregnancy test. The MUSC research pharmacy compounded and dispensed the oxytocin nasal spray (24 IU) and matching placebo (saline) and was responsible for treatment randomization. Half of the participants were randomized to receive placebo on scanning visit one while the other half received oxytocin on scanning visit one. While 24 IU is the most commonly used dosage in research studies, it was also recently validated as the most effective dosage for modulating the amygdala in response to emotional faces (Spengler et al., Citation2017). Under the supervision of the research staff, participants self-administered the nasal sprays approximately 45 min prior to the start of each scanning session. All but three participants in the study completed the fMRI scans within one week of each other, with many (n = 16; 10 participants in the PTSD group, 6 in the control group) completing the second scan within 24 h. Participants were not required to complete the fMRI scans at the identical time of day. Intranasal oxytocin has a half-life of approximately 3–4 h, thus, 24 h is an adequate minimum washout period commonly employed in the clinical oxytocin literature (Koch et al., Citation2016a; Palgi, Klein, & Shamay-Tsoory, Citation2016).
2.4. fMRI data acquisition
Images were acquired on a Siemens Trio 3.0-T scanner with a 12-channel head coil (Siemens Medical, Erlangen, Germany). During initial scanner tuning, localizing, and structural scanning, participants were shown relaxation images (i.e. 20 scenic pictures, each displayed for 30 s). A high-resolution T1-weighted MPRAGE anatomical scan (TR = 8.1 ms, TE = 3.7 ms, flip angle = 8°, field of view = 256 mm, 1.0 mm) covering the entire brain and positioned using a sagittal scout image was acquired for co-registration and normalization of functional images. T2*-weighted gradient echo EPI images were acquired with the following parameters: TR = 2500 ms, TE = 27 ms, flip angle = 77º, 40 axial slices (FOV = 224 x 224 mm, thickness = 3.5 mm voxels with 0.5 mm gap, in interleaved order, 160 volumes). The scanning planes were oriented parallel to the anterior commissure–posterior commissure line.
2.5. Implicit facial affect recognition task
The facial affect recognition task (see ) was modelled after studies by Rauch et al. (Citation2006, p. 1998), which used brief stimuli presentations and neutral face masks to target automatic processing of threat cues (i.e. fearful faces). To minimize explicit and central executive processing of facial affect in this task, participants were required to identify the gender of the neutral face masks. Kouider and Dehaene (Citation2007) suggested that brief presentations (i.e. 50 ms or less), visual masking and redirection of central processing are effective at inducing implicit or automatic processing. Emotional adult faces were selected from a variety of validated stimuli sets (Ekman & Friesen, Citation1976; Langner et al., Citation2010; Tottenham et al., Citation2009). Images were standardized in size and enclosed in the same oval surround. The faces depicted men and women of Caucasian, Asian, and African American ethnicities expressing three different categories of emotion: fear, anger, and happiness. Angry and happy faces were included for the purpose of testing the specificity of oxytocin effects on fear processing in PTSD. In a block design, participants viewed a series of 56 same-gender faces depicting the same emotion within a block; participants were then prompted to identify the gender of the faces. Each emotional face was presented for 50 ms followed by a neutral facemask (from a different individual) for 167 ms, then a blank screen for 291 ms. At the end of the block, participants reported the gender using two buttons on a response pad. Assignment of face sets to sessions was counterbalanced across subjects. The task included 6 pseudorandomly ordered task blocks (3 emotions x 2 genders) and 7 rest blocks (27.5 s each) consisting of a crosshair on the screen.
Figure 2. Illustration of the facial affect recognition task. (a) Six task blocks depicting male or female faces expressing angry, happy or fear emotions were interleaved with rest blocks consisting of a central crosshair for visual fixation. Each task block lasted 27.8 s followed by a 5-s gender identification block. Each rest block lasted 27.5 sec. (b) Illustration of a single trial within each task block. An emotional face was presented for 50 ms followed by a neutral face mask for 167 or 183 ms followed by a completely blank screen for 291 ms. Fifty-six trials were presented in a single task block. (c) Illustration of the gender identification block, which lasted 5 s. Subjects were asked ‘What gender were the faces that you just saw? MALE – Press Thumb, FEMALE – Press Index Finger’.
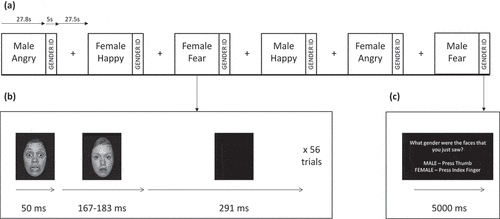
2.6. Statistical methods
Based on the literature associated with our primary outcome (BOLD signal change in the amygdala) available at the time of study design, the appropriate effect size for this study ranged between 1.2 and 1.4 (Felmingham et al., Citation2010; Rauch et al., Citation2000b). To detect an effect size (f) of 1.2 with a type 1 error protection level of .05 and n = 12, this study with group n = 15 had power (1 ß) of .80 (Cohen, Citation1988).
Data were preprocessed using FMRI Expert Analysis Tool (FEAT) Version 5.63, part of FMRIB’s Software Library (FSL; www.fmrib.ox.ac.uk/fsl). The 4D images for each subject were corrected for head motion using MCFLIRT, then spatially smoothed (FWHM = 8.0 mm) and temporally filtered (cutoff = 100 s). Grand mean intensity normalization was applied to each 4D dataset. MCFLIRT was used to register each subject’s 4D image to MNI standard space using the high-resolution T1 MPRAGE image. For the first-level analysis conducted separately in each subject, each of the experimental conditions (rest, angry, fear, happy and gender identification phase) was an explanatory variable with double-gamma HRF convolution applied and temporal derivatives added. The six rotations and displacements of head movement were added as confound variables. Time-series statistical analysis was carried out using FILM with local autocorrelation correction (Woolrich, Ripley, Brady, & Smith, Citation2001).
Left and right amygdala regions of interest (ROI) were defined using the Harvard-Oxford probabilistic structural atlas, then featquery was used to extract the contrast of parameter estimate (cope) value for the Fear > Rest contrast converted into percent signal change. For the primary ROI analysis, analysis of variance (ANOVA) was used to examine the effect of group (PTSD vs. control), drug (oxytocin vs. placebo) and the group x drug interaction on activation (percent signal change) separately in the left and right amygdala. Although the fear versus rest contrast does not control for non-specific responses associated with conscious perception of neutral faces, no literature exists to suggest that perceiving neutral faces should differ between PTSD and trauma-exposed participants (Bell, et al., Citation2017). Thus, our a priori hypothesis focused on fear versus rest rather than fear versus another emotional condition. Secondary ANOVAs examined the effect of group, drug and emotion (fear, angry, happy versus rest cope values converted to percent signal change) to determine whether group or drug effects for fearful faces also emerged for happy or angry faces.
Because the hypothesis-driven ROI analysis focused only on left and right amygdala, a supplementary whole-brain voxel-wise analysis was conducted to explore additional brain regions that might be modulated by drug or subject group for fearful faces versus rest. This analysis was not conducted to test the specific hypotheses for this study, but rather to explore additional regions of activation. In this analysis, separate PTSD and childhood trauma-exposed group statistical maps for the Fear > Rest contrast in the placebo and oxytocin conditions were generated using multilevel linear modelling (FLAME1; Beckmann, Jenkinson, & Smith, Citation2003; Woolrich, Citation2008; Woolrich, Behrens, Beckmann, Jenkinson, & Smith, Citation2004) and cluster thresholded using Z > 2.3 and corrected p = .05 (Worsley, Citation2001). Separate activation maps were examined for each Group x Drug condition due to small sample sizes.
3. Results
3.1. Demographics and descriptive statistics
As presented in , participants in the PTSD group had a higher total CTQ score compared to the childhood trauma-exposed control group (t = 3.70, p < 0.001). The PTSD group reported more emotional abuse (t = 2.02, p = 0.05), physical abuse (t = 2.78, p = 0.05), sexual abuse (t = 2.41, p < 0.05), emotional neglect (t = 2.70, p = 0.01) and physical neglect (t = 3.27, p < 0.001) as compared to the control group. The PTSD group also reported more subjective anxiety before (t = 5.52, p < 0.001; t = 4.97, p < 0.001) and after (t = 2.67, p= 0.01; t = 2.61, p = 0.01) both fMRI scans compared to the control group.
Table 1. Demographic and clinical characteristics by group.
3.2. Regions of interest: left and right amygdala
The primary group x drug ANOVA revealed no differences in fMRI signal magnitude (Fear > Rest) between the PTSD and the control group within either the left (f1, 31 = 0.78, p = .38, ηp2 = .025) or right (f1, 31 = 0.24, p = .63, ηp2 = .008) amygdala ROIs. There was no significant main effect of drug condition (f1, 31 = 1.1, p = .31, ηp2 = .033) or drug condition x group interaction (f1, 31 = 1.2, p = .28, ηp2 = 0.037) in the left amygdala response. Similarly, there were no significant effects of drug condition (f1, 31 = 1.2, p = .29, ηp2 = .036) or drug condition x group interaction (f1, 31 = .19, p = 0.67, ηp2 = 0.006) in the right amygdala response (). The secondary group x drug x emotion ANOVA to examine specificity of fear processing revealed that there were no significant effects of drug, emotion, group or their 2- and 3-way interactions on activation within left or right amygdala (see ).
3.3. Correlations with childhood Trauma
Correlations between CTQ scores and the change in amygdala response due to drug condition (Oxytocin – Placebo percent signal change for Fear > Rest in each amygdala ROI) were examined separately in the PTSD and childhood trauma-exposed control groups. There was a significant negative correlation between oxytocin-related fMRI signal change and CTQ scores in both left amygdala (rho = −.54, p = .024) and right amygdala (rho = −.48, p= .053) in the PTSD group but not in the control group (p’s > .20; ). Secondary correlations examining the association between CTQ and change in amygdala response for Happy > Rest and Anger > Rest conditions were not significant for either amygdala ROI (p’s > .15)
Figure 4. Scatterplots illustrating the correlation between change in (a) left amygdala and (b) right amygdala fMRI response due to drug (Oxytocin minus Placebo) as a function of Childhood Trauma Questionnaire scores. Positive Oxytocin minus Placebo difference scores reflect greater amygdala activation on oxytocin and negative Oxytocin minus Placebo difference scores reflect greater amygdala activation on placebo. OT = Oxytocin. PBO = Placebo. PTSD = Posttraumatic stress disorder group. SD = Standard deviation.
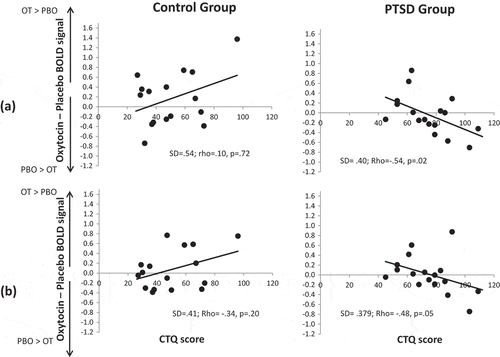
3.4. General linear model supplementary analyses
Fear versus rest activation across the whole brain in the placebo and oxytocin conditions for the PTSD and childhood trauma-exposed groups are shown in and local maxima within clusters provided in . shows that the amygdala was significantly activated for some of the contrasts but the amygdala did not yield a local maximum, as shown in . In the childhood trauma-exposed control group, the left amygdala response was absent in the placebo condition, but present in the oxytocin condition. The right amygdala response was present under both conditions. In contrast, the PTSD group showed right amygdala activation, which fell in the right inferior occipital cluster, after both oxytocin and placebo, but the left amygdala response was absent on oxytocin and present on placebo. For both groups, the brain stem showed activation in the oxytocin condition but these areas were not activated in the placebo condition. The PTSD group showed activation in the paracingulate gyrus on oxytocin but not on placebo.
Table 2. Clusters and local maxima for activation in the Fear > Rest contrast for each group and drug condition.
Figure 5. Results of the voxel-wise general linear model analysis for the Fear > Rest contrast for each participant group (PTSD and childhood trauma-exposed groups) and drug condition (yellow = placebo, blue = oxytocin). Activation is significant at a cluster-corrected threshold of Z > 2.33, p = .05. Crosshairs in each brain slice are centred on left amygdala, right amygdala or right paracingulate region. MNI x-coordinate is shown for each slice.
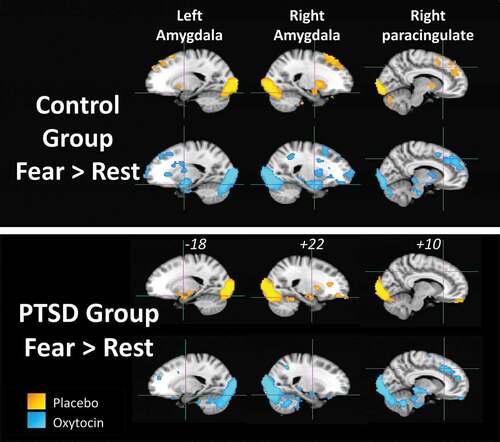
4. Discussion
This study explored the effects of a single 24 IU dose of intranasal oxytocin on amygdala reactivity to briefly presented and masked fearful faces among individuals with PTSD related to childhood trauma exposure compared to individuals with childhood trauma exposure without PTSD. In the primary ROI analyses (depicted in ) we did not find diagnostic (PTSD vs. no PTSD), drug (oxytocin vs. placebo), or interaction (diagnostic x drug) effects on amygdala responses to fearful faces. However, correlations between childhood trauma severity and drug-related change in amygdala response (depicted in ) revealed that participants in the PTSD group who reported more severe childhood trauma exposure demonstrated less amygdala reactivity to fearful faces on oxytocin than on placebo, an effect that did not generalize to angry or happy faces. By utilizing both analyses, this exploratory study examined both overall outcomes and addressed an important question of how overall results are modulated by trauma exposure severity. Existing literature indicates that trauma exposure severity and chronicity may be important considerations in the maintenance and treatment of PTSD symptoms in various populations (Bedard‐Gilligan et al., Citation2015; Hendriks, De Kleine, Broekman, Hendriks, & Van Minnen, Citation2018; Jakob, Lamp, Rauch, Smith, & Buchholz, Citation2017). The current findings, although preliminary and exploratory, add to the developing literature by showing that oxytocin dampens amygdala reactivity to fear cues among more severely childhood-trauma exposed individuals with PTSD, thus providing modest support for the premise that oxytocin may target neural mechanisms of resilience and recovery in this particularly high-risk population.
Intranasal oxytocin may attenuate the amygdala hyperresponsivity to subconscious threat cues that is central to the development and maintenance of PTSD (Rabellino et al., Citation2016) and predicts behavioural PTSD treatment response (e.g. Fonzo et al., Citation2017). Oxytocin has been proposed as a potential pharmacotherapeutic strategy for enhancing response to behavioural interventions for PTSD based on evidence of its effects on fear regulation (Koch et al., Citation2014), with preliminary support of acceptability and feasibility from a recent pilot study of oxytocin-enhanced Prolonged Exposure (Flanagan, Sippel, Wahlquist, Moran-Santa Maria, & Back, Citation2017). To date, however, there are still very few studies examining basic effects of oxytocin on clinically relevant behavioural and neural processes among individuals with PTSD. Extant research has shown that oxytocin enhances working memory, neural responses to social reward, and connectivity within neural networks that support emotion regulation while blunting amygdala responses to emotional stimuli (Flanagan et al., Citation2018b; Frijling et al., Citation2016; Koch et al., Citation2016a, Citation2016b; Nawijn et al., Citation2016). A related line of research has examined the ability of oxytocin to enhance fear extinction and ameliorate exacerbated responses to conditioned stimuli among individuals with anxiety disorders and PTSD (Acheson et al., Citation2013; Eckstein et al., Citation2014; Petrovic et al., Citation2008). Lastly, one study has examined the ability of oxytocin to prevent onset of PTSD with overall null findings but positive effects for participants who showed elevated distress immediately posttrauma (van Zuiden et al., Citation2016). These are important lines of inquiry which might be informed by the current study’s findings on amygdala reactivity because fear extinction is an amygdala-mediated process central to effective PTSD treatment (Rauch et al., Citation2006). Therefore, future research should examine whether oxytocin added to evidence-based psychotherapies for PTSD improves treatment response by reducing amygdala hyperresponsivity to emotional stimuli. It also remains possible that oxytocin could have beneficial effects on PTSD prevention. Future studies should replicate and refine the design employed by previous studies such as that by van Zuiden et al. (Citation2016) to more thoroughly test this hypothesis.
Existing literature has demonstrated that in addition to the hallmark symptoms of PTSD relating to fear such as avoidance and reexperiencing, symptoms such as numbing and hyperarousal significantly and severely impact the wellbeing and functioning of individuals with PTSD (Hellmuth, Jaquier, Young-Wolff, & Sullivan, Citation2013; Zuj, Palmer, Lommen, & Felmingham, Citation2016). Our preliminary and exploratory findings regarding conditions other than fear (i.e. angry and happy faces) provide a basis to inform the design and implementation of future studies aiming to target such processes known to be relevant to the day-to-day symptom experience among individuals with PTSD.
The lack of a difference in fear-related amygdala responsivity between individuals with PTSD and childhood trauma-exposed controls was somewhat unexpected given that previous studies report strong amygdala hyper-reactivity to emotion and trauma stimuli (Hayes et al., Citation2012; Shin, Rauch, & Pitman, Citation2006). However, others have similarly found no differences in amygdala responses to fearful or trauma cues when individuals with PTSD are compared to trauma-matched control groups (Fonzo et al., Citation2016; Koch et al., Citation2016a; Shin et al., Citation2006). In addition, one meta-analysis found that amygdala hyperactivity was found in studies that compared individuals with PTSD to healthy controls without a history of trauma exposure (Shin et al., Citation2006). Finally, alterations in amygdala activity have been found in healthy individuals who report a significant history of childhood trauma, but do not meet diagnostic criteria for a psychiatric disorder (Dannlowski et al., Citation2013). Thus, it is possible that trauma exposure itself, rather than PTSD, may lead to hyperactive amygdala responses to negatively valenced stimuli.
In addition to the current study’s main finding that amygdala hyperresponsivity to fearful faces was reduced in those PTSD subjects who reported more childhood trauma, the supplemental voxel-wise whole-brain analyses revealed that in the trauma control group, left amygdala reactivity was present on oxytocin but absent on placebo. Although the voxel-wise analysis was exploratory and did not statistically compare oxytocin and placebo in the trauma control group, these preliminary findings are broadly in line with findings from a study showing that oxytocin reduced amygdala responses to explicitly presented emotional faces in police officers with PTSD but increased amygdala responses in matched controls (Koch et al., Citation2016b).
Additional research is necessary to clarify the characteristics of individuals who might benefit from oxytocin treatment, and at what point in the development or maintenance of symptomatology oxytocin would ideally be administered. Existing literature has identified individual and contextual differences that influence the effects of oxytocin on neurobiological and behavioural responses among individuals with trauma exposure and PTSD. In the current study, groups were balanced by sex because sex is known to influence oxytocin responses (Ditzen et al., Citation2012; Flanagan et al., Citation2018a; Rilling et al., Citation2014). Our finding of less fear-related amygdala reactivity among more severely childhood-trauma exposed PTSD individuals under oxytocin further emphasizes the importance of considering factors such as trauma exposure severity, chronicity, and date/developmental stage of onset when testing how to facilitate the effective translation of oxytocin for clinical use.
These findings, while preliminary, might also inform future studies examining the potential for oxytocin to enhance adaptive emotion recognition and empathy among individuals with a criminal offense history. Specifically, previous studies demonstrate that individuals with a criminal history or propensity for maladaptive social behaviour (e.g. aggression and violent behaviour) display low anxiety, poorer recognition of fear, and low empathy (Blake & Gannon, Citation2008). Abundant literature shows that within certain contexts, oxytocin has the potential to enhance prosocial behavioural and cognition including empathy (Hurlemann et al., Citation2010), but other literature demonstrates that oxytocin can enhance maladaptive social behaviour (Flanagan et al., Citation2018a; Pfundmair, Reinelt, DeWall, & Feldmann, Citation2018). Future studies should examine the ability of oxytocin to correct hyporeactivity in the amygdala and limbic regions in response to facial affect, especially among individuals with known social deficits and propensity toward maladaptive social behaviour.
5. Limitations
Several limitations of the current exploratory study should be considered. One limitation is that the severity of childhood trauma exposure as measured by the CTQ was greater in the PTSD group compared to the control group, though variability in childhood trauma was similar between groups as evidenced by similar standard deviations. Future studies can improve on the current sampling plan by considering more rigorous minimum inclusion criteria related to childhood trauma. Future studies can also improve on this design by increasing the specificity of inclusion criteria for PTSD versus control groups. Future studies should also include an assessment of endogenous oxytocin levels at one or more time points given the lack of literature examining whether and how intranasal oxytocin and basal levels of oxytocin might interact (Busnelli et al., Citation2016; Russell et al., Citation2018; Sippel et al. in preparation).
While this study was designed as a preliminary proof-of-concept project and employs a within-subjects crossover design to maximize statistical power, it is possible that the expected effects of oxytocin might have been more difficult to detect than anticipated upon the initial study design. More specifically, while the overall patterns that emerged from our study are useful to inform future studies, they are preliminary and should be interpreted with caution. Related to this challenge is that the small sample size limited our supplementary whole-brain analyses to an exploratory approach to comparing oxytocin versus placebo effects in trauma-exposed and PTSD groups. More adequately powered studies with larger samples of more diverse participants are also necessary to examine the potentially unique effects of oxytocin on the neural correlates of PTSD resulting from trauma exposure during adulthood versus childhood. Larger samples would also allow for examination of potential moderators of oxytocin response, such as sex differences in oxytocin effects on amygdala functioning; however, sex differences in amygdala responses among PTSD samples are not reliably observed (e.g. Felmingham et al., Citation2010). While the previous study of oxytocin effects on emotional face processing in individuals with PTSD and resilient control participants found no sex differences (Koch et al., Citation2016b), another study found sex-dependent effects of oxytocin on reactivity to a facial processing task among healthy adults (Luo et al., Citation2017). While the type and severity of childhood trauma exposure varied widely in this sample, age at which participants experienced childhood trauma exposure(s) was not recorded. Future studies can improve on this by examining the potential role of chronicity of trauma exposure in oxytocin response. While extending external validity of the findings, these characteristics might influence amygdala responses. It will also be important for future studies to consider the importance of examining the association between PTSD symptom severity and trauma exposure severity in relation to oxytocin effects on amygdala responses. The inclusion of left-handed individuals, which was not formally assessed, is another potential limitation of this study. However, previous research has shown that amygdala responses to faces are not lateralized as some other cognitive such as speech might be.
To improve internal validity, these findings should be replicated among individuals with PTSD only and those who are either required to be stable on medication doses or free of psychiatric medications. Another limitation is that the measure used to establish PTSD diagnosis was changed early in the study to reduce participant burden. While both measures have excellent psychometric properties, an ideal design would remain consistent for all participants. Generalizability is limited to individuals who experienced childhood trauma; future research can examine effects of oxytocin on fear-related processes in PTSD related to other types of trauma.
6. Conclusions
The current exploratory study did not find differences in recruitment of the amygdala in response to fearful faces between participants in the PTSD group and those in the childhood trauma-exposed control group. However, individual variation in oxytocin’s ability to modulate amygdala response depending on severity of childhood trauma exposure provides an extension of the existing literature conducted among adults with recent PTSD and trauma-exposed resilient adults. Future adequately powered studies are necessary to translate these findings into human laboratory and neuroimaging trials using repeated oxytocin doses and larger PTSD treatment and prevention-focused clinical trials.
Acknowledgments
None.
Disclosure statement
No potential conflict of interest was reported by the authors.
Additional information
Funding
References
- Acheson, D., Feifel, D., de Wilde, S., Mckinney, R., Lohr, J., & Risbrough, V. (2013). The effect of intranasal oxytocin treatment on conditioned fear extinction and recall in a healthy human sample. Psychopharmacology, 229, 199–16.
- Agorastos, A., Pittman, J. O. E., Angkaw, A. C., Nievergelt, C. M., Hansen, C. J., Aversa, L. H., … Baker, D. G. (2014). The cumulative effect of different childhood trauma types on self-reported symptoms of adult male depression and PTSD, substance abuse and health-related quality of life in a large active-duty military cohort. Journal of Psychiatric Research, 58, 46–54.
- Baumgartner, T., Heinrichs, M., Vonlanthen, A., Fischbacher, U., & Fehr, E. (2008). Oxytocin shapes the neural circuitry of trust and trust adaptation in humans. Neuron, 58, 639–650.
- Beckmann, C. F., Jenkinson, M., & Smith, S. M. (2003). General multilevel linear modeling for group analysis in FMRI. Neuroimage, 20, 1052–1063.
- Bedard‐Gilligan, M., Duax Jakob, J. M., Doane, L. S., Jaeger, J., Eftekhari, A., Feeny, N. C., & Zoellner, L. A. (2015). An investigation of depression, trauma history, and symptom severity in individuals enrolled in a treatment trial for chronic PTSD. Journal of Clinical Psychology, 71, 725–740.
- Bell, C. J., Colhoun, H. C., Frampton, C. M., Douglas, K. M., McIntosh, V. V., Carter, F. A., ... Loughlin, A. (2017). Earthquake brain: Altered recognition and misclassification of facial expressions are related to trauma exposure but not posttraumatic stress disorder. Frontiers in Psychiatry, 8, 278.
- Bernstein, D. P., Stein, J. A., Newcomb, M. D., Walker, E., Pogge, D., Ahluvalia, T., … Zule, W. (2003). Development and validation of a brief screening version of the childhood Trauma questionnaire. Child Abuse & Neglect, 27, 169–190.
- Blake, D. D., Weathers, F. W., Nagy, L. M., Kaloupek, D. G., Gusman, F. D., Charney, D. S., & Keane, T. M. (1995). The development of a clinician-administered PTSD scale. Journal of Traumatic Stress, 8, 75–90.
- Blake, E., & Gannon, T. (2008). Social perception deficits, cognitive distortions, and empathy deficits in sex offenders: A brief review. Trauma, Violence, & Abuse, 9, 34–35.
- Bryant, R. A., Felmingham, K. L., Kemp, A. H., Das, P., Hughes, G., Peduto, A., & Williams, L. (2008). Amygdala and ventral anterior cingulate activation predicts treatment response to cognitive behaviour therapy for post-traumatic stress disorder. Psychological Medicine, 38, 555–561.
- Busnelli, M., Dagani, J., de Girolamo, G., Balestrieri, M., Pini, S., Saviotti, F. M., … Chini, B. (2016). Unaltered oxytocin and vasopressin plasma levels in patients with schizophrenia after 4 months of daily treatment with intranasal oxytocin. Journal of Neuroendocrinology, 28, 1–7.
- Chang, S. W., Barter, J. W., Ebitz, R. B., Watson, K. K., & Platt, M. L. (2012). Inhaled oxytocin amplifies both vicarious reinforcement and self reinforcement in rhesus macaques (Macaca mulatta). Proceedings of the National Academy of Sciences of the USA, 109, 959–964.
- Cisler, J. M. (2017). Childhood Trauma and functional connectivity between Amygdala and medial prefrontal cortex: A dynamic functional connectivity and large-scale network perspective. Frontiers in Systems Neuroscience, 11, 29.
- Cisler, J. M., Sigel, B. A., Kramer, T. L., Smitherman, S., Vanderzee, K., Pemberton, J., & Kilts, C. D. (2015). Amygdala response predicts trajectory of symptom reduction during trauma-focused cognitive-behavioral therapy among adolescent girls with PTSD. Journal of Psychiatric Research, 71, 33–40.
- Cloitre, M., Stolbach, B. C., Herman, J. L., Van der Kolk, B., Pynoos, R., Wang, J., & Petkova, E. (2009). A developmental approach to complex PTSD: Childhood and adult cumulative trauma as predictors of symptom complexity. Journal of Traumatic Stress, 22, 399–408.
- Cohen, J. (1988). Statistical power for the social sciences. Hillsdale, NJ: Laurence Erlbaum and Associates.
- Dannlowski, U., Kugel, H., Huber, F., Stuhrmann, A., Redlich, R., Grotegerd, D., … Suslow, T. (2013). Childhood maltreatment is associated with an automatic negative emotion processing bias in the amygdala. Human Brain Mapping, 34, 2899–2909.
- Dannlowski, U., Stuhrmann, A., Beutelmann, V., Zwanzger, P., Lenzen, T., Grotegerd, D., … Kugel, H. (2012). Limbic scars: Long-term consequences of childhood maltreatment revealed by functional and structural magnetic resonance imaging. Biological Psychiatry, 71, 286–293.
- Ditzen, B., Nater, U. M., Schaer, M., La Marca, R., Bodenmann, G., Ehlert, U., & Heinrichs, M. (2012). Sex-specific effects of intranasal oxytocin on autonomic nervous system and emotional responses to couple conflict. Social Cognitive and Affective Neuroscience, 8, 897–902.
- Dodhia, S., Hosanagar, A., Fitzgerald, D. A., Labuschagne, I., Wood, A. G., Nathan, P. J., & Phan, K. L. (2014). Modulation of resting-state Amygdala-frontal functional connectivity by oxytocin in generalized social anxiety disorder. Neuropsychopharmacology, 39, 2061–2069.
- Domes, G., Heinrichs, M., Glascher, J., Buchel, C., Braus, D. F., & Herpertz, S. C. (2007). Oxytocin attenuates amygdala responses to emotional faces regardless of valence. Biological Psychiatry, 62, 1187–1190.
- Ebert, A., Kolb, M., Heller, J., Edel, M., Roser, P., & Brüne, M. (2013). Modulation of interpersonal trust in borderline personality disorder by intranasal oxytocin and childhood trauma. Social Neuroscience, 8, 305–313.
- Eckstein, M., Becker, B., Scheele, D., Scholz, C., Preckel, K., Schlaepfer ... Hurlemann, R. (2014). Oxytocin facilitates the extinction of conmeasuring facial movement environmental psychology and nonverbal behaviorditioned fear in humans. Biological Psychiatry, 78, 194–202.
- Ekman, P., & Friesen, W. V. (1976). Measuring facial movement environmental psychology and nonverbal behavior. Environmental Psychology and Nonverbal Behavior, 1, 56–75.
- Felmingham, K. L., Williams, L. M., Kemp, A. H., Liddell, B., Falconer, E., Peduto, A., & Bryant, R. (2010). Neural responses to masked fear faces: Sex differences and trauma exposure in posttraumatic stress disorder. Journal of Abnormal Psychology, 119, 241–247.
- First, M. B., Frances, A. J., Pincus, H. A., Vettorello, N., & Davis, W. W. (1994). DSM-IV in progress. Changes in substance-related, schizophrenic, and other primarily adult disorders. Hospital & Community Psychiatry, 45, 18–20.
- Flanagan, J. C., Baker, N. L., McRae, A. L., Brady, K. T., & Moran‐Santa Maria, M. (2015). Effects of adverse childhood experiences on the association between intranasal Oxytocin and social stress reactivity among individuals with cocaine dependence. Psychiatry Research, 229, 94–100.
- Flanagan, J. C., Fischer, M. S., Nietert, P. J., Back, S. E., Moran-Santa Maria, M. M., Snead, A., & Brady, K. T. (2018a). Effects of Oxytocin on cortisol reactivity and conflict resolution behaviors among couples with substance misuse. Psychiatry Research, 260, 346–352.
- Flanagan, J. C., Hand, A., Jarnecke, A. M., Moran-Santa Maria, M. M., Brady, K. T., & Joseph, J. (2018b). Effects of Oxytocin on working memory and executive control system connectivity in posttraumatic stress disorder. Experimental and Clinical Psychopharmacology, 26, 391–402.
- Flanagan, J. C., Sippel, L. M., Wahlquist, A., Moran-Santa Maria, M. M., & Back, S. E. (2017). Augmenting Prolonged Exposure Therapy for PTSD with intranasal Oxytocin: A randomized, placebo-controlled pilot trial. Journal of Psychiatric Research, 98, 64–69.
- Foa, E. B., Cashman, L., Jaycox, L., & Perry, K. (1997). The validation of a self-report measure of posttraumatic stress disorder: The posttraumatic diagnostic scale. Psychological Assessment, 9, 445–451.
- Fonzo, G. A., Goodkind, M. S., Oathes, D. J., Zaiko, Y. V., Harvey, M., Peng, K. K., … Etkin, A. (2017). PTSD psychotherapy outcome predicted by brain activation during emotional reactivity and regulation. American Journal of Psychiatry, 174, 1163–1174.
- Fonzo, G. A., Huemer, J., & Etkin, A. (2016). History of childhood maltreatment augments dorsolateral prefrontal processing of emotional valence in PTSD. Journal of Psychiatric Research, 74, 45–54.
- Frijling, J. L., van Zuiden, M., Koch, S., Nawijn, L., Veltman, D. J., & Olff, M. (2016). Intranasal oxytocin affects amygdala functional connectivity after trauma script-driven imagery in distressed recently trauma-exposed individuals. Neuropsychopharmacology, 41, 1286–1296.
- Geuze, E., Vermetten, E., Ruf, M., de Kloet, C. S., & Westenberg, H. G. (2008). Neural correlates of associative learning and memory in veterans with posttraumatic stress disorder. Journal of Psychiatric Research, 42, 659–669.
- Goodwin, R. D., & Stein, M. B. (2004). Association between childhood trauma and physical disorders among adults in the United States. Psychological Medicine, 34, 509–520.
- Grant, B. F., Goldstein, R. B., Saha, T. D., Chou, S. P., Jung, J., Zhang, H., … Hasin, D. S. (2015a). Epidemiology of DSM-5 alcohol use disorder: Results from the national epidemiologic survey on alcohol and related conditions III. JAMA Psychiatry, 72, 757–766.
- Grant, M. M., Wood, K., Sreenivasan, K., Wheelock, M., White, D., Thomas, J., … Deshpande, G. (2015b). Influence of early life stress on intra- and extra-amygdaloid causal connectivity. Neuropsychopharmacology, 40, 1782–1793.
- Green, J. G., McLaughlin, K. A., Berglund, P. A., Gruber, M. J., Sampson, N. A., Zaslavsky, A. M., & Kessler, R. C. (2010). Childhood adversities and adult psychiatric disorders in the national comorbidity survey replication I: Associations with first onset of DSM-IV disorders. Archives of General Psychiatry, 67, 113–123.
- Hayes, J. P., Hayes, S. M., & Mikedis, A. M. (2012). Quantitative meta-analysis of neural activity in posttraumatic stress disorder. Biology of Mood & Anxiety Disorders, 2, 2–9.
- Heim, C., Shugart, M., Craighead, W. E., & Nemeroff, C. B. (2010). Neurobiological and psychiatric consequences of child abuse and neglect. Developmental Psychobiology, 52, 671–690.
- Hellmuth, J. C., Jaquier, V., Young-Wolff, K. C., & Sullivan, T. P. (2013). Posttraumatic stress disorder symptom clusters, alcohol misuse, and women‘s use of intimate partner violence. Journal of Traumatic Stress, 26, 451–458.
- Helpman, L., Marin, M.-F., Papini, S., Zhu, X., Sullivan, G. M., Schneier, F., … Neria, Y. (2016). Neural changes in extinction recall following prolonged exposure treatment for PTSD: A longitudinal fMRI study. Neuroimage: Clinical, 12, 715–723.
- Hendriks, L., De Kleine, R. A., Broekman, T. G., Hendriks, G., & Van Minnen, A. (2018). Intensive prolonged exposure therapy for chronic PTSD patients following multiple trauma and multiple treatment attempts European. Journal of Psychotraumatology, 9, 1–14.
- Hurlemann, R., Patin, A., Onur, O. A., Cohen, M. X., Baumgartner, T., Metzler, S., … Kendrick, K. M. (2010). Oxytocin enhances amygdala-dependent, socially reinforced learning and emotional empathy in humans. Journal of Neuroscience, 30, 4999–5007.
- Jakob, J. M., Lamp, K., Rauch, S. A., Smith, E. R., & Buchholz, K. R. (2017). The impact of trauma type or number of traumatic events on PTSD diagnosis and symptom severity in treatment seeking veterans. The Journal of Nervous and Mental Disease, 205, 83–86.
- Kaufman, J., Plotsky, P. M., Nemeroff, C. B., & Charney, D. S. (2000). Effects of early adverse experiences on brain structure and function: Clinical implications. Biological Psychiatry, 48, 778–790.
- Kelleher, I., Keeley, H., Corcoran, P., Ramsay, H., Wasserman, C., Carli, V., … Cannon, M. (2013). Childhood trauma and psychosis in a prospective cohort study: Cause, effect, and directionality. American Journal of Psychiatry, 170, 734–741.
- Kessler, R. C., Petukhova, M., Sampson, N. A., Zaslavsky, A. M., & Wittchen, H. U. (2012). Twelve-month and lifetime prevalence and lifetime morbid risk of anxiety and mood disorders in the Unites States. International Journal of Methods in Psychiatric Research, 21, 169–184.
- Kessler, R. C., Sonnega, A., Bromet, E., Hughes, M., & Nelson, C. B. (1995). Posttraumatic stress disorder in the national comorbidity survey. Archives of General Psychiatry, 52, 1048–1060.
- Kirsch, P., Esslinger, C., Chen, Q., Mier, D., Lis, S., Siddhanti, S., … Meyer-Lindenberg, A. (2005). Oxytocin modulates neural circuitry for social cognition and fear in humans. The Journal of Neuroscience: the Official Journal of the Society for Neuroscience, 25, 11489–11493.
- Koch, S., van Zuiden, M., Nawijn, L., Frijling, J. L., Veltman, D. J., & Olff, M. (2014). Intranasal oxytocin as strategy for medication-enhanced psychotherapy of PTSD: Salience processing and fear inhibition processes. Psychoneuroendocrinology, 40, 242–256.
- Koch, S., van Zuiden, M., Nawijn, L., Frijling, J. L., Veltman, D. J., & Olff, M. (2016a). Intranasal oxytocin normalizes amygdala functional connectivity in posttraumatic stress disorder. Neuropsychopharmacology, 41, 2041–2051.
- Koch, S. B., van Zuiden, M., Nawijn, L., Frijling, J. L., Veltman, D. J., & Olff, M. (2016b). Intranasal oxytocin administration dampens amygdala reactivity towards emotional faces in male and female PTSD patients. Neuropsychopharmacology, 41, 1495–1504.
- Koenen, K. C., Moffitt, T. E., Poulton, R., Martin, J., & Caspi, A. (2007). Early childhood factors associated with the development of post-traumatic stress disorder: Results from a longitudinal birth cohort. Psychological Medicine, 37, 181–192.
- Kouider, S., & Dehaene, S. (2007). Levels of processing during non-conscious perception: A critical review of visual masking. Philosophical Transactions of the Royal Society of London B: Biological Sciences, 362, 857–875.
- Kumar, J., Völlm, B., & Palaniyappan, L. (2015). Oxytocin affects the connectivity of the precuneus and the amygdala: A randomized, double-blinded, placebo-controlled neuroimaging trial. International Journal of Neuropsychopharmacology, 18, 1–7.
- Labuschagne, I., Phan, K. L., Wood, A., Angstadt, M., Chua, P., Heinrichs, M., … Nathan, P. J. (2010). Oxytocin attenuates amygdala reactivity to fear in generalized social anxiety disorder. Neuropsychopharmacology, 35, 2403–2413.
- Langner, O., Dotsch, R., Bijlstra, G., Wigboldus, D., Hawk, S. T., & van Knippenberg, A. (2010). Presentation and validation of the radboud faces database. Cognition & Emotion, 24, 1377–1388.
- Laugharne, J., Kullack, C., Lee, C. W., McGuire, T., Brockman, S., Drummond, P. D., & Starkstein, S. (2016). Amygdala volumetric change following psychotherapy for posttraumatic stress disorder. The Journal of Neuropsychiatry and Clinical Neurosciences, 28, 312–318.
- Luo, L., Becker, B., Geng, Y., Zhao, Z., Gao, S., Zhao, W., … Kendrick, K. M. (2017). Sex-dependent neural effect of oxytocin during subliminal processing of negative emotion faces. NeuroImage, 162, 127–137.
- Marusak, H. A., Martin, K. R., Etkin, A., & Thomason, M. E. (2015). Childhood trauma exposure disrupts the automatic regulation of emotional processing. Neuropsychopharmacology, 40, 1250–1258.
- Milad, M. R., Pitman, R. K., Ellis, C. B., Gold, A. L., Shin, L. M., Lasko, N. B., … Rauch, S. L. (2009). Neurobiological basis of failure to recall extinction memory in posttraumatic stress disorder. Biological Psychiatry, 66, 1075–1082.
- Nanni, V., Uher, R., & Danese, A. (2012). Childhood maltreatment predicts unfavorable course of illness and treatment outcome in depression. A Meta-Analysis American Journal of Psychiatry, 169, 141–151.
- Nawijn, L., van Zuiden, M., Koch, S., Frijling, J. L., Veltman, D. J., & Olff, M. (2016). Intranasal oxytocin enhances neural processing of monetary reward and loss in post-traumatic stress disorder and traumatized controls. Psychoneuroendocrinology, 66, 228–237.
- Norrholm, S. D., Jovanovic, T., Gerardi, M., Breazeale, K. G., Price, M., Davis, M., … Rothbaum, B. O. (2016). Baseline psychophysiological and cortisol reactivity as a predictor of PTSD treatment outcome in virtual reality exposure therapy. Behaviour Research and Therapy, 82, 28–37.
- Olff, M., Langeland, W., Witteveen, A., & Denys, D. (2010). A psychobiological rationale for oxytocin in the treatment of posttraumatic stress disorder. CNS Spectrums, 15, 522–530.
- Palgi, S., Klein, E., & Shamay-Tsoory, S. G. (2016). Oxytocin improves compassion toward women among patients with PTSD. Psychoneuroendocrinology, 64, 143–149.
- Petrovic, P., Kalisch, R., Singer, T., & Dolan, R. J. (2008). Oxytocin attenuates affective evaluations of conditioned faces and amygdala activity. Journal of Neuroscience, 28, 6607–6615.
- Pfundmair, M., Reinelt, A., DeWall, C. N., & Feldmann, L. (2018). Oxytocin strengthens the link between provocation and aggression among low anxiety people. Psychoneuroendocrinology, 93, 124–132.
- Phelps, E. A., O‘Connor, K. J., Gatenby, J. C., Gore, J. C., Grillon, C., & Davis, M. (2001). Activation of the left amygdala to a cognitive representation of fear. Nature Neuroscience, 4, 437–441.
- Pitman, R. K., Rasmusson, A. M., Koenen, K. C., Shin, L. M., Orr, S. P., Gilbertson, M. W., … Liberzon, I. (2012). Biological studies of post-traumatic stress disorder. Nature Reviews Neuroscience, 13, 769–787.
- Rabellino, D., Densmore, M., Frewen, P. A., Théberge, J., & Lanius, R. A. (2016). The innate alarm circuit in post-traumatic stress disorder: Conscious and subconscious processing of fear-and trauma-related cues. Psychiatry Research: Neuroimaging, 248, 142–150.
- Rauch, S. L., Shin, L. M., & Phelps, E. A. (2006). Neurocircuitry models of posttraumatic stress disorder and extinction: Human neuroimaging research—Past, present, and future. Biological Psychiatry, 60, 376–382.
- Rauch, S. L., Whalen, P. J., Shin, L. M., McInerney, S. C., Macklin, M. L., Lasko, N. B., … Pitman, R. K. (2000a). Exaggerated amygdala response to masked facial stimuli in posttraumatic stress disorder: A functional MRI study. Biological Psychiatry, 47, 769–776.
- Rauch, S. L., Whalen, P. J., Shin, L. M., McInerney, S. C., Macklin, M. L., Lasko, N. B., … Pitman, R. K. (2000b). Exaggerated amygdala response to masked facial stimuli in posttraumatic stress disorder: A functional MRI study. Biological Psychiatry, 47, 769–776.
- Rilling, J. K., Demarco, A. C., Hackett, P. D., Chen, X., Gautam, P., Stair, S., … Pagnoni, G. (2014). Sex differences in the neural and behavioral response to intranasal oxytocin and vasopressin during human social interaction. Psychoneuroendocrinology, 39, 237–248.
- Ring, R. H., Malberg, J. E., Potestio, L., Ping, J., Boikess, S., Luo, B., … Rosenzweig-Lipson, S. (2006). Anxiolytic-like activity of oxytocin in male mice: Behavioral and autonomic evidence, therapeutic implications. Psychopharmacology, 185, 218–225.
- Russell, J., Maguire, S., Hunt, G. E., Kesby, A., Suraev, A., Stuart, J., … McGregor, I. S. (2018). Intranasal oxytocin in the treatment of anorexia nervosa: Randomized controlled trial during re-feeding. Psychoneuroendocrinology, 87, 83–92.
- Sheehan, D. V., Lecrubier, Y., Sheehan, K. H., Amorim, P., Janavs, J., Weiller, E., ... Dunbar, G. C. (1998). The Mini-International Neuropsychiatric Interview (M.I.N.I.): The development and validation of a structured diagnostic psychiatric interview for DSM-IV and ICD-10. The Journal of Clinical Psychiatry, quiz 34–57, 59(Suppl 20), 22–33.
- Shin, L. M., Rauch, S. L., & Pitman, R. K. (2006). Amygdala, medial prefrontal cortex, and hippocampal function in PTSD annals of the New York. Academy of Sciences, 1071, 67–79.
- Shin, L. M., Wright, C. I., Cannistraro, P. A., Wedig, M. M., McMullin, K., Martis, B., … Rauch, S. L. (2005). A functional magnetic resonance imaging study of amygdala and medial prefrontal cortex responses to overtly presented fearful faces in posttraumatic stress disorder. Archives of General Psychiatry, 62, 273–281.
- Sippel, L. M., King, C., Wahlquist, A., & Flanagan, J. C. (in preparation) an examination of endogenous Oxytocin in a pilot randomized clinical trial of Oxytocin-enhanced psychotherapy for PTSD.
- Spengler, F. B., Schultz, J., Scheele, D., Essel, M., Maier, W., Heinrichs, M., & Hurlemann, R. (2017). Kinetics and dose dependency of intranasal oxytocin effects on amygdala reactivity. Biological Psychiatry, 82(12), 885–894.
- Spielberger, C. D., Gorsuch, R. L., Lushene, R., Vagg, P. R., & Jacobs, G. A. (1983). Manual for the state-trait anxiety inventory. Palo Alto, CA: Consulting Psychologists Press.
- Sripada, R. K., King, A. P., Garfinkel, S. N., Wang, X., Sripada, C. S., Welsh, R. C., & Liberzon, I. (2012). Altered resting-state amygdala functional connectivity in men with posttraumatic stress disorder. Journal of Psychiatry and Neuroscience, 37, 241.
- Stevens, J. S., Ely, T. D., Sawamura, T., Guzman, D., Bradley, B., Ressler, K. J., & Jovanovic, T. (2016). Childhood maltreatment predicts reduced inhibition‐related activity in the rostral anterior cingulate in PTSD, but not trauma‐exposed controls. Depression and Anxiety, 33, 614–622.
- Teicher, M. H., Andersen, S. L., Polcari, A., Anderson, C. M., & Navalta, C. P. (2002). Developmental neurobiology of childhood stress and trauma. The Psychiatric Clinics of North America, 25, 397–426, vii-viii.
- Tottenham, N., Tanaka, J. W., Leon, A. C., McCarry, T., Nurse, M., Hare, T. A., … Nelson, C. (2009). The NimStim set of facial expressions: Judgments from untrained research participants. Psychiatry Research, 168, 242–249.
- Tunnard, C., Rane, L. J., Wooderson, S. C., Markopoulou, K., Poon, L., Fekadu, ... Cleare, A. J. (2014). The impact of childhood adversity on suicidality and clinical course in treatment-resistant depression. Journal of Affective Disorders, 152, 122–130.
- van Zuiden, M., Frijling, J. L., Nawijn, L., Koch, S. B. J., Goslings, J. C., Luitse, J. S., … Olff, M. (2016). Intranasal oxytocin to prevent PTSD symptoms: A randomized controlled trial in emergency department patients. Biological Psychiatry, 81, 1030–1040.
- Veer, I. M., Oei, N., van Buchem, M. A., Spinhoven, P., Elzinga, B. M., & Rombouts, S. (2015). Evidence for smaller right amygdala volumes in posttraumatic stress disorder following childhood trauma. Psychiatry Research: Neuroimaging, 233, 436–442.
- Whalen, P. J., Rauch, S. L., Etcoff, N. L., McInerney, S. C., Lee, M. B., & Jenike, M. A. (1998). Masked presentations of emotional facial expressions modulate amygdala activity without explicit knowledge. Journal of Neuroscience, 18, 411–418.
- Witt, D. M., Winslow, J. T., & Insel, T. R. (1992). Enhanced social interactions in rats following chronic, centrally infused oxytocin. Pharmacology Biochemistry and Behavior, 43, 855–861.
- Woodward, S. H., Neylan, T. C., Mellman, T. A., & Ross, R. J. (2006). Distinguishing current from remitted posttraumatic stress disorder. Archives of General Psychiatry, 63, 940–941.
- Woolrich, M. W. (2008). Robust group analysis using outlier inference. Neuroimage, 41, 286–301.
- Woolrich, M. W., Behrens, T. E., Beckmann, C. F., Jenkinson, M., & Smith, S. M. (2004). Multilevel linear modelling for FMRI group analysis using Bayesian inference. Neuroimage, 21, 1732–1747.
- Woolrich, M. W., Ripley, B. D., Brady, M., & Smith, S. M. (2001). Temporal autocorrelation in univariate linear modeling of FMRI data. Neuroimage, 14, 1370–1386.
- Worsley, K. J. (2001). Statistical analysis of activation images. In P. Jezzard, P. M. Matthews, & S. M. Smith (Eds.), Functional MRI: An introduction to methods. New York, NY: OUP.
- Zuj, D. V., Palmer, M. A., Lommen, M. J., & Felmingham, K. L. (2016). The centrality of fear extinction in linking risk factors to PTSD: A narrative review. Neuroscience & Biobehavioral Reviews, 69, 15–35.