ABSTRACT
Metformin has beneficial effects on several age-related diseases (e.g., diabetes, obesity, cancer) and extends lifespan in nematodes and mice. Acarbose, an FDA-approved agent for treating type 2 diabetes, prevents breakdown of complex carbohydrates. Both compounds have been suggested as potential anti-aging interventions and acarbose has been shown to extend mouse longevity by the Intervention Testing Program (ITP). One potential next step is to assess the effect of these interventions on healthspan and lifespan in non-human primates. The common marmoset (Callithrix jacchus) is a small new world monkey with a relatively short life span and small size, both valuable for the translation potential of this nonhuman primate species for the study of aging and chronic disease. However, the dosing and assessment of potential side effects of either metformin or acarbose in this species have yet to be assessed. This study evaluated the pharmacokinetics of two dosage levels each of metformin or acarbose (given separately) in two small groups of young marmosets (n = 5/group) treated for 24 h to define the pharmacokinetics of each drug. The ability to rapidly and reliably dose socially housed marmosets with an oral form of acarbose or metformin that is well tolerated indicates that this species is a reliable model for testing acarbose and metformin in a safe and efficient way in a long-term intervention.
Introduction
The aging process drives increasing susceptibility to death and disease and is the single greatest risk factor for numerous pathological outcomes including metabolic disease, neurodegeneration, cancer, etc. With evidence that the aging process can be delayed in laboratory animals, including invertebrates and rodents, there is increasing hope that clinical applications to improve, or prevent, declines in health among aging human populations can be developed. Among potential approaches, pharmaceutical interventions are likely to have the easiest implementation and greatest compliance clinically.
Over the last few decades, several candidate pharmaceuticals that extend longevity in mice have been discovered, many from the Interventions Testing Program (ITP) supported by the National Institute on Aging. While rapamycin, an inhibitor of mTOR signaling has been one of the greatest success stories among these candidates, there are a growing number of other compounds with a positive impact on murine lifespan. Acarbose is an α-glucosidase inhibitor that delays uptake of complex carbohydrates in the gut and is used to treat type 2 diabetes [Citation1]. In mice, acarbose was reported to significantly extend the lifespan of mice when administered with early or late in life [Citation2,Citation3]. Similarly, metformin is part of the first-line treatment for type 2 diabetes mellitus and reduces hyperglycemia primarily by inhibiting hepatic gluconeogenesis [Citation4,Citation5]. While metformin has been reported to extend lifespan of male mice in at least one study, others have failed to show a significant effect of this drug on mouse lifespan [Citation2,Citation6]. However, there is strong support for testing the effects of metformin on human aging due in part to its relative safety and widespread use [Citation7,Citation8].
With a goal towards translation, we have used a non-human primate (NHP) species, the common marmoset (Callithrix jacchus), to test whether those drugs that extend rodent lifespan may have beneficial effects in a species more closely related to humans. Transitioning findings directly from rodents to humans have been in many cases challenging due to basic evolutionary differences between these species and testing interventions in NHP may help bridge this divide. The common marmoset, in particular, has qualities including small size and relatively short average lifespan for a NHP that make it in many ways particularly well suited for aging research [Citation9]. In this regard, we have successfully transitioned rapamycin towards testing a marmoset longevity study [Citation10]. One of the first challenges in designing such a study is characterizing the basic pharmacokinetics in this species to identify dosing regiments likely to be effective [Citation10,Citation11]. Here we define the basic pharmacokinetics of acarbose and, separately, metformin in a colony of laboratory marmosets. This represents a first step in testing the viability of these drugs for potential long-term intervention studies on healthy aging in the marmoset.
Materials and methods
Animals, drug delivery, and training
All marmosets used in this research were housed at the Barshop Institute for Longevity and Aging Studies at the UT Health San Antonio (UTHSA). The Institutional Animal Care and Use Committee (IACUC) of UTHSA is responsible for monitoring housing and animal condition regularly to ensure all guidelines are met for the safety and health of the animals. This research was reviewed and approved by the UTHSA IACUC and experiments were conducted in compliance with the US Public Health Service’s Policy on Humane Care and Use of Laboratory Animals and the Guide for the Care and Use of Laboratory Animals and adhered to the American Society of Primatologists (ASP) principles for the ethical treatment of non-human primates.
The subjects for this pilot study were common marmoset monkeys (Callithrix jacchus) housed at the South Texas Center for Biology in Medicine (STCBM) at UTHSA. The entire study used 10 marmosets (); the same group of animals was used first for acarbose treatment and then for metformin treatment with a 2-week washout period in between. Animals were randomly assigned to one of 2 different doses for each drug. Acarbose (300 µg or 600 µg) or metformin (2 mg or 3 mg) was delivered orally for assessment of single-dose pharmacokinetics. The dosage regimens were based on therapeutic doses used in humans and scaled down for the smaller body size (~400 g). Drugs were mixed well with EnsureTM and then delivered orally in a syringe. The drug were administered in the home cages of the animals. Marmoset training to receive oral dosing by syringe has been described previously [Citation10]. EnsureTM was used because it is a food item with which the marmosets are familiar as they receive it regularly for dietary enrichment. It is generally well received by the animals. EnsureTM offered the advantage of dosing each animal individually while they are group housed, using a syringe delivery, and thus the intake of each daily dose could be accounted for. While enrolled in the study, each animal received three diet choices daily provided ad libitum: Harlan Teklad purified marmoset diet (TD99468), Mazuri Callitrichid gel diet (5MI5) and ZuPreem.
Table 1. Biological characteristics of study subjects.
The animals were monitored for signs of gastrointestinal side effects – vomiting, diarrhea, lack of appetite, and postures indicative of abdominal pain – at the time of dosing resulting in three observations per day for acarbose and two times per day for metformin. Animals were monitored for a period of 2 weeks after each dosing.
Animals were weighed prior to each study by placing a scale in the cage. Technical staff monitored the animals for any gastrointestinal distress on a daily basis. During these experiments, the treated animals did not show any displays of diarrhea, vomiting, or anorexia over a 24-h period.
Animals were given a single administration to evaluate the pharmacokinetics of the drugs. For each drug/dose combination, blood was drawn from the treated animals and tested by HPLC/MS/MS to determine whether therapeutic blood levels (~1–2 µg/mL) are achieved and to detect known toxic levels (>50 µg/mL). Animals were bled repeatedly on the first day of dosing at 0/15/30/60/120/240/480/1440 min, in order to establish pharmacokinetic parameters.
The maximum limit of daily volume of blood collection was honored with this procedure. Non-sedated animals were bled while restrained in a device designed especially for this purpose with this species. Animals remained in the restraint throughout the first 30 min following dosing on day 1. For subsequent collections, the marmosets were again placed in the same device. This routine has been employed regularly in our lab for blood collections for testing such as PK and oral glucose tolerance testing with no untoward effects.
Measurement of acarbose using HPLC-tandem MS
Acarbose was obtained from Sigma Chemical Company (St. Louis, MO). HPLC grade methanol was purchased from Fisher (Fair Lawn, NJ). All other reagents were purchased from Sigma Chemical Company (St. Louis, MO). Milli-Q water was used for preparation of all solutions.
The HPLC system consisted of a Shimadzu SIL 20A HT autosampler, LC-20AD pumps [Citation2], and an AB Sciex API 3200 tandem mass spectrometer with turbo ion spray. The LC analytical column was a Grace Alltima C18 (4.6 x 150 mm, 5 micron) purchased from Alltech (Deerfield, IL) and was maintained at 25°C during the chromatographic runs using a Shimadzu CT-20A column oven. Mobile phase A contained 10 mM ammonium formate and 0.1% formic acid dissolved in HPLC grade methanol. Mobil phase B contained 10 mM ammonium formate and 0.1% formic acid dissolved in 90% HPLC grade methanol. The flow rate of the mobile phase was 0.4 ml/min. Acarbose was eluted with a gradient. The initial mobile phase was 100% B and at 0.10 min after injection was ramped to 100% A. From 4.0 min to 5.0 min the mobile phase was maintained at 100% A and at 5.1 min was switched immediately back to 100% B and ran for 4.9 min to equilibrate the column before the next injection. The acarbose transition was detected at 646.03 Da (precursor ion) and the daughter ion was detected at 304.20 Da.
Acarbose super stock solutions were prepared in methanol at a concentration of 1 mg/ml and stored in aliquots at −80°C. A working stock solution was prepared each day from the super stock solutions at a concentration of 10 μg/ml and used to spike the calibrators. Calibrator samples were prepared daily by spiking serum and serially diluting to achieve final concentrations of 0, 2.5, 5.0, 10.0, 20.0 ng/ml.
To quantify acarbose concentration in plasma, 0.05 mL of calibrator and unknown serum samples were mixed with 200 ul of a solution containing 0.1% formic acid and 10 mM ammonium formate dissolved in 100% HPLC grade methanol. The samples were vortexed vigorously for 2 min, and then centrifuged at 13,000 g for 5 min at 23°C (subsequent centrifugations were performed under the same conditions). Supernatants were transferred to 1.5 ml microfilterfuge tubes and spun at 13,000 g for 1 min and then 20 µL of the final extracts were injected into the LC/MS/MS. The peak area response of acarbose for each unknown sample was compared against a linear regression of calibrator response peak areas to quantify acarbose. The concentration of acarbose was expressed as ng/mL plasma.
To quantify acarbose concentration in feces, 20 mg of feces was collected in 16 × 101 mm polypropylene tubes for calibrators and unknowns. Calibrator samples were spiked at concentrations of 0, 0.5, 1.0, 5.0, and 10.0 µg acarbose/mg feces. Four mL of mobile phase B was then added to the tubes and the tubes were vortexed and sonicated on ice for four bursts of 5 s each with 5 s between each sonication. The tubes were then centrifuged at 4700 rpm in a Beckman Coulter Allegra X12 centrifuge for 10 min. Three hundred microliters of the supernatants were then transferred to microfilterfuge tubes and spun at 13,000 g for 1 min. The filtered extract was then transferred to autosampler vials and 10 µL was injected into the LCMS system. The peak area response of acarbose for each unknown sample was compared against a linear regression of calibrator response peak areas to quantify acarbose. The concentration of acarbose was expressed as µg/mg feces.
Measurement of metformin (MET) in whole blood or plasma using HPLC-tandem MS
MET and phenformin (PHEN; internal standard) were obtained from Sigma Chemical Company (St. Louis, MO). HPLC grade methanol and acetonitrile were purchased from Fisher (Fair Lawn, NJ). All other reagents were purchased from Sigma Chemical Company (St. Louis, MO). Milli-Q water was used for preparation of all solutions.
The HPLC system consisted of a Shimadzu SCL-10A Controller, LC-10AD pump with a FCV-10AL mixing chamber, SIL-10AD autosampler, and an AB Sciex API 3200 tandem mass spectrometer with turbo ion spray. The LC analytical column was a Grace Alltima C18 (4.6 x 150 mm, 5 micron) purchased from Alltech (Deerfield, IL) and was maintained at 60°C during the chromatographic runs using a Shimadzu CTO-10A column oven. The mobile phase contained 0.1% formic acid dissolved in 50% HPLC grade acetonitrile. The flow rate of the mobile phase was 0.5 ml/min. The MET transition was detected at 130.0 Da (precursor ion) and the daughter ion was detected at 71.0 Da. PHEN was detected at 206.2 Da and the daughter ion was 60.1 Da.
MET and PHEN super stock solutions were prepared in water at a concentration of 1 mg/ml and stored in aliquots at −80°C. A working stock solution was prepared each day from the super stock solutions at a concentration of 10 μg/ml and used to spike the calibrators. Calibrator samples were prepared daily by spiking whole blood or plasma to achieve final concentrations of 0, 15.6, 62.5, 250, 1000, 4000 ng/ml.
MET was quantified in uncoagulated blood or plasma (EDTA). Briefly, 0.1 mL of the calibrator and unknown whole blood or plasma samples were mixed with 5 µL of 10 µg/mL PHEN (internal standard), and 1.5 mL of acetonitrile. The samples were vortexed vigorously for 2 min, and then centrifuged at 4600 g for 5 min at 23°C. Supernatants were transferred to 10 × 75 mm glass tubes and dried under a stream of nitrogen. The residues were then redissolved in 150 µl mobile phase, transferred to 1.5 ml microfilterfuge tubes and spun at 13,000 g for 1 min. The final extracts were transferred to autosampler vials and 25 µL of the were injected into the LC/MS/MS. The ratio of the peak area of MET to that of the internal standard PHEN (response ratio) for each unknown sample was compared against a linear regression of calibrator response ratios to quantify MET. The concentration of MET was expressed as ng/mL whole blood or plasma.
Results
Pharmacokinetics of acarbose in marmoset plasma and feces
Acarbose is not well absorbed from the intestinal tract. Nevertheless, several studies have reported detectable plasma levels of acarbose after oral doses of the drug. A single administration of acarbose at either of two doses (300 µg and 600 µg) was administered to marmosets to evaluate the pharmacokinetics of the drug in this species. shows the plasma acarbose concentration for the 24 h following the initial dose. All animals achieved our goal of monitoring acarbose levels in blood, at two doses. We report here variation among animals within each dose group in their concentration of acarbose in plasma after treatment. That is, some animals show much higher levels of plasma acarbose than others within the same treatment group. The mean time to achieve maximum acarbose levels (Tmax) in the plasma was 2 h after either dose was administered for all the subjects. Maximum acarbose concentrations in the plasma (Cmax) ranged from 5.28 to 68.1 ng/mL in subjects receiving 300 µg dose and from 11.5 to 36.8 ng/mL in subjects receiving 600 µg dose. Some, but not all, animals still showed detectable concentrations of plasma acarbose after 24 h following dosing.
Figure 1. Acarbose (300 or 600 µg) doses were administered to marmosets in Ensure liquid diet. Single doses produced Acarbose levels in blood. Top panels show the concentration of ACA (ng/ml) per dose/animal. Bottom panels show the average concentration of ACA ±SD per dose.
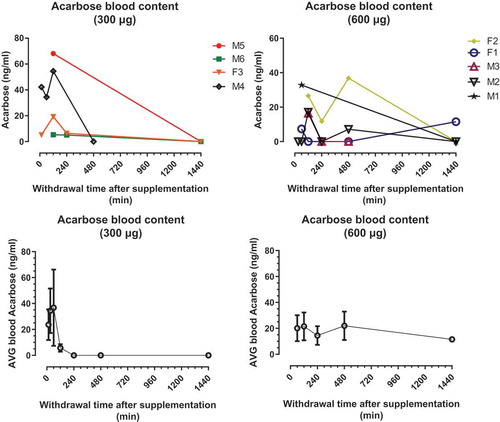
Fecal samples were collected opportunistically during 72 h following initial dosing of all marmosets. shows the fecal acarbose concentration for the 24 h following the initial dose. The concentrations of acarbose in stool in each interval of collection were variable among animals within each dose group over the 72-h period after initial dose was administered. Maximum acarbose concentrations in the fecal pellets (Cmax) ranged from 0.0108 to 0.692 µg/mg in subjects receiving 300 µg dose and from 1.01 to 2.37 µg/mg in subjects receiving 600 µg dose. Some, but not all, animals still showed detectable concentrations of plasma acarbose after 72 h following dosing.
Pharmacokinetics of metformin in marmoset plasma
Similar to the acarbose study above, a single administration of metformin at either of two dose (2 or 3 mg) was used to assess the pharmacokinetics of this drug in marmosets. shows plasma metformin concentrations for marmosets in each of the dosing groups. All animals showed elevated concentrations of metformin in the blood following administration with some degree of variation among animals. The mean time to maximum plasma metformin concentrations (Tmax) was 2 h after either dose. Maximum metformin concentrations (Cmax) in plasma ranged from 180.5 to 353 ng/mL for the 2 mg dose and from 388.5 to 518.3 ng/mL for the 3 mg dose. By 24 h the average plasma concentration of 2 mg dose group was near 0 while that of the 3 mg dose group was still relatively high.
Discussion
The main outcome of this study is the determination of basic pharmacokinetics in the common marmoset of two pharmaceuticals, acarbose and metformin, of interest to delay mammalian aging. Clinically, metformin and acarbose are both used for treating diseases of metabolic function including diabetes and hyperglycemia. However, there is growing evidence that these drugs may have effects beyond their action on glucose metabolism. For example, metformin use has been reported to reduce the risk for certain forms of cancer [Citation12,Citation13]. Metformin use has also been associated with cognitive improvement in patients with particular forms of dementia [Citation14]. Acarbose use is associated with cardiovascular benefits in diabetic patients [Citation15,Citation16] and has been shown to be protective against atherosclerosis and myocardial infact in laboratory animal models [Citation17,Citation18]. Acarbose has been shown to prevent liver injury in diabetic patients [Citation19]. Acarbose use has also been associated with significant changes in the makeup of the gut microbiota [Citation20,Citation21]. It would be of interest to follow long term how these pleiotropic effects may drive the outcomes of intervention to promote healthy aging.
There is growing evidence of sex-specificity in the outcomes of both acarbose and metformin, particularly in the degree of effect. While acarbose extends lifespan in both sexes of mice, males showed 22% longer lifespan compared to only 5% in females [Citation3]. Improved glucose tolerance associated with acarbose treatment in mice was also specific to males and driven by the presence of functional male gonads [Citation22]. Interestingly, this same report showed that females did not improve glucose tolerance in response to acarbose unless functional ovaries were removed. Similarly, changes in the metabolome and in hypothalamic inflammation associated with acarbose treatment have been reported as male-specific outcomes of this drug [Citation23,Citation24]. Though equivocal, metformin has been reported to extend male median lifespan only [Citation2]. However, we showed sex-specific effects of metformin on glucose metabolism in mice, with female responding in part due to higher blood concentrations of metformin at a single given dose [Citation25]. Understanding this relationship between drug interaction and genetic and hormonal sex will be important for future studies.
Of note, we report here that measurable quantities of acarbose make it to the blood stream even after a single dose of acarbose. While acarbose is thought to pass through the gut in a non-metabolized form, it has been reported that approximately 1–2% of the ingested acarbose is absorbed into circulation from the intestine [Citation26]. Even account for this very low absorption, we found significant (approximately 25–50 ng/mL) concentrations in the blood. Effects of acarbose outside of its α-glucosidase activity are unclear, although there is evidence that acarbose can slow proliferation and migration of smooth muscle cells in culture [Citation27]. In addition, acarbose has been shown to activate cellular respiration in cell culture [Citation28]. In line with this, acarbose has been shown to alter mitochondrial ATP-sensitive potassium channels and reduce oxidative stress in vivo suggesting direct effects on mitochondrial function or utilization of energetic substrates [Citation18,Citation29]. Similarly, metformin has been shown to directly affect mitochondrial function and energetic outcomes both in vitro and in vivo [Citation13,Citation30,Citation31]. How these outcomes might promote longevity is unclear, but these findings suggest a potential shared mechanism of action between these anti-aging compounds that might be exploited for further study.
As we have discussed elsewhere, comparative intervention studies using the common marmoset can make significant strides towards translation of studies in mice towards application in human populations [Citation9,Citation32]. Certainly, studies testing the effects of compounds like acarbose and metformin on marmoset lifespan would be informative, but such long-term studies should also include more inclusive assessments of health or healthspan [Citation33]. That is, it will be important to test whether acarbose or metformin (or even combination) will slow the physiological, functional and molecular changes associated with aging in mammalian species [Citation34–Citation40]
Acknowledgments
Animal care was provided by the Department of Lab Animal Research (LAR) at UTHSA. Aubrey Sills and Yuhong Liu performed research intervention in marmosets, collected data and prepared samples.
Disclosure statement
No potential conflict of interest was reported by the authors.
Additional information
Funding
References
- Rosak C, Mertes G. Critical evaluation of the role of acarbose in the treatment of diabetes: patient considerations. Diabetes Metab Syndr Obes. 2012;5:1–8. PubMed PMID: 23093911; PubMed Central PMCID: PMC3476372.
- Strong R, Miller RA, Antebi A, et al. Longer lifespan in male mice treated with a weakly estrogenic agonist, an antioxidant, an alpha-glucosidase inhibitor or a Nrf2-inducer. Aging Cell. 2016;15(5):872–884. PubMed PMID: 27312235.
- Harrison DE, Strong R, Allison DB, et al. Acarbose, 17-alpha-estradiol, and nordihydroguaiaretic acid extend mouse lifespan preferentially in males. Aging Cell. 2014;13(2):273–282. PubMed PMID: 24245565; PubMed Central PMCID: PMC3954939.
- Cusi K, DeFronzo RA. Metformin: a review of its metabolic effects. Diabetes Rev. 1998;6(2): 89–131. PubMed PMID: WOS:000077170200002.
- Hundal RS, Krssak M, Dufour S, et al. Mechanism by which metformin reduces glucose production in type 2 diabetes. Diabetes. 2000;49(12):2063–2069. PubMed PMID: WOS:000165573500013.
- Martin-Montalvo A, Mercken EM, Mitchell SJ, et al. Metformin improves healthspan and lifespan in mice. Nat Commun. 2013;4:2192. PubMed PMID: 23900241; PubMed Central PMCID: PMC3736576.
- Justice JN, Ferrucci L, Newman AB, et al. A framework for selection of blood-based biomarkers for geroscience-guided clinical trials: report from the TAME Biomarkers Workgroup. Geroscience. 2018. PubMed PMID: 30151729. DOI:10.1007/s11357-018-0042-y.
- Barzilai N, Crandall JP, Kritchevsky SB, et al. Metformin as a tool to target aging. Cell Metab. 2016;236:1060–1065. PubMed PMID: WOS:000378000600018.
- Tardif SD, Mansfield KG, Ratnam R, et al. The marmoset as a model of aging and age-related disease. Inst Lab Anim Res J. 2011;52(1):54–65.
- Tardif S, Ross C, Bergman P, et al. Testing efficacy of administration of the antiaging drug rapamycin in a nonhuman primate, the common marmoset. J Gerontol A Biol Sci Med Sci. 2015;70(5):577–587. PubMed PMID: 25038772; PubMed Central PMCID: PMC4400395.
- Lin JH. Applications and limitations of interspecies scaling and in vitro extrapolation in pharmacokinetics. Drug Metab Dispos. 1998;26(12): 1202–1212. PubMed PMID: WOS:000077689700010.
- Decensi A, Puntoni M, Goodwin P, et al. Metformin and cancer risk in diabetic patients: a systematic review and meta-analysis. Cancer Prev Res. 2010;3(11):1451–1461. PubMed PMID: 20947488.
- Zhang ZJ, Bi Y, Li S, et al. Reduced risk of lung cancer with metformin therapy in diabetic patients: a systematic review and meta-analysis. Am J Epidemiol. 2014;180(1):11–14. PubMed PMID: 24920786.
- Lin Y, Wang K, Ma C, et al. Evaluation of metformin on cognitive improvement in patients with non-dementia vascular cognitive impairment and abnormal glucose metabolism. Front Aging Neurosci. 2018;10:227. PubMed PMID: 30100873; PubMed Central PMCID: PMC6074058.
- Holman RR, Coleman RL, Chan JCN, et al. Effects of acarbose on cardiovascular and diabetes outcomes in patients with coronary heart disease and impaired glucose tolerance (ACE): a randomised, double-blind, placebo-controlled trial. Lancet Diabetes Endocrinol. 2017 Nov;5:877–886. PubMed PMID: 28917545.
- Hsu PF, Sung SH, Cheng HM, et al. Cardiovascular benefits of acarbose versus sulfonylureas in type 2 diabetic patients treated with metformin. J Clin Endocrinol Metab. 2018;103:3611–3619. PubMed PMID: 30113697.
- Chan KC, Yu MH, Lin MC, et al. Pleiotropic effects of acarbose on atherosclerosis development in rabbits are mediated via upregulating AMPK signals. Sci Rep. 2016;6:38642. PubMed PMID: 27924924; PubMed Central PMCID: PMC5141573.
- Minatoguchi S, Zhang Z, Bao N, et al. Acarbose reduces myocardial infarct size by preventing postprandial hyperglycemia and hydroxyl radical production and opening mitochondrial KATP channels in rabbits. J Cardiovasc Pharmacol. 2009;54(1):25–30. PubMed PMID: 19487955.
- Chao CT, Wang J, Huang JW, et al. Acarbose use and liver injury in diabetic patients with severe renal insufficiency and hepatic diseases: a propensity score-matched cohort study. Front Pharmacol. 2018;9. PubMed PMID: WOS:000441016700001. DOI:10.3389/Fphar.2018.00860
- Gu Y, Wang X, Li J, et al. Analyses of gut microbiota and plasma bile acids enable stratification of patients for antidiabetic treatment. Nat Commun. 2017;8(1):1785. PubMed PMID: 29176714; PubMed Central PMCID: PMC5702614.
- Zhang X, Fang Z, Zhang C, et al. Effects of acarbose on the gut microbiota of prediabetic patients: a randomized, double-blind, controlled crossover trial. Diabetes Therapy. 2017;8(2):293–307. PubMed PMID: 28130771; PubMed Central PMCID: PMC5380489.
- Garratt M, Bower B, Garcia GG, et al. Sex differences in lifespan extension with acarbose and 17-alpha estradiol: gonadal hormones underlie male-specific improvements in glucose tolerance and mTORC2 signaling. Aging Cell. 2017;166:1256–1266. PubMed PMID: 28834262; PubMed Central PMCID: PMC5676051.
- Gibbs VK, Brewer RA, Miyasaki ND, et al. Sex-dependent differences in liver and gut metabolomic profiles with acarbose and calorie restriction in C57BL/6 mice. J Gerontol A Biol Sci Med Sci. 2018;732:157–165. PubMed PMID: 28651373; PubMed Central PMCID: PMC5861978.
- Sadagurski M, Cady G, Miller RA. Anti-aging drugs reduce hypothalamic inflammation in a sex-specific manner. Aging Cell. 2017;164:652–660. PubMed PMID: 28544365; PubMed Central PMCID: PMC5506421.
- Weiss R, Fernandez E, Liu Y, et al. Metformin reduces glucose intolerance caused by rapamycin treatment in genetically heterogeneous female mice. Aging (Albany NY). 2018;10:386–401. PubMed PMID: 29579736.
- Ahr HJ, Boberg M, Krause HP, et al. Pharmacokinetics of acarbose .1. Absorption, concentration in plasma, metabolism and excretion after single administration of [C-14]acarbose to rats, dogs and man. Arzneimittel-Forsch. 1989;39-2(10):1254–1260. PubMed PMID: WOS:A1989AW02200017.
- Yu MH, Lin MC, Huang CN, et al. Acarbose inhibits the proliferation and migration of vascular smooth muscle cells via targeting Ras signaling. Vascul Pharmacol. 2018;103–105:8–15. PubMed PMID: 29432898.
- Sahdeo S, Tomilov A, Komachi K, et al. High-throughput screening of FDA-approved drugs using oxygen biosensor plates reveals secondary mitofunctional effects. Mitochondrion. 2014;17:116–125.
- Rosen P, Osmers A. Oxidative stress in young Zucker rats with impaired glucose tolerance is diminished by acarbose. Horm Metab Res. 2006;389:575–580. PubMed PMID: WOS:000241498400005.
- Chakraborty A, Chowdhury S, Bhattacharyya M. Effect of metformin on oxidative stress, nitrosative stress and inflammatory biomarkers in type 2 diabetes patients. Diabetes Res Clin Pract. 2011;931:56–62. PubMed PMID: 21146883.
- Andrzejewski S, Gravel SP, Pollak M, et al. Metformin directly acts on mitochondria to alter cellular bioenergetics. Cancer Metab. 2014;2:12. PubMed PMID: 25184038; PubMed Central PMCID: PMC4147388.
- Salmon AB. Moving toward ‘common’ use of the marmoset as a non-human primate aging model. Pathobiol Aging Age Relat Dis. 2016;6:32758. PubMed PMID: 27452489; PubMed Central PMCID: PMCPMC4958916.
- Kaeberlein M. How healthy is the healthspan concept? GeroScience. 2018;40:361–364.
- Lee HJ, Feliers D, Barnes JL, et al. Hydrogen sulfide ameliorates aging-associated changes in the kidney. GeroScience. 2018;40(2):163–176. PubMed PMID: 29717417; PubMed Central PMCID: PMC5964063.
- Masser DR, Hadad N, Porter H, et al. Analysis of DNA modifications in aging research. Geroscience. 2018;40(1):11–29. PubMed PMID: 29327208; PubMed Central PMCID: PMC5832665.
- An JY, Quarles EK, Mekvanich S, et al. Rapamycin treatment attenuates age-associated periodontitis in mice. GeroScience. 2017;39(4):457–463. PubMed PMID: PMC5636779.
- Moore TL, Bowley B, Shultz P, et al. Chronic curcumin treatment improves spatial working memory but not recognition memory in middle-aged rhesus monkeys. Geroscience. 2017;39(5–6):571–584. PubMed PMID: WOS:000418822500006.
- Kim S, Myers L, Wyckoff J, et al. The frailty index outperforms DNA methylation age and its derivatives as an indicator of biological age. Geroscience. 2017;391:83–92. PubMed PMID: WOS:000401331200009.
- Kane AE, Gregson E, Theou O, et al. The association between frailty, the metabolic syndrome, and mortality over the lifespan. Geroscience. 2017;392:221–229. PubMed PMID: WOS:000401636600008.
- Snider TA, Richardson A, Stoner JA, et al. The geropathology grading platform demonstrates that mice null for Cu/Zn-superoxide dismutase show accelerated biological aging. GeroScience. 2018;402:97–103. PubMed PMID: PMC5964058.