ABSTRACT
Tumour-derived exosomes (TEX) are a subset of extracellular vesicles (EVs) present in body fluids of patients with cancer. The role of this exosome subset in melanoma progression has been of interest ever since ex vivo studies of exosomes produced by melanoma cell lines were shown to suppress anti-melanoma immune responses. To study the impact of melanoma-derived exosomes (MTEX) present in patients’ plasma on melanoma progression, isolation of MTEX from total plasma exosomes is necessary. We have developed an immunoaffinity-based method for MTEX capture from plasma of melanoma patients. Using mAb 763.74 specific for the CSPG4 epitope uniquely expressed on melanoma cells, we separated MTEX from non-tumour cell-derived exosomes and evaluated the protein cargo of both fractions by quantitative flow cytometry. Melanoma-associated antigens were carried by MTEX but were not detectable in exosomes produced by normal cells. Separation of plasma-derived MTEX from non-MTEX provides an opportunity for future evaluation of MTEX as potential biomarkers of melanoma progression and as surrogates of melanoma in tumour liquid biopsy studies.
Introduction
Exosomes are virus-sized vesicles that originate from the endocytic compartment of parent cells and serve as an intercellular communication system in all unicellular and multicellular organisms [Citation1]. They are the smallest subset (30–150 nm) of extracellular vesicles (EVs) produced by all cells and are present in all body fluids [Citation2]. EVs represent a heterogeneous population of vesicles derived from various tissue and hematopoietic parent cells, including tumour cells. Human tumours, including melanoma, create a microenvironment that promotes their progression while inhibiting anti-tumour immune responses mediated by the host [Citation3]. Tumour-derived exosomes (TEX), a subset of EVs which are actively secreted by tumour cells, have recently emerged as carriers of numerous receptors, ligands and factors that modulate functions of recipient cells [Citation4]. TEX accumulate in body fluids of cancer patients and are considered to be responsible for delivering suppressive as well as stimulatory signals to immune cells [Citation5]. Using exosomes isolated from supernatants of human tumour cell lines, we have previously shown that the molecular profile of TEX is enriched in receptors and ligands involved in reprogramming of immune cells [Citation6]. Because TEX can interfere with tumour antigen-specific immunity, they might influence disease activity, therapeutic responses and disease outcome [Citation7]. TEX are also emerging as potential tumour surrogates, and their molecular and genetic contents might be viewed as promising disease biomarkers.
To date, studies of TEX have been largely performed with vesicles isolated from supernatants of tumour cell lines, where all vesicles are tumour-cell derived and represent “pure” TEX. Much of what we know about the TEX cargo or functions is based on analyses performed with EVs produced by tumour cell lines. However, exosomes in body fluids of cancer patients are mixtures of TEX and normal tissue cell-derived exosomes [Citation8]. Studies of plasma-derived exosomes have been challenging, and several limitations have been identified, including the lack of an isolation method for exosomes in body fluids that is rapid, efficient, cost-effective and applicable to clinical monitoring. Further, no strategies and reagents for separation of TEX from the bulk of non-tumour-derived vesicles exist. Current studies of plasma-derived TEX are performed with total EVs isolated by one of many available methods [Citation9] and are based on the premise that TEX represent a substantial, albeit variable, subset of total plasma EVs in patients with cancer.
We have recently described a method for isolation of morphologically intact, biologically active exosomes from cancer patients’ plasma or other body fluids by mini-size exclusion chromatography (mini-SEC) [Citation10]. Capitalizing on the capability to isolate from patients’ plasma of exosomes that are partially free of “contaminating” plasma proteins, we have developed an immunoaffinity-based capture method to separate melanoma cell-derived exosomes (MTEX) from the bulk of non-tumour exosomes present in melanoma patients’ plasma [Citation10]. To capture MTEX we use the monoclonal antibody (mAb) 763.74 specific for a peptide epitope of the tumour antigen, chondroitin sulphate peptidoglycan 4 (CSPG4). This epitope is selectively expressed on melanoma cells and not detectable on normal cells, with the exception of activated pericytes in the tumour microenvironment (TME) [Citation11–Citation13]. As a result, this mAb can differentiate malignant from normal cells. The CSPG4 epitope recognized by the 763.74 mAb is present on >80% of melanomas [Citation11], and it is carried by exosomes produced by melanoma cells (Supplementary Figure 1). Using this unique CSPG4-specific mAb, we have developed a novel and highly effective method for the isolation of a subset of CSPG4+ exosomes produced by melanoma cells (MTEX) from a pool of plasma-derived exosomes. The immune based capture and characterization of MTEX obtained from plasma of patients with melanoma offers a unique opportunity for future molecular profiling of the MTEX cargo in relation to clinical and clinicopathological data as well as disease activity in patients with melanoma.
Materials & methods
Patients and plasma specimens
Venous blood samples were obtained from patients with melanoma seen in the UPMC Hillman Cancer Center Melanoma Program Outpatient Clinic by one of the co-authors, John M. Kirkwood and his associates. Blood samples from patients were collected as per the IRB approval #970186, and all blood donors signed an informed consent form. The disease status of patients who donated blood for the study is listed in Supplementary Table 1. Blood samples were processed to separate plasma, which was then divided into 2 mL aliquots and stored frozen at −80°C until thawed and used for exosome isolation.
Table 1. Titration of Mel526 exosomes for use in MTEX capture with anti-CSPG4 mAb 763.74a.
Exosome isolation from plasma
Plasma samples were pre-clarified by centrifugation first at 2000 g for 10 min at 4º C and then at 10,000g for 30 min. Filtration through 0.22 µm Millipore filter was then performed, and a 1 mL aliquot of clarified plasma was used for exosome isolation by mini-Size-Exclusion Chromatography (SEC) as previously described [Citation10]. Briefly, 1.5 cm×12 cm mini-columns (Bio-Rad, Hercules, CA, USA; Econo-Pac columns) were packed with Sepharose 2B (Sigma-Aldrich, St. Louis, MO, USA). A porous frit was placed on top of the gel to minimize disturbance during exosome isolation. Each column was washed with 20 mL of PBS and 1.0 mL of clarified plasma was loaded onto the column and eluted with PBS. One mL fractions were collected, with the bulk of exosomes eluting in the fraction #4. The EM morphology, the vesicle size range, numbers of nanoparticles in the fraction #4 and their molecular cargos were evaluated as previously described [Citation10].
Melanoma cell line
The Mel526 cell line was obtained from Dr. Walter J. Storkus, U. of Pittsburgh. The cell line was cultured in RPMI 1640 medium supplemented with 10% (v/v) exosome-depleted fetal bovine serum (FBS) at 37ºC in an atmosphere of 5% CO2 in air. The cell line was tested for Mycoplasma contamination at regular intervals and remained Mycoplasma negative.
Exosome isolation from supernatants of Mel526 cell line
Supernatants were collected from 48-72h cultures of Mel526 cells and were clarified by centrifugation first at 2,000xg for 10 min and then at 10,000xg for 30 min. Both centrifugations were performed at 4ºC. Supernatants were passed through 0.22µM Millipore filters. Each clarified supernatant was then concentrated on Viva-Spin cartridges (Sartorius, MWCO 100,000). Exosomes were isolated from the concentrated supernatant using mini-SEC as described above. To optimize exosome isolation and capture, at least 5 separately generated supernatants were used.
Protein content of exosomes
The protein content of the isolated exosome fractions was measured using BCA protein assay kit (Pierce Biotechnology, Rockford, IL).
Antibodies used for exosome capture
Monoclonal antibodies (mAbs) 763.74 and 225.28 which recognize distinct and spatially distant epitopes of CSPG4 were generated and characterized [Citation14,Citation15]. The mAbs were purified from ascitic fluids by affinity chromatography on Protein G. The purity and activity of mAbs were assessed by SDS-PAGE and by binding assays with CSPG4-expressing cells. CD63-specific mAb (clone H5C3) was purchased from Biolegend (San Diego, CA). For immunocapture of exosomes, anti-CSPG4 mAbs and anti-CD63 mAb were biotinylated using a one-step antibody biotinylation kit purchased from Miltenyi Biotec (Auburn, CA) as recommended by the manufacturer.
Antibodies used for antigen detection on exosomes
In addition to anti-CSPG4 mAbs, the following mAbs specific for melanoma-associated antigens (MAA) were used for detection: anti-gp100 (clone HI10a), anti-VLA4 (clone 9F10), anti-TYRP2 (clone 93260) purchased from Biolegend and anti-MelanA (clone A106) purchased from Santa Cruz Biotechnology (Dallas, TX). Anti-CSPG4 mAbs were labelled for flow cytometry using a Lightning-Link APC Antibody Labelling Kit purchased from Novus Biologicals (Littleton, CO).
Immune capture of MTEX from plasma-derived exosomes
illustrates the strategy for immunocapture of exosomes using anti-CSPG4 mAb 763.74. Following mAbs biotinylation, the optimal ratios of exosome: mAbs: beads were established in preliminary experiments (see below). An aliquot of the miniSEC fraction #4 containing plasma-derived exosomes (10µg protein) was co-incubated with biotinylated anti-CSPG4 mAb 763.74 (4μg) for 12h at 4°C. Subsequently, MTEX capture was performed using 100μL of streptavidin-coated magnetic beads from an ExoCap™ Streptavidin Kit (MBL International Corporation, Woburn, MA) in a 1.5 mL Eppendorf microfuge tube, which was then gently agitated on a shaker at 4ºC overnight. Next, the bead-exosome complexes were collected using a magnet, and the supernatant containing non-captured exosomes was harvested. MTEX captured on beads were washed twice with fresh PBS and re-suspended in 250 µL of PBS for analyses of the exosome cargo by flow cytometry. Supernatant containing non-captured exosomes was harvested, and the exosomes were re-captured on streptavidin beads coated with biotinylated anti-CD63 Abs in preparation for the detection of antigens present in the exosome cargo by flow cytometry.
Figure 1. A schema for isolation of exosomes from plasma by mini-SEC followed by capture of MTEX from fraction #4 which contains the bulk of non-aggregated exosomes . The recovered exosomes are partly “purified” by removal of protein/high density lipoproteins (HDL) complexes on the miniSEC column. MTEX are co-incubated with biotinylated, pre-tittered anti-CSPG4 Ab and are captured on streptavidin-coated magnetic beads, and the bead-bound MTEX-Ab complexes are recovered using a magnet and non-captured exosomes in the supernatant are recovered. Both fractions are available for analysis (A), although free-floating non-tumour-derived exosomes have to be re-captured on beads for subsequent profiling by flow cytometry.
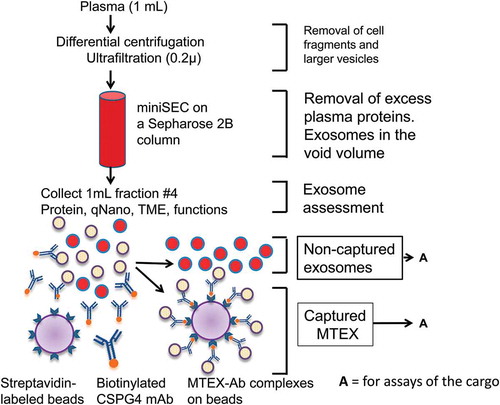
Titrations of CSPG4-specific mAb 763.74 for MTEX capture
To determine the optimal protein concentrations of biotinylated anti-CSPG4 mAb 763.74 for MTEX capture, titrations were performed using MTEX isolated by miniSEC from culture supernatants of the Mel526 cell line. The optimal ratio of the capture mAb: exosome protein: magnetic bead volume was determined using different concentrations of the biotinylated anti-CSPG4 mAb 763.74, constant protein levels of exosomes (5 or 10ug) and different bead volumes. Briefly, MTEX were mixed with biotinylated-763.74 mAb at the concentration of 1, 2, 4, 10 or 20 μg in 1.5 mL microfuge tubes. Following an overnight incubation at 4ºC with gentle agitation on a shaker, streptavidin-coated magnetic beads (25, 50, 100 or 150 µL) were added, and incubation on a shaker was continued overnight. Beads were harvested using a magnet, washed 2X with PBS and re-suspended in 250 µL of PBS for flow cytometry. The optimal ratios of the three components (i.e. exosomes, capture mAb and beads) were established by flow cytometry measuring the percentages and mean fluorescence intensities (MFI) of CSPG4+ or CD63+ exosomes bound onto streptavidin-coated magnetic beads. The lowest mAb concentration that captured all MTEX in the tested exosome sample was considered to be the optimal concentration.
To study molecular cargo of non-captured exosomes (see by flow cytometry, they had to be loaded onto magnetic beads. Therefore, these exosomes (10ug protein) were re-captured using 2 μg of biotinylated anti-CD63 mAb and 100 uL of streptavidin-coated beads. Anti-CD63 mAb was titrated in preliminary experiments using exosomes isolated from the Mel526 cell line supernatants, as described above for anti-CSPG4 mAbs. Once placed on beads, exosomes were washed 2X with PBS and re-suspended in 250 μL of PBS in preparation for antigen profiling by flow cytometry.
Recovery of captured MTEX and non-captured exosomes
Since protein levels of the total exosome fraction #4 used for immunocapture of MTEX were known, the recovery of CSPG4+ exosomes after immunocapture was determined by subtracting protein levels measured in the non-captured fractions from the total exosome protein used for capture.
Antigen detection on exosomes by flow cytometry
The staining method used for quantitative flow cytometry analysis of the cargo of exosomes loaded on beads was modified from that described by Morales-Kastrana and Jones [Citation16]. is a schematic presentation of the method used for loading of exosome-Ab complexes onto streptavidin-labelled beads followed by staining with a fluorochrome-labelled detection Ab of interest (e.g. anti-CSPG4 Ab in . Briefly, for detection of exosome-associated proteins, exosomes (0.4 μg/100 uL PBS) captured on beads as described above were first pre-incubated with 10 uL de-complemented mouse serum in 1.5 mL microfuge tubes for 30 minutes. This step was included to block non-specific binding of detection Abs to captured exosomes. pre-titered fluorochrome-labelled detection mAbs or isotype control mAbs were then added to respective microfuge tubes, which were incubated for 1h at RT with gentle agitation on a shaker. Each detection Ab was used singly for detection of a specified exosome component. The tubes were placed on a magnet and supernatants were carefully removed without disturbing the pelleted beads. Preliminary titration experiments were performed individually for every detection Ab to determine its optimal concentration to be used with 5 or 10μg of exosome protein and with 10μL of streptavidin-labelled beads. The beads were washed 2X with 100μL aliquots of PBS and the volume was adjusted to 200μL with PBS for flow cytometry. Flow cytometry exosome profiling was performed using GalliosTM flow cytometer (Beckman Coulter). This detection method provided relative fluorescence intensity (RFI) values defined as the ratio of MFI with detection Ab/MFI with isotype control Ab (). The RFI values for captured MTEX were normalized to total exosome protein (in
Figure 2. Detection of CSPG4+ exosomes immunocaptured on beads by flow cytometry. Exosomes are captured on streptavidin-labelled beads using pre-titered biotin-labelled anti-CSPG4 mAb (763.74 mAb). The antigen (CSPG4) carried by the bead-bound exosomes is detected using a fluorochrome-labelled and pre-tittered detection anrti-CSPG4 mAb (225.28 mAb). The flow cytometry-based detection provides the relative fluorescence intensity (RFI) value for exosomes carrying the antigen. RFI = MFI of detection Ab/MFI of isotype control Ab.
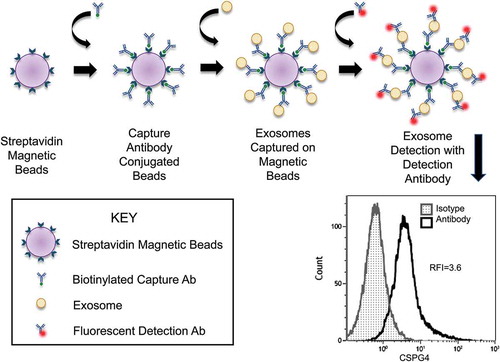
Reproducibility of the MTEX capture and detection methods
Reproducibility of MTEX capture by anti-CSPG4 mAb 763.74 and of exosome capture by anti-CD63 mAb was evaluated by using exosomes of three melanoma patients and capturing/re-capturing exosomes 3X. The nine separate capture values for each mAb were used to calculate the experimental error. Similarly, to evaluate reproducibility of flow cytometry-based detection, captured exosomes on beads were obtained from three donors and tested in three parallel assays for percentages of CSPG4+ or CD63+ exosomes. The data were used to calculate the experimental error.
Data analysis
The data were summarized by descriptive statistics (IBM SPSS, version 23) such as means and standard errors (SE). Statistical analysis was performed using GraphPad Prism (version 6). Flow cytometry analyses were performed with VenturiOne version 5.0 or Kaluza v1.5 (Beckman Coulter). To determine reproducibility of MTEX immunocapture or Ab-based detection by flow cytometry, interclass correlation coefficients were calculated. The standard error measurement was used to determine the 95%prediction intervals. A p-value of <0.05 was considered to be statistically significant for all the presented data.
Results
Titrations of anti-CSPG-4 mAb, exosomes and beads for immunocapture of MTEX
We first proceeded to establish the optimal ratio of mAb: exosome: beads for capture of MTEX using exosomes isolated from supernatants of the Mel526 cell line. This cell line was selected, because it has high surface CSGP4 expression (Supplementary Figure 1). Furthermore, the exosomes isolated from its supernatants carry CSPG4, as determined by flow cytometry analysis of exosomes stained with CSPG4-specific mAb 763.74 (Supplementary Figure 1). In the titration experiments with these Mel526 cell-derived exosomes, MTEX immune capture with 763.74 mAb was followed by flow cytometry-based detection using mAb 225.28 specific for a distinct CSPG4 epitope or the anti-CD63 mAb to confirm the exosome origin of MTEX. The data in show that with 1 μg of anti-CSPG4 mAb 763.74, 5 μg MTEX protein and 100 μL of beads, all of the captured exosomes were CSPG4+ and nearly all were CD63+. Titrations performed with higher concentrations (2–20 μg) of biotinylated anti-CSPG4 mAb 763.74 gave lower MTEX recovery (data not shown). As all exosomes in the supernatant of Mel526 cells are MTEX, these results indicated that under the experimental conditions defined above, nearly all CSPG4+CD63+ MTEX were being captured. The immunocapture experiment with anti-CSPG4 mAb 763.74 was repeated 3 times with the mean capture efficiency for CSPG4+ MTEX of 98% and for CD63+ MTEX of 95%.
Next, to optimize the volume of beads used for MTEX capture, additional titrations were performed as shown in . Anti-CSPG4 mAb 763.74 was used at the concentration of 1μg with MTEX protein at 5 μg, as above, while volumes of beads varied from 25 to 150 μL as shown in . Detection of the CSPG4 epitope or of CD63 protein by flow cytometry indicated that 100μL of beads were sufficient for near complete capture of 5μg of MTEX by 1μg of anti CSPG4 mAb 763.74 ().
Table 2. Titrations of bead volumes for MTEX capture with biotinylated anti-CSPG4 mAba.
Selection of the strategy for MTEX immunocapture
Using the experimental conditions defined above, we next selected the strategy for capture of MTEX from the total exosome fractions. Two approaches were compared using exosomes isolated from supernatants of a Mel526 cell line. In the first, biotinylated anti-CSPG4 mAb 763.74 was added to exosomes in suspension to capture MTEX. This was followed by binding of the MTEX-Ab complexes to streptavidin-charged beads. In the second approach, exosomes were added to the suspension of streptavidin-charged beads already coated with anti-CSPG4 mAb 763.74. Both immunocapture strategies were followed by detection of CSPG4+ and CD63+ exosomes by flow cytometry. We observed no difference in the capture efficiency of Mel526 cell-derived MTEX with either of the two capture strategies (data not shown). Therefore, we have selected to use the capture platform in which the Ab is added to exosome suspensions prior to capture of the complex onto beads in all subsequent experiments.
Antibody titrations for capture of plasma-derived MTEX
Exosomes from plasma of melanoma patients originate from various cell types and only a fraction is expected to be tumour cell-derived. MTEX levels are likely to vary in every sample. When the capture conditions established for Mel526 cell-derived MTEX capture were tested using exosomes in the mini-SEC fraction #4 obtained from plasma of melanoma patients, minimal or no immunocapture of MTEX was detected. Therefore, additional titration experiments were performed to define capture conditions optimal for isolation of MTEX from plasma-derived exosomes. Plasma specimens obtained from three randomly selected melanoma patients were used as a source of exosomes. The anti-CSPG4 mAb 763.74 concentration was increased to 4μg and the exosome protein level to 10ug, keeping the beads volume at 100μL. The data in show that patient no.1 had no CSPG4+ MTEX, patient no. 2 had a moderate level and patient no.3 had even more, as measured by flow cytometry. The results suggested that successful capture of CSPG4+MTEX from melanoma patients’ plasma required higher concentrations of the capture mAb and of exosomes relative to concentrations used to capture MTEX from supernatants of Mel526 cells. To demonstrate that the MTEX capture in these specimens was complete, we calculated protein levels in the captured and non-captured fractions. In patient no.1, the MTEX fraction contained 22% of the input exosome protein; in patient no.2, 24% and in patient no. 3, 58% (Supplementary Table 2). The data suggested that the exosome protein distribution between captured vs non-captured fractions was roughly comparable to the RFI values for CSPG4+ MTEX measured by flow cytometry. Specifically, in patient no.3, with the highest content of captured CSPG4+MTEX at an RFI of 2.7, the protein recovery at 58% was also higher than that for the other two patients (22% and 24%, respectively).
Table 3. Immunocapture of MTEX from plasma of 3 patients with melanomaa.
MTEX capture from plasma of melanoma patients with different disease stages
Levels of MTEX in plasma of patients with melanoma may vary, depending on the disease stage and activity [Citation7]. Therefore, it was necessary to test whether the capture conditions established with exosomes of three randomly selected melanoma patients are valid for capture of MTEX in patients with early disease as well as those with metastatic melanoma. Plasma exosomes were isolated from two patients with less advanced disease (pts #4 and #5) and from a patient with disseminated metastatic melanoma (pt #6; see Supplementary Table 1). First, we confirmed that the ratio of 4 μg anti-CSPG4 mAb: 10 μg exosomes: 100 μL beads was optimal for capture by titrating the mAb concentration with exosomes at 5 or 10 μg protein levels and measuring MTEX recovery in all three patients (). No MTEX were captured using 5 μg exosomes. Utilizing exosomes at 10μg, mAb titrations confirmed that 4μg of the mAb was optimal for recovery of nearly all MTEX present in the input exosomes (i.e. fraction #4). Using an aliquot of total exosomes, the RFI values for CSPG4+ MTEX were determined for the three patients by detection flow cytometry performed prior to immunocapture (). These pre-capture values for MTEX/total input exosomes were almost identical to the percentages of CSPG4+MTEX recovered after immunocapture with 4μg of anti-CSPG4 mAb 763.74/10 μg exosome protein/100μL beads. The data indicated that under the capture conditions used, MTEX recovery was nearly complete even for patient no.6, whose total plasma exosomes were enriched in MTEX. Based on these results, 4 μg of anti-CSPG4 mAb 763.74 was used for MTEX capture in all subsequent experiments with plasma-derived exosomes.
Table 4. MTEX capture from plasma-derived exosomes and MTEX recovery in melanoma patients with different disease stagesa.
Reproducibility of the methodology to immunocapture MTEX from melanoma patients’ plasma
Using exosomes from plasma of patients no.4, no. 5, no. 6 and the capture conditions defined above, MTEX capture with anti-CSPG4 mAb 763.74 as well as re-capture with anti-CD63 mAb of non-MTEX were repeated three times in three separate experiments. The antigen detection (for CSPG4 or CD63) by flow cytometry with captured/non-captured exosomes was then performed. The recoveries in percentages of captured CSPG4+MTEX and CSPG4+ or CD63+ non-MTEX captured in each of the 3 patients in 3 independent experiments are listed in the Supplementary Table 3. The data show that MTEX capture with anti-CSPG4 mAb followed by detection with anti-CSPG4 mAb (using either the mAb 763.74 or mAb 225.28) had the intra-class correlation coefficient of 0.98 with the 95% prediction interval at ± 5.8. For the MTEX detection with anti-CD63 mAb (data not shown) the values were 0.97 ± 6.8. Also, the re-capture of MTEX from the same total exosomes with additional aliquots of anti-CSPG4 mAb consistently gave negative results. These data indicated that MTEX capture with anti-CSPG4 mAb was highly reproducible and reliably captured all CSPG4+ exosomes present in the total input exosome fractions. The RFI values for captured CSPG4+ and CD63+ exosomes usually correspond to each other, suggesting that captured CSPG4+MTEX are largely CD63+ (). We compared efficiency of exosome immunocapture using anti-CSPG4 mAb vs. anti-CD63 mAb and found the results were similar (data not shown). Additionally, using exosomes captured with anti-CD63 Ab, we performed detection with labelled 763.74 Ab in the presence of unlabelled 225.28 Ab and vice versa to show that these Abs do not interfere with one another in detection of exosomes captured on beads
Figure 3. Flow cytometry-based detection of CSPG4 antigen or CD63 antigen carried on exosomes which were immunocaptured with anti-CSPG4 mAb from plasma of five melanoma patients. Note that the RFI values for CSPG4+MTEX captured from plasma of these patients varied, and that the frequency of CSPG4+ exosomes corresponds to that of CD63+ exosomes, suggesting that most of captured MTEX are CD63 +. The asterisks (patients #6 and #8) indicate patients with the highest RFIs and highly advanced metastatic disease (see Supplementary Table 1).
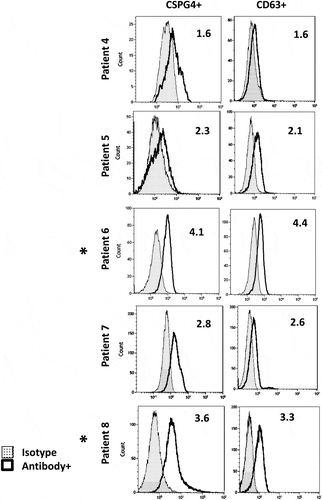
Phenotypic analysis of captured MTEX and non-captured exosomes
A representative antigen detection experiment using plasma exosomes of a patient #6 with metastatic melanoma is shown in . In this patient, nearly all of captured MTEX were CSPG4+ and a comparable fraction was CD63+ (RFI values 4.1 vs. 4.4). The non-captured exosomes after re-capture with anti-CD63 Ab were not stained with labelled CSPG4-specific mAb 763.74; and most were stained by anti-CD63 mAb. Staining of these captured MTEX for selected melanoma-associated antigens (MAA) provided a limited cargo profile, showing enrichment in TYRP2 and MelanA, but low contents of gp100 and VLA4 in the MTEX of patient no.6. summarizes the antigen detection data for the CSPG4+MTEX captured from plasma-derived exosomes of five melanoma patients. The content of different MAAs is highly variable among these patients. In contrast to the MAA profile of MTEX in patients no.6 and no.8 with advanced metastatic disease, the MTEX of patient no. 4 with early disease carry few MAAs. Further, plasma-derived exosomes of this patient contained few MTEX relative to a high proportion of MTEX in patients no.6 and no.8.
Table 5. Detection of melanoma-associated antigens (MAAs) carried by captured MTEX or non-captured exosomesa.
Figure 4. A representative flow cytometry for detection of melanoma-associated antigens (MAAs) on the immunocaptured MTEX fraction and on non-captured exosomes of patient no.6. Note that MAAs are carried by CSPG4+ MTEX but not by non-captured exosomes.
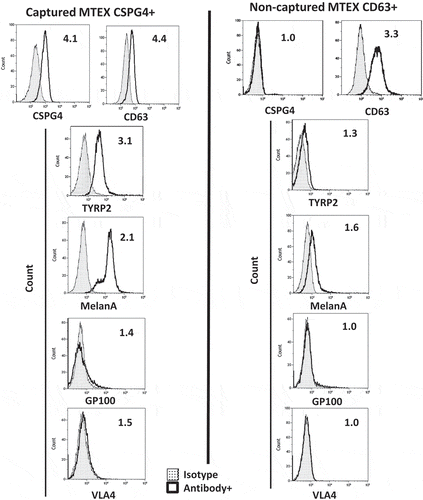
The non-captured exosomes were negative for the CSPG4 epitope and either did not carry or carried a low cargo of the other MAAs tested (). The exception was patient no.6, whose non-captured exosomes carried MelanA.
Discussion
Recent studies of EVs in patients with cancer indicate that these vesicles are heterogeneous, encompassing a mixture of EVs derived from normal and tumour cells [Citation8,Citation17]. Only some of EVs populating all body fluids in these patients are tumour-derived and it is likely that the ratios of tumour cell-derived vs normal cell-derived EVs vary from patient to patient. Human tumours produce and release a variety of EV subtypes which originate from different cell compartments and differ in molecular and genetic composition, size and, potentially, in biological effects they mediate [Citation18]. Among these various EV subtypes, exosomes, and especially TEX, have been the focus of intense scientific inquiry [Citation19,Citation20]. TEX have been reported to mediate immune suppression and to carry a variety of immunosuppressive molecules and factors known to interfere with immune cell functions [Citation4,Citation21]. TEX are also involved in tumour growth and progression via autocrine or juxtacrine interactions [Citation20] and in immune regulation via the cross-talk with immune cells in the tumour microenvironment (TME) [Citation22]. Of special interest is the potential role of TEX as biomarkers of tumour progression and as liquid tumour biopsies [Citation23]. To date, however, TEX have largely been studied as by-products of tumour cell lines maintained in cultures or as an undefined component of total exosomes in plasma of patients with cancer. In the absence of methodology to isolate TEX and separate them from non-tumour-derived vesicles, the concept of a “liquid biopsy” as a surrogate of the tumour is currently being tested with total EV fractions isolated from patients’ plasma. Several methods for EV isolation have been introduced, but as yet none are validated technologies [Citation9] . Given the existing phenotypic and functional heterogeneity of EVs in patients’ plasma, further progress in establishing the liquid biopsy paradigm is dependent upon the development of methods for isolation and separation of TEX from the bulk of EVs present in body fluids of cancer patients. Only by confirming the tumour origin, nature and molecular/genetic content of TEX will it become possible to reliably define their role as circulating surrogates of tumour cells.
Exosomes circulating in cancer patients’ plasma are expected to carry a unique set of membrane-embedded molecules, which mimic those present in the parent tumour cells. Thus, TEX could be separated from non-tumour-derived vesicles using antibodies specific for these tumour-associated antigens (TAAs). Indeed, many attempts have been made to use mAbs specific for various human TAAs such as CD34 [Citation24], EPCAM [Citation25], HER2 [Citation25], Survivin [Citation26], PSA [Citation27], tetraspanin [Citation28] or glipican-1 [Citation19] for isolation of TEX from cancer plasma. The technologies using mAbs for immune capture of exosomes depend on flow cytometry, bead-based captures, microfluidic chips or immunocapture-based ELISA as reviewed by Chiesi and collaborators [Citation29]. Conceptually, all of these methods have the same limitation; namely, they target antigens which are tumour-associated and are not tumour-specific. While these antigens may be overexpressed in tumour cells, their concomitant expression on normal cells results in immune capture from patients’ plasma of exosomes that cannot be said to be tumour-derived. Hence, the above strategies provide an enrichment in TEX but do not separate tumour-derived from non-tumour-derived exosomes.
We have developed and describe here a new immunoaffinity-based method for separation of melanoma-derived exosomes (dubbed MTEX) from normal cell-derived exosomes in plasma of patients with melanoma. MTEX isolation from patients’ plasma depends on recognition by mAbs specific for antigenic epitopes of melanoma-associated antigens (MAAs) that are expressed only on melanoma cells and are absent from normal tissue cells. Few mAbs with such restricted specificity exist. We have generated and extensively characterized mAbs recognizing unique epitopes of CSPG4 on melanoma cells [Citation11–Citation15] that are also carried by exosomes produced by these cells. One of the mAbs (clone 763.74) with high binding avidity to the defined CSPG-4 epitope was selected for MTEX isolation and their separation from normal cell-derived exosomes in plasma.
In addition to a requirement for an Ab with unique specificity for an epitope expressed exclusively on melanoma cells, MTEX capture and isolation from plasma is strictly dependent on the availability of “contaminant”-depleted, morphologically intact, non-aggregated exosomes, which carry the antigenic epitope recognized by the mAb selected for capture. However, when isolated from plasma, all EVs are coated by plasma proteins or protein/nucleic acid aggregates, which limit access and recognition of antigens. To overcome this limitation, we aimed first at the removal of the bulk of plasma “contaminants” by passing pre-cleared plasma through a mini-SEC column [Citation10]. The vesicles recovered in the column void volume are non-aggregated, partly “purified” CD63+TSG101+ exosomes that are morphologically intact, functional and sized at 30-150nm [Citation10]. The mini-SEC-based exosome isolation is simple, reliable, high throughput and inexpensive, and thus is applicable to serial exosome isolations on a clinical scale [Citation10]. The plasma-derived exosomes isolated by mini-SEC are incubated with a selected capture mAb, which is biotinylated to allow for its binding to streptavidin-coated beads 3 µm in diameter that are large enough to accommodate mAb-MTEX complexes at the density necessary for subsequent detection by flow cytometry [Citation16].
One could argue that immune capture of exosomes directly from plasma, without initial mini-SEC-based isolation, would simplify MTEX recovery. We have attempted to add the capture mAbs directly to pre-cleared plasma. However, binding of the capture mAb to exosomes was in a large part blocked in the presence of plasma proteins, compromising the reproducibility of immune capture (data not shown). In contrast, the addition of appropriately titrated capture mAbs to isolated and partly “purified” exosomes was effective in selective capture of CSPG4+ MTEX with highly reproducible results (Supplementary Table 3).
Admittedly, the requirement for optimizing the ratios of exosomes: capture Abs: beads that is necessary for anchoring the exosome-Ab complexes to beads is labour-intensive. However, once this ratio was established for anti-CSPG4 mAb 763.74, MTEX capture reliably segregated total exosomes into melanoma-derived MTEX decorated by melanoma-associated antigens (MAAs) and non-captured exosomes with the molecular profile largely devoid of MAAs. It is notable that the molecular profiles of isolated MTEX were qualitatively and quantitatively distinct for every melanoma patient.
Once separated from each other, both MTEX and non-captured exosomes were phenotyped to determine their respective protein profiles, using an on-bead flow cytometry-based detection method (). As this method is dependent on coupling exosomes to beads, the non-captured exosomes had to be re-captured using biotinylated anti-CD63 Abs and streptavidin-coated beads prior to detection. Using carefully pre-tittered fluorochrome-labelled detection Abs, we obtained semi-quantitative individual assessments based on relative flow intensity (RFI) values for each targeted protein carried by exosomes. This provided a molecular profile limited to the few proteins that we tested for. The advantages of this detection method are that it is economical, as small numbers of exosomes coupled to beads are needed, and that Ab-based amplification increases its sensitivity to enable detection of exosome antigens present in femtomolar quantities, which are not detectable even by mass spectrometry in our hands. It is possible to measure double positive exosomes in one tube (instead of separate single staining) and to evaluate intraluminal content of exosomes after permeabilization by this method. This exosome detection method has replaced the western blots in our laboratory, allowing for evaluation of the capture efficiency as well as the molecular cargo in both captured and non-captured exosomes.
The rationale for MTEX capture was to probe their molecular content for the presence of antigens that could potentially serve as biomarkers of melanoma progression and outcome. The ability to isolate MTEX, to separate them from non-tumour-derived vesicles and to probe their cargo for MAA or other molecular or genetic markers of the tumour will facilitate testing of the role of MTEX as liquid biopsies in melanoma. When applied to serial monitoring of MTEX in plasma of melanoma patients, the capture strategy we have introduced is likely to be instrumental in evaluating biological and clinical significance of MTEX as potential melanoma biomarkers. However, it is likely that the profile of MTEX rather than any single MAA will serve as a biomarker of disease activity, progression and outcome. In the ongoing studies, we are currently correlating MTEX levels in melanoma patients’ plasma, their molecular profiles and their functional properties with the clinical endpoints, including stage, clinical course of the disease, response to therapy and outcome.
Supplementary_Figure_and_Tables_Sharma_et_al._JEV_revision.docx
Download MS Word (143.3 KB)Acknowledgments
This work was supported in part by NIH Grants R01 CA168628 and R21 CA205644 to TLW and NIH grant P50 CA121973 to JMK. LM was a fellow of the Swiss National Foundation (#PBSKP3 140119/1). This project also used UPMC Hillman Cancer Center shared facilities that are supported in part by award P30 CA047904.
Disclosure statement
No potential conflict of interest was reported by the authors.
Supplementary materials
Supplemental data for an article can be accessed here.
Additional information
Funding
References
- Wendler F, Stamp GW, Giamas G. Tumor-stromal cell communication: small vesicles signal big changes. Trends Cancer. 2016 Jul;2(7):1–14.
- Keller S, Ridinger J, Rupp AK, et al. Body fluid derived exosomes as a novel template for clinical diagnostics. J Transl Med. 2011;9:86.
- Whiteside TL. Tumor-derived Exosomes and their role in tumor-induced immune suppression. Vaccines (Basel). 2016 Oct 20;4:4.
- Whiteside TL. Exosomes and tumor-mediated immune suppression. J Clin Invest. 2016 Apr 1;126(4):1216–1223.
- Valenti R, Huber V, Iero M, et al. Tumor-released microvesicles as vehicles of immunosuppression. Cancer Res. 2007 Apr 1;67(7):2912–2915.
- Wieckowski EU, Visus C, Szajnik M, et al. Tumor-derived microvesicles promote regulatory T cell expansion and induce apoptosis in tumor-reactive activated CD8+ T lymphocytes. J Immunol. 2009 Sep 15;183(6):3720–3730.
- Peinado H, Aleckovic M, Lavotshkin S, et al. Melanoma exosomes educate bone marrow progenitor cells toward a pro-metastatic phenotype through MET. Nat Med. 2012 Jun;18(6):883–891.
- Koliha N, Wiencek Y, Heider U, et al. A novel multiplex bead-based platform highlights the diversity of extracellular vesicles. J Extracell Vesicles. 2016;5:29975.
- Li P, Kaslan M, Lee SH, et al. Progress in Exosome Isolation Techniques. Theranostics. 2017;7(3):789–804.
- Hong CS, Funk S, Muller L, et al. Isolation of biologically active and morphologically intact exosomes from plasma of patients with cancer. J Extracell Vesicles. 2016;5:29289.
- Campoli MR, Chang CC, Kageshita T, et al. Human high molecular weight-melanoma-associated antigen (HMW-MAA): a melanoma cell surface chondroitin sulfate proteoglycan (MSCP) with biological and clinical significance. Crit Rev Immunol. 2004;24(4):267–296.
- Wang X, Katayama A, Wang Y, et al. Functional characterization of an scFv-Fc antibody that immunotherapeutically targets the common cancer cell surface proteoglycan CSPG4. Cancer Res. 2011 Dec 15;71(24):7410–7422.
- Schlingemann RO, Rietveld FJ, de Waal RM, et al. Expression of the high molecular weight melanoma-associated antigen by pericytes during angiogenesis in tumors and in healing wounds. Am J Pathol. 1990 Jun;136(6):1393–1405.
- Wang Y, Sabbatino F, Wang X, et al. Detection of chondroitin sulfate proteoglycan 4 (CSPG4) in melanoma. Method Mol Biol. 2014;1102:523–535.
- Temponi M, Gold AM, Ferrone S. Binding parameters and idiotypic profile of the whole immunoglobulin and Fab’ fragments of murine monoclonal antibody to distinct determinants of the human high molecular weight-melanoma associated antigen. Cancer Res. 1992 May 1;52(9):2497–2503.
- Morales-Kastresana A, Jones JC. Flow cytometric analysis of extracellular vesicles. Method Mol Biol. 2017;1545:215–225.
- Koliha N, Heider U, Ozimkowski T, et al. Melanoma affects the composition of blood cell-derived extracellular vesicles. Front Immunol. 2016;7:282.
- Atay S, Godwin AK. Tumor-derived exosomes: a message delivery system for tumor progression. Commun Integr Biol. 2014 Jan 1;7(1):e28231.
- Melo SA, Luecke LB, Kahlert C, et al. Glypican-1 identifies cancer exosomes and detects early pancreatic cancer. Nature. 2015 Jul 9;523(7559):177–182.
- Matei I, Kim HS, Lyden D. Unshielding exosomal RNA unleashes tumor growth and metastasis. Cell. 2017 Jul 13;170(2):223–225.
- Whiteside TL. Immune modulation of T-cell and NK (natural killer) cell activities by TEXs (tumour-derived exosomes). Biochem Soc Trans. 2013 Feb 1;41(1):245–251.
- Muller L, Mitsuhashi M, Simms P, et al. Tumor-derived exosomes regulate expression of immune function-related genes in human T cell subsets. Sci Rep. 2016;6:20254.
- Whiteside TL. The potential of tumor-derived exosomes for noninvasive cancer monitoring. Expert Rev Mol Diagn. 2015 Aug;2:1–18.
- Hong CS, Muller L, Boyiadzis M, et al. Isolation and characterization of CD34+ blast-derived exosomes in acute myeloid leukemia. PloS One. 2014;9(8):e103310.
- Fang S, Tian H, Li X, et al. Clinical application of a microfluidic chip for immunocapture and quantification of circulating exosomes to assist breast cancer diagnosis and molecular classification. PloS One. 2017;12(4):e0175050.
- Khan S, Jutzy JM, Valenzuela MM, et al. Plasma-derived exosomal survivin, a plausible biomarker for early detection of prostate cancer. PloS One. 2012;7(10):e46737.
- Logozzi M, Angelini DF, Iessi E, et al. Increased PSA expression on prostate cancer exosomes in in vitro condition and in cancer patients. Cancer Lett. 2017 Sep;10(403):318–329.
- Logozzi M, de Milito A, Lugini L, et al. High levels of exosomes expressing CD63 and caveolin-1 in plasma of melanoma patients. PloS One. 2009;4(4):e5219.
- Zarovni N, Corrado A, Guazzi P, et al. Integrated isolation and quantitative analysis of exosome shuttled proteins and nucleic acids using immunocapture approaches. Methods. 2015 Oct;01(87):46–58.