ABSTRACT
This article aims to document the growth in extracellular vesicle (EV) research. Here, we report the growth in EV-related studies, patents, and grants as well as emerging companies with major intent on exosomes. Four different databases were utilized for electronic searches of published literature: two general databases – Scopus/Elsevier and Web of Science (WoS), as well as two specialized US government databases – the USA Patent and Trademark Office and National Institutes of Health (NIH) of the Department of Health and Human Services. The applied combination of key words was carefully chosen to cover the most commonly used terms in titles of publications, patents and grants dealing with conceptual areas of EVs. Within the time frame from 1 January 2000 to 31 December 2016, limited to articles published in English, we identified output using search strategies based upon Scopus/Elsevier and WoS, patent filings and NIH Federal Reports of funded grants. Consistently, USA and UK universities are the most frequent among the top 15 affiliations/organizations of the authors of the identified records. There is clear evidence of upward streaming of EV-related publications. By documenting the growth of the EV field, we hope to encourage a roster of independent authorities skilled to provide peer review of manuscripts, evaluation of grant applications, support of foundation initiatives and corporate long-term planning. It is important to encourage EV research to further identify biomarkers in diseases and allow for the development of adequate diagnostic tools that could distinguish disease subpopulations and enable personalized treatment of patients.
Significance and innovation
In the last decade, there has been an unexpected growth of publications in the field of exosomes, microvesicles, and extracellular vesicles (EVs). Despite this growth, scientists from other fields, patient advocates and philanthropic agencies have not fully embraced the field. This uncertainty reflects the lack of reference standards, publications on replication of results, broad understanding of the functional and regulatory features of these particles and exemplars of translation from basic science to patient care. This uncertainty has made difficult the acceptance of this field by the public, regulatory agencies, politicians, investors and corporate representatives responsible for plotting long-term strategies. By reviewing the expansion of the EV field, we hope to promote forward-thinking initiatives and acceptance.
EVs are lipid-based membrane vesicles of endocytic origin that are released by both healthy and cancerous cells [Citation1]. The term “exosomes” is often used for a nano-scale subpopulation of EVs, presumably of endocytic origin, although this last point is rarely demonstrated. The term “microvesicles” is used for EVs of various sizes, presumably shed from the plasma membrane. EVs can be speciated by their concentrations in biofluid, their cargo and their functional effects. The particles are distinguishable from other residents of biofluids including circulating cells from which vesicles emerge, circulating tumour cells, both cell-free DNA (cfDNA) and RNA, and genetic materials bound to circulating lipids [Citation2,Citation3]. Shed into biofluids by organ-specific cell populations, EVs contain and express genetic materials on their surfaces reflective of the parent cell [Citation4]. All human biofluids, such as blood and urine, contain EVs whose transferred genetic materials alter functions of local or distant recipient cells.
Recent interest in exosomes and microvesicles comes because of the identification of their function as mediators of intercellular communication and immune regulation [Citation4,Citation5]. As EVs exist at numbers exceeding 1000 particles for each cell of origin, their enumeration in biofluids offers quantitative advantages over the paucity of circulating tumour cells, exfoliated circulating DNA, interference RNA, and antibodies directed against cytoplasmic proteins [Citation6]. Thus, quantities of EVs can be isolated from bodily fluids through “minimally invasive” approaches and prepared using relatively low-cost high-throughput ultracentrifugation and filtration; and selectively isolated and accumulated using affinity procedures [Citation7–Citation9].
Not surprizingly, EVs (generally called “exosomes”, but we prefer here the generic term) have emerged as biomarkers for disease diagnosis and prognosis and are being constructed as targeted therapeutics in the US and Europe. EVs offer an attractive pathway providing both minimally invasive diagnostics as well as longitudinal therapeutic response metrics of cancer. This approach involves EVs from blood for cancers of lung, breast and leukaemia; from urine for prostate cancer diagnosis and rejection metrics for renal allografts; and cerebrospinal fluid (CSF) for glioblastoma and Alzheimer’s disease [Citation10,Citation11].
Cancer institutes and designated cancer centres are the potential largest end-users of these liquid biopsies; with an estimated revenue share of 38% – significantly higher than hospitals, academic research institutes, and diagnostic centres [Citation12]. The lead position of cancer institutes will expand as exosomal therapies come on line for gastrointestinal cancer management, for the diagnosis of inoperable cancers and to address cancer-specific gene amplifications and mutations [Citation10,Citation11,Citation13]. As companies develop targeted therapies, there will be a corresponding demand for companion “liquid biopsy” response metrics reflecting changes in the targeted cancer pathway [Citation12] as well as resistance mechanisms of cells. Generally, the commercialization of therapeutics is more financially rewarding than companion diagnostics, and thus commercialization currently favours EV pipelines with product launches in Italy, the UK, US and Korea [Citation12,Citation14]. Companies with major intent include Exosome Sciences, Inc. (US), Exosome Diagnostics Inc. (US), NanoSomix Inc. (US), ThermoFisher Scientific Inc. (US), YMIR Genomics LLC and System Biosciences Inc. (US).
By highlighting EV research, we provide a document that can be used to stimulate support for these novel approaches and provide the basis for increased interaction with the pharmaceutical industry and collaborators in neuroscience, molecular biology, cell and gene therapy, and imaging. Accelerating the maturation of EV studies, in turn, allows for better communication among scientists of different backgrounds and provenances, as well as between academia and industry, for an effective translation of bench discoveries to clinical translation.
These interactions have been limited by a “myopic” view of this new discipline. Thus, grant reviews may lack rosters with EV scientists, early-stage angel investing may lack EV-knowledgeable financiers, and Pharma interest may depend on “marketing divisions” with minimal knowledge of vesicular applications. By documenting the growth of the EV field, we hope to encourage a roster of independent authorities skilled to provide peer review of manuscripts, evaluation of grant applications, support of foundation initiatives and corporate long-term planning. Individuals with these capacities exist but are not easily identified using common search engines.
The progress of EV research recapitulates many of the historical throes of the field of immune regulation. During the 1960s and 1970s, it became apparent that, in the immune system, T-cells play a singular role in the regulation of B-cell function and, second, that the T-cell compartment is heterogeneous, containing many subsets [Citation15]. These discoveries, bolstered by enumeration and sequestration studies of T- and B-cell subsets, in turn advanced cancer research [Citation16]. Seminal advances followed and influenced vaccination, the use of monoclonal antibodies in therapy and the development of antibody-‐based drugs as well as immunomodulatory techniques to the benefit of patients with immune disorders, and those with transplantation complications [Citation16–Citation18]. These knowledge milestones of immune regulation offer a roadmap to the design of exosome-based treatment. Consequently, T- and B-cell functions are now each being reassessed in the light of EV research. Recent discovery of antigen-specific exosomes offers hope for developing engineered exosomes able to precisely target regulatory molecules relevant to areas of pathology, all while minimizing side effects in other areas of the brain or body [Citation4,Citation5,Citation10,Citation11,Citation19]. The potential to create relevant biomarkers would render benefit towards patients who could benefit from immune therapies [Citation19–Citation21]. Thus, EV research will further identify diseases biomarkers and offer development of diagnostic tools that could distinguish and stratify disease subpopulations and enable personalized treatment protocols.
Methods
To calculate growth metrics of the EV field, we used online databases and platforms to index publications, patents and government-funded grants within the last decade. We plotted the changes in memberships to societies concerned with vesicular research, along the geographic locations of subscribers. Finally, we discuss global market trends for exosome diagnostics and therapeutics, as well as profile select companies whose major focus is on exosomes.
Publications
The international Scopus and Web of Science Core Collection (WoS) indices were examined to provide metrics of quality and quantity of exosome scientific research. WoS comprises seven online databases inclusive of 12,000 worldwide journals, including Open Access journals, and 150,000 conference proceedings. Publication and Citation counts for articles were obtained from Scopus, the largest abstract and citation database of peer-‐reviewed literature (journals, books and conference proceedings), which indexes over 15,000 journals from over 4,000 international publishers.
Search terms consisted of key words for exosome research. These terms included “exosome” and “microvesicle”. These terms were then juxtaposed to analytic terms relevant to exosome research including: “Nanoparticle tracking analysis” (NTA), “resistive pulse sequencing” (RPS), “flow cytometry”, and “enzyme-linked immunosorbent assay”. Terms were also juxtaposed to analytic terms relevant to biological substrates including: biofluids (“plasma”, “serum”, “CSF”, “urine”) and against cell types (“erythrocyte”, “platelet”, “lymphocyte”, “neutrophil”, “neuron”, “glia”, “cardiomyocyte”, “endothelial cell”), and representative diseases (“cancer”, “heart disease”, “infection”). For these search terms we calculated the changes in both rates of publication and citations for both exosome research terms and analytic terms.
The Scopus results were arranged into seven categories: year, source, author, affiliation, country or territory, document type and subject area. Journals containing key words were then sorted according to each journal’s ranking (SJR). This provided insight into a journal’s “influence” upon the subject field, the likelihood of acceptance of the concept and of citation of the article. We calculated each source’s “Normalized Impact per Paper” (SNIP), a measure of the contextual citation impact = a weighted measure based upon the total of citations in a subject field. From SNIPs, we were able to compare the sources in different subject fields and presented the ratio between the mean citation count per paper for each source compared with the citation potential of its subject field. The SNIP indices (1) consider the frequency with which authors cite papers and balances fields with heavy citation use and those with less frequent use, (2) weigh the speed of maturation of the citation impact, and (3) weigh the extent to which the field’s literature is included within the database. In addition, we utilized the (4) Scopus CiteScore, a measure of the citation impact of journal articles and other sources. We included the (5) Impact Factor distribution for articles and identified those with one of more citations. It should be noted that Scopus and JCR selectively cover journals. Google Scholar Metrics (GSM), is differently refined and provides search terms identified in the ten journals with the highest number of relevant citations. The GSM “h-index” is a metric of scientific productivity and impact based upon the number of citations bestowed upon the researcher’s most cited papers. The “h-index” discounts disproportions imposed by two extremes: highly cited papers and papers that have not yet been cited. An “h-index” of 20 means there are 20 items that have 20 citations or more.
Patents and intellectual property
The USA Patent and Trademark Office (USPTO) is an agency of the US Department of Commerce that issues patents for inventions, trademark registrations for products and intellectual properties. Patents (other than Provisional Patents) as intellectual property relevant to exosome research were tracked using USPTO’s patent database. Search terms employed include “exosome”, “microvesicle”, and “extracellular vesicle”. These were then juxtaposed to the previously used analytic technology terms.
Government funding
The US National Institutes of Health (NIH) of the Department of Health and Human Services is the largest biomedical research agency in the world. We used the NIH Federal Reporter database, to identify the grant applications funded with the key words: “exosome”, “microvesicle”, and “extracellular vesicle”. These were juxtaposed to the technology “NTA”. These data were then reported by NIH funding agency.
Global market trends for exosome-based diagnostics and therapeutics
We recorded BCC Research’s data regarding global market trends within their “Exosome Diagnostics and Therapeutics: Global Markets”. The authors highlight the main segments of the exosome industry and market trends.
US companies focused on exosomes
Dates of incorporation and current market value of companies with major intent focused on exosomes were documented by researching online resources. Companies profiled in this paper include Aethlon Medical, Inc. (US), Exosome Diagnostics Inc. (US), NanoSomix Inc. (US), ThermoFisher Scientific Inc. (US), YMIR Genomics LLC and System Biosciences Inc. (US).
Results
Publications and frequency of EV terms since FY2000
Articles published as indexed by JCR/WoS since 2000 are shown in Figure 1(a). WoS includes over 12,000 of the highest impact journals worldwide. Of English-language articles (2000–2016), 5,480 noted “exosome(s)” and/or “microvesicle(s)”. For the entire period of publications, 182,845 citations containing key words appeared (). The mean number of citations for each article was 33.37, yielding an “h-index” of 176. The h-index reflects the productivity and citation impact of the publications of a scientist and is an author-level metric based on the scientist’s most cited papers.
Figure 1. Evolution of EV articles and citations (source: Web of Science/Journal Citation Reports). Bar graphs indicate: (a) number of articles published (5,480) and (b) the number of times key words were cited (182,845) each year for the study period (2000-2016). (c) Data from graph A as a table to demonstrate increasing record counts per year, culminating in 20.47% of records published in 2016.
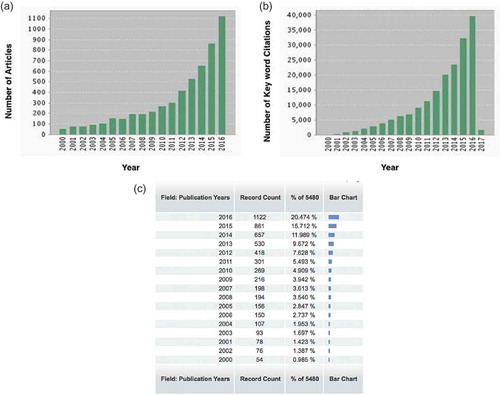
For each year, there was a successive rapid increase in publications and citations. Indeed, one-fifth of all EV and microvesicle records included in the timespan were published in the year 2016 alone ().
Plos One is the journal with the highest count of English-language EV-relevant publications (~5.78% of all records). A second publication site was the Journal of Biological Chemistry followed by Scientific Reports, Oncotarget and Journal of Immunology (Figure 2(a)). There was an annual rise in counts for the targeted concept, Journal of Extracellular Vesicles (JEV), the official scientific journal of the International Society for Extracellular Vesicles (ISEV) (). No other journal appeared to encourage EV-distinct publications.
Figure 2. Titles of source journals for data (source: Web of Science/Journal Citation Reports). (a) 10 journals holding the highest number of articles containing EV-key words indexed by JCR/WoS; (b) citations of articles in Journal of Extracellular Vesicles (JEV) throughout the study period (2000–2016).
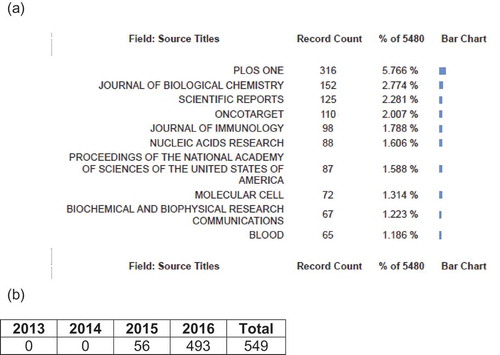
JCR/WoS analyses emphasize publications (38.1% of all records) from the USA; followed by China (11.8%), Germany (10.1%) and France and Japan (7%). We did not speciate by year the significant contribution of the European countries commencing prior to FY2000 – contributions well known by the scientific community. A total of 68 countries and/or territories have published EV-related articles. Uncertain is whether these geographic trends reflect broad governmental initiatives (as in the US NIH EV RFP solicitations) or represent investigator-initiated studies. One well-spring of activities appeared to be academic-based initiatives. Of 1,471 academic or research organizations (JCR/WoS), Harvard University represented approximately 2.8% of all records, with a mean each of 62 key word results; followed by Oxford University, the French National Centre for Scientific Research, and the Mayo Clinic.
We attempted to examine surrogates of exosome publications highlighting diagnostic and therapeutic technologies. By juxtaposing EV and technology terms, 603 articles were identified (Figure 3(a)) including 16,038 occurrences (). The number of citations per published cross-referenced term was 26.6, yielding an h-index of 63. We delved into the major topics of EV publications. Three major EV themes involved: (1) biofluids (2,002 articles (Figure 4(a)) with 69,250 citations (); (2) cell types, (1,699 articles () with 59,185 citations (); and (3) token diseases (1,819 articles () with 51,913 citations (). In general, there were fewer search results obtained from juxtaposing key words with key analytic terms than from searching key words alone.
Figure 3. Evolution in the number of EV articles and citations (source: Web of Science/Journal Citation Reports). Bar graphs provide: (a) number of articles published (509) as well as the (b) frequency of key words juxtaposed to terms of analytic technology (10,963) for the study period (2000–2016).
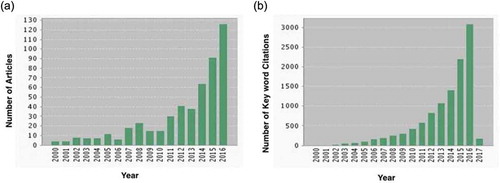
Figure 4. Evolution in the number of EV articles and citations (source: Web of Science/Journal Citation Reports). Bar graphs of (a) the number of articles published (2,002) as well as (b) the number of times key words were juxtaposed to analytic biofluid terms (69,250); (c) number of articles published (1,699) as well as (d) the number of times key words were juxtaposed to analytic cell type terms (59,185); (e) number of articles published (1,819) as well as (f) the number of times key words were juxtaposed to analytic token disease terms (51,913) of the study period (2000–2016).
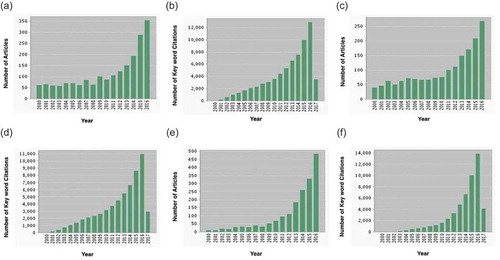
The frequency of combined EV terms over the last 16 years as indexed by Scopus () are similar to those reported by JCR/WoS.
The visual analyses provided by Scopus compare sources’ CiteScores, SJRs and SNIPs. In fact, journals with high CiteScores also have high SJRs and SNIPs. Molecular Cell is the journal with the highest CiteScore, SJR and SNIP; followed by the Proceedings of the National Academy of Sciences of the USA of America (PNAS). SNIP data reflect annual increases for most sources, especially Molecular Cell, PNAS, Blood and Nucleic Acids Research ().
There has been a general increase in global scientific publications against which are reflected exosome publications and citations. Bibliometric analysts Lutz Bornmann and Ruediger Mutz have estimated a general scientific publication rise of 8–9% each year, a doubling of global scientific output every 9 years [Citation22]. Here, we identify both the publication and citation rates, along with corresponding h-indices, within the field of EV to underscore the notion that this field is growing more rapidly than that of scientific publications in general. Our results for EV publications show approximate increases of 733-fold in the past 9 years – a trajectory of activity analogous to that of prior fields including T-cell subsets, circulating B lymphocytes and circulating tumour cells. Averaged on an annualized basis, this yearly rise of EV-related publication is 81% (). Notably, out of the total 5,480 EV-related articles published within our designated timespan, an estimated 20% were published in 2016 alone – in mark contrast to the ~1% that were published in the year 2000 () as an added emphasis on the recent “surge” in EV research. Similarly, when we associated key words with citations, we observed a 1000-fold increase in the last 9 years, equating to a mean 111% annual increase in citations containing EV-related terms in each successive year (). Furthermore, when we narrowed the focus on the only journal encouraging EV-distinct publications, the number of EV-related citations of articles in the JEV throughout the study period also increases on an annual basis – jumping 10-fold from 56 citations in 2015 to 493 citations in 2016 alone (). Albeit yielding fewer records in total for the allotted time period, searches juxtaposing key words with relevant analytic terms (i.e. key technology, biofluid, cell type or token disease) nonetheless demonstrated significant annual increases. When EV-related terms were meshed with key technologies, searches revealed 40% more publications () and 40% more citations () in the year 2016 compared with in 2015; similar percentage increases were observed when key words were crossed with analytic biofluid (), cell type () and token disease () terms. When comparing 2016 to 2015, predominating percentage increases were observed when EV-related terms were crossed with analytic token disease terms (48% more publications and 40% more citations), followed by analytic cell types (31% more publications and 25% more citations) and finally analytic biofluids (21% more publications and 30% more citations). We cannot predict that EVs will become a “nodal” field with established card-carrying scientists and multiple levels of collaborations and proprietary journals. Indeed, prior publications may not relate to the ultimate establishment of a field. These publications may “light up” and then fizzle as the field either evaporates or melds into another rubric. In a similar vein, a scientist’s publications, citations and h-index define neither his colleagues’ view of the ultimate “field” in which he studies nor the acceptance of that field. There is a need for other statistical normalization. One approach is to approximate “research fronts” – clusters of papers frequently cited together as a vantage point from which to watch science unfold hinging upon the cognitive and social connections forged as investigators cite others. Research fronts appear when science attains a novel level of activity and coherence, and investigators belong to an “invisible college”. Clarivate Analytics’ Research Fronts 2016 identifies trends among the top 10 research fronts. Research fronts draw on Essential Science Indicators data from 2009 to 2015. The report’s main field of clinical medicine features three research front groups, namely “drug resistance mechanisms and genetic monitoring”. Ranked fifth research front in clinical medicine, “circulating tumour DNA for the detection of tumours acquired resistance” corresponds to a major proponent of EV research. Moreover, the report identifies emerging research fronts within the same research areas. It identifies 18 emerging research fronts in biological sciences, which cover a series of topics, such as the genetic basis of important disease and the application of nanotechnology in biomedicine. Among selected topics, research in “nanotechnological carriers for cancer chemotherapy” – which include vesicular and particulate systems such as liposomes – ranks tenth on the list. Regarding clinical medicine, 21 emerging research fronts were selected. Of the six fronts focusing on cancer, “mechanisms of long non-coding RNA promoting cancer cell proliferation and metastasis” ranks seventh.
Patents and intellectual property
EV-related patents (UTSPO’s database) increased in growth over the past decade (Figure 7(a)). Since 2000, a total of 524 US patents cited “exosome(s)”, “extracellular vesicle” and/or “microvesicle(s)”. The largest rise, 160%, was observed between the years 2012 and 2013. When juxtaposed with key technology terms, 14 patents were identified (). Although the number of search results obtained from crossing key words with key technologies was significantly lower than that obtained from simply searching key words alone, the trends in terms of patent number growth rates per successive year are the same.
Figure 5. Evolution in the number of articles and citations (source: Scopus): line graph of the frequency of combined EV terms cited over the last 16 years (59,185).
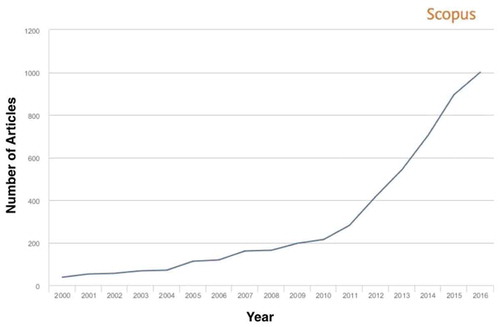
Figure 6. Scopus visual analyses (for the top 10 journals with the highest record counts). Visual analyses provided by Scopus depict line graphs relative to sources: (a) CiteScore, a measure of the citation impact of sources including journal articles; (b) SJR (source: Journal Ranking), which provides insight into a journal’s “prestige”, which influences the subject field, journal citation quality and reputation; and (c) SNIP (source: Normalized Impact per Paper), which more or less demonstrates annual increases for the majority of sources, particularly the journal Molecular Cell, which stands at first place. Generally, journals with the highest CiteScores (A) also corresponded to journals with the highest SJRs (b) and SNIPs (c). Trends depicted are consistent: Molecular Cell as being the journal with the highest CiteScore, SJR and SNIP since the year 2000; followed by the Proceedings of the National Academy of Sciences of the USA of America. Journals occupying subsequent places include Proceedings of the National Academy of Sciences of the USA, Blood and Nucleic Acids Research (respectively).
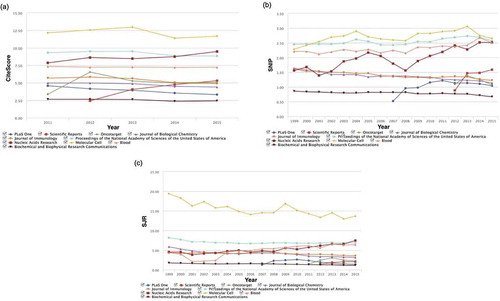
Government funding
The evolution of grants indexed by NIH Reporter is shown in Figure 8(a). Since 2000, a total of 948 NIH grants cited “exosome(s)” and/or “microvesicle(s)”. Successive increases were identified; culminating in a 201% increase in 2016. When juxtaposed with the key technology term “NTA”, 52 grants were identified (). Although the number of search results obtained from crossing key words with one of many key technologies was significantly lower than that obtained from simply searching key words alone, the trends in terms of grant number growth rates per successive year are the same.
Global market trends for exosome diagnostics and therapeutics
More than 100 registered clinical trials were gleaned from Smithsonian and EEC data; EVs constitute a novel platform for diagnosis [Citation23]. Various clinical opportunities are exponentially increasing owing to the strong growth in research focusing on exploring circulating miRNA modulation in disease and the various pharmaceutical companies looking to drive miRNA therapeutics into clinics [Citation6,Citation23]. Currently, there 35 clinical trials are underway relating cancer to exosomes – approximately two-thirds of those 35 trials relate to diagnostics, and the rest to therapeutics. The technology has spurred and been spurred by companies focused on exosomes. The global market for exosome diagnostics and therapeutics is projected to grow from $16.1 million in 2016 to 111.8 million in 2021 – a five-‐year compound annual growth rate (CAGR) of 47.3% [Citation14]. Within this market, diagnostics represent the largest sector and is expected to grow from $10.0 million in 2016 to $100.0 million in 2021 at a CAGR of 58.5% for the period [Citation14]. Major factors contributing to this growth include increased demand for the use of exosomes as biomarkers in the diagnosis of cancer, as well as the increased prevalence of cancer because of the rise in ageing population and lifestyle changes [Citation14]. Based on the sample type, blood as biofluid reflects the highest growth potential, with over 93% of the market share by revenues. However, the urine sample segment is anticipated to witness noticeable growth throughout 2016 [Citation14]. Therapeutics, on the other hand, is expected to grow from $5.0 million in 2016 to $10.0 million in 2021 at a CAGR of 14.9% [Citation14]. The inertia in this market reflects indecision of the FDA and therapeutic authorities. Thus, it is likely there will emerge therapeutics within countries with “fast-track” EV approval authorities including Italy, Korea and China. At present, North America accounted for more than half of the global exosome diagnostic and therapeutic market share in 2016 [Citation14].
The growth of the global exosome diagnostic and therapeutic market reflects global and country-wide emphases on cancer diagnosis and personalized therapy, government funding for exosomal research and commercial and orphan advocacy group imperatives to clinical trials [Citation24]. Their interest is strengthened by advances in techniques used in exosome isolation, analytical procedures, and innovative and advanced applications of exosomes. Other prominent growth factors include increasing preference and cost-adjusted decision-making by oncologists towards non-invasive diagnostic procedure for detecting cancer [Citation14,Citation24]. By contrast, strict government regulations and uncertainties as to the biologic and therapeutic definitions of EV therapies pose challenges to EV-market growth.
FDA approvals for the various non-invasive diagnostic tests to detect cancer are expected to define the regulatory landscape for liquid biopsy tests. Companies in the liquid biopsy market space are expected to invest more on test kits and cost-containment procedures to provide innovative diagnostic solutions to the oncologists and physicians for detecting metastasis [Citation12].
US companies with major intent focused on exosomes
Companies are funnelling large sums of money towards internal research into biomarkers, diagnostics and therapeutics. The traditional method used for exosome isolation is differential ultracentrifugation [Citation25]. The result is a crude exosome preparation that is suited for functional assays or biomarker discovery. Alternative approaches bypass labour-intensive processes and substitute automated, high-throughput approaches. We cite several examples without being comprehensive, judging relative efficacy or excluding preparative kits. RNA from exosomes can be prepared directly using a spin-column-based exosomal RNA isolation kit. Also based on spin-column concentration, there are RNA enrichment kits as well. There are also novel, fast and low-cost protocols for isolating EVs and/or exRNA from urine that gives a higher yield than current commercial kits or ultracentrifugation. On the other hand, total exosome isolation reagent lines enable collection with a rapid spin by using a proprietary polymer that causes the vesicles to precipitate. Additionally, researchers can collect specific exosomal subsets by using antibody-conjugated beads. In a similar vein, the 10 major device companies, skilled in flow technologies for cell analyses, have explored adaptations to EV studies towards “liquid biopsy” and longitudinal monitoring of personalized therapies. EVs are analysed using NTA, TRPS, SPR, atomic force microscopy, cryo-electron microscopy and others [Citation26], and device manufacturers are providing flow cytometry approaches for quantitative particle-by-particle multiplex analyses of EVs [Citation27]. Flow-cytometry resolution can approach 20 nm, and reagent EV and antibody standards are emerging to enable replication studies. In anticipation of improved flow-cytometry instrumentation and reagents, a broad scientific community effort on standardization of flow cytometry approached for EV analysis is already underway, led by ISEV-ISAC-ISTH EV flow-cytometry workgroup. Cooperative standards for reporting have been a mainstay of the International Society ISEV. Their workshop “evRNA analysis and bioinformatics” provided guidelines to consider when performing analysis [Citation28]. These were further expanded as minimal information for studies on EVs (MISEV) guidelines [Citation29]. Researchers developed the EV-track crowdsourcing knowledgebase, which contains records of experimental parameters of EV-related studies [Citation30]. The EV-METRIC, created by extracting nine relevant experimental parameters and condensing them into a single metric, describes the type of information necessary to interpret and reproduce EV experiments. The field will be expanded by compendia of the molecular content of different types of EVs. Examples of integrated databases providing high-throughput datasets of vesicular components include EVpedia [Citation31], Vesiclepedia [Citation32] and Exocarta [Citation33].
In sum, the EV/exosome “toolbox” is filling rapidly, and those tools are powering exosome research at a furious pace. With exosome-based diagnostics just over the horizon, it is paramount to encourage growth in the EV-field to further research exosomes’ potential in diagnosing and treating human disease.
Acknowledgements
The authors acknowledge and thank Dr Stephen Gould, Jordana Serero (MD candidate at McGill University), for her review of the manuscript, and Sergei Gulnik, who read the manuscript and commented on analytics. Supported by Carter/Hochberg NIH: 5UH3TR000931-04
Disclosure statement
Dr. Hochberg is President of CASCENT Inc. and TAVEC Inc (both exosome therapeutic companies without conflict).
Additional information
Funding
References
- Merchant ML, Rood IM, Deegens JK, et al. Isolation and characterization of urinary extracellular vesicles: implications for biomarker discovery. Nat Rev Nephrol. 2017. DOI:10.1038/nrneph.2017.148
- Pap E, Falus A, Buzás EI. Membrane vesicles, current state‐of‐the‐ art: emerging role of extracellular vesicles. Cell Mol Life Sci. 2011;68(16):2667‐88.
- Simons M, Raposo G. Exosomes‐vesicular carriers for intercellular communication. Curr Opin Cell Biol. 2009;21(4):575‐81.
- Corrado C, Raimondo S, Chiesi A, et al. Exosomes as intercellular signaling organelles involved in health and disease: basic science and clinical applications. Int J Mol Sci. 2013;14(3):5338‐66.
- Théry C, Ostrowski M, Segura E. Membrane vesicles as conveyors of immune responses. Nat Rev Immunol. 2009;9(8):581‐93.
- Krebs MG, Hou J-M, Ward TH, et al. Circulating tumour cells: their utility in cancer management and predicting outcomes. Ther Adv Med Oncol. 2010;2(6):1–11.
- Liga A, Vliegenthart AD, Oosthuyzen W, et al. Exosome isolation: a microfluidic road-map. Lab Chip. 2015;15(11):2388–2394.
- Pugholm LH, Revenfeld ALS, Søndergaard EKL, et al. Antibody-based assays for phenotyping of extracellular vesicles. Biomed Res Int. 2015;2015:524817.
- Yuana Y, Böing AN, Grootemaat AE, et al. Handling and storage of human body fluids for analysis of extracellular vesicles. J Extracell Vesicles. 2015;4:29260.
- Perakis S, Speicher MR. Emerging concepts in liquid biopsies. BMC Med. 2017;15:75.
- Gold B, Cankovic M, Furtado LV, et al. Do circulating tumor cells, exosomes, and circulating tumor nucleic acids have clinical utility? A report of the association for molecular pathology. J Mol Diagn. 2015;17(3):209–224.
- 2016 Market research report by grand view research; liquid biopsy market size & forecast by application (Therapy Selection for Metastatic Breast Cancer (MBS), Therapy Selection for Other Metastatic Cancers, Molecular Monitoring, Molecular Monitoring), By Sample Type (Blood, Urine, Tissue & Saliva), By Biomarker Type (Circulating Tumor Cells (CTC), Circulating Tumor DNA (ctDNA), Exosomes), By Technology (Single Gene Analysis (PCR Microarrays), Multi-gene-parallel Analysis (NGS)) and trend analysis from 2013 – 2030. http://www.grandviewresearch.com/industry-analysis/liquid-biopsy-market
- Munson P, Shukla A. Exosomes: potential in cancer diagnosis and therapy. Medicines (Basel). 2015;2(4):310–327.
- Tcherpakov M. Exosome Diagnostics and Therapeutics: Global Markets. Wellesley, MA: BCC Research; 2016.
- Alberts B, Johnson A, Lewis J, et al. Molecular biology of the cell. 4th. New York: Garland Science;2002. Helper T Cells and Lymphocyte Activation. Available from: https://www.ncbi.nlm.nih.gov/books/NBK26827/
- Scott AM, Allison JP, Wolchok JD. Monoclonal antibodies in cancer therapy. Cancer Immunity. 2012;12:14.
- Chames P, Van Regenmortel M, Weiss E, et al. Therapeutic antibodies: successes, limitations and hopes for the future. Br J Pharmacol. 2009;157(2):220–233.
- Weiner LM, Surana R, Wang S. Antibodies and cancer therapy: versatile platforms for cancer immunotherapy. Nat Reviews Immunol. 2010;10(5):317–327.
- Kawikova I, Askenase PW. Diagnostic and therapeutic potentials of exosomes in CNS diseases. Brain Res. 2015 Aug 18;1617: 63–71. DOI:10.1016/j.brainres.2014.09.070.
- Braccioli L, van Velthoven C, Heijnen CJ. Exosomes: a new weapon to treat the central nervous system. Mol Neurobiol. 2014 Feb;49(1):113–119. DOI:10.1007/s12035-013-8504-9.
- Rapisuwon S, Vietsch EE, Wellstein A. Circulating biomarkers to monitor cancer progression and treatment. Comput Struct Biotechnol J. 2016 Jun 1;14: 211–222. DOI:10.1016/j.csbj.2016.05.004.
- Van Noorden R (2014, May 7). Global scientific output doubles every nine years. [cited 2017 Mar 01]. Available from: http://blogs.nature.com/news/2014/05/global-scientific-output-doubles-every-nine-years.htm
- Igaz P. Circulating microRNAs in disease diagnostics and their potential biological relevance. 1. Basel (Switzerland): Springer; 2015. p. 46–49.
- Naito Y, Yoshioka Y, Ochiya T. The functional role of exosomes in cancer biology and their potential as biomarkers and therapeutic targets of cancer. Gan To Kagaku Ryoho. 2015 Jun;42(6):647–655.
- Li P, Kaslan M, Lee SH, et al. Progress in exosome isolation techniques. Theranostics. 2017;7(3):789–804.
- Szatanek R, Baj-Krzyworzeka M, Zimoch J, et al. The methods of choice for Extracellular Vesicles (EVs) characterization. Int J Mol Sci. 2017;18(6):1153.
- Koliha N, Wiencek Y, Heider U, et al. A novel multiplex bead-based platform highlights the diversity of extracellular vesicles. J Extracell Vesicles. 2016;5(1):29975.
- Stevanato L, Thanabalasundaram L, Vysokov N, et al. Investigation of content, stoichiometry and transfer of miRNA from human neural stem cell line derived exosomes. Plos One. 2016;11(1). DOI:10.1371/journal.pone.0146353
- Wen C, Seeger RC, Fabbri M, et al. Biological roles and potential applications of immune cell-derived extracellular vesicles. J Extracell Vesicles. 2017;6(1):1400370.
- Deun JV, Mestdagh P, Wever OD, et al. Abstract LB-107: EV-TRACK: transparent reporting and centralizing knowledge of extracellular vesicles to support the validation of extracellular vesicle biomarkers in cancer research. Cancer Res. 2017;77(13 Supplement). DOI:10.1158/1538-7445.am2017-lb-107
- Kim D, Lee J, Simpson RJ, et al. EVpedia: A community web resource for prokaryotic and eukaryotic extracellular vesicles research. Semin Cell Dev Biol. 2015;40:4–7.
- Kalra H, Simpson RJ, Ji H, et al. Vesiclepedia: a compendium for extracellular vesicles with continuous community annotation. PLoS Biol. 2012;10(12). DOI:10.1371/journal.pbio.1001450
- Mathivanan S, Fahner CJ, Reid GE, et al. ExoCarta 2012: database of exosomal proteins, RNA and lipids. Nucleic Acids Res. 2011;40(D1). DOI:10.1093/nar/gkr828