ABSTRACT
Through traditional medicine, there were diseases and disorders that previously remained untreated or were simply thought to be incurable. Since the discovery of mesenchymal stem cells (MSCs), there has been a flurry of research to develop MSC-based therapy for diseases and disorders. It is now well-known that MSCs do not typically engraft after transplantation and exhibit their therapeutic effect via a paracrine mechanism. In addition to secretory proteins, MSCs also produce extracellular vesicles (EVs), membrane-bound nanovesicles containing proteins, DNA and RNA. The secreted vesicles then interact with target cells and deliver their contents, imparting their ultimate therapeutic effect. Unlike the widely studied cancer cells, the yield of MSC-exosomes is a limiting factor for large-scale production for cell-free therapies. Here we summarise potential approaches to increase the yield of such vesicles while maintaining or enhancing their efficacy by engineering the extracellular environment and intracellular components of MSCs.
Introduction
Regenerative medicine itself was a novel idea: using different cell types to restore any damaged, ageing or lost tissue of the human body. The earliest application of cellular therapy would date back to World War I, where blood transfusions were used to save wounded soldiers’ lives, eventually leading to the discovery of hematopoietic stem cells and then giving rise to stem cell-based therapies. To this day, there are over 6600 stem cell-related clinical trials underway in the USA alone and many more internationally (www.ClinicalTrials.gov).
Mesenchymal stem cells (MSCs) are multipotent cells that can differentiate into various cell types such as osteoblasts, chondrocytes and adipocytes in vitro [Citation1]. They can be obtained from several sources such as bone marrow, placenta, adipose tissue, amniotic fluid, and umbilical cord to name a few [Citation2]. They have been coined the “paramedics” of the human body, traversing to injury sites for tissue repair or wound healing [Citation3–Citation5]. In addition to their role in the tissue regeneration, MSCs are also immune privileged, having the ability to be introduced to an immune system without eliciting an inflammatory response. But there are risks factors involved when considering MSCs as a cell-based treatment. Due to the cells’ multipotency, there is the chance of tumorigenesis if the regulation of proliferation/differentiation were to dysfunction. Several studies have revealed a low engraftment rate of MSCs after transplantation; yet, their therapeutic effects remain long after implantation, suggesting that their effects are achieved through paracrine mechanisms rather than replacing the lost cells with newly differentiated cells [Citation6–Citation8]. For example, the conditioned medium (CM) from MSCs (MSC-CM) was observed to protect the cardiomyocytes by interrupting mitochondria-mediated cell death [Citation5]. Several studies have shown that by altering the culture environment of cells such as growing in low oxygen atmosphere [Citation9–Citation11] or adding biomolecules such as brain-derived neurotrophic factor mimetics or peptides [Citation12–Citation14] could lead to improved functional effect of the CM. Now it is recognised that not only do the MSC-CM comprise of cytokines and growth factors, but it also contains extracellular vesicles (EVs) that may play a key role in imparting these effects [Citation9–Citation11,Citation15–Citation17].
Overview of extracellular vesicles
EVs were thought to function solely as the “dumpster” of cells, a way to dispose of unwanted proteins and other molecules, but they have now emerged as one of the significant mediators in cell–cell communication for many processes such as immune response, angiogenesis, proliferation and differentiation [Citation5,Citation6,Citation8,Citation18–Citation21]. There are three widely known EVs: apoptotic bodies, microvesicles and exosomes. The apoptotic bodies can be the largest, with their diameters ranging from 800 to 5000 nm, while microvesicles are smaller, between 50 and 1000 nm. Both of these EVs have similar biogenesis as they bleb from the cell plasma membrane. The difference is that the apoptotic bodies bleb from apoptotic cells while the microvesicles bleb from living cells. The blebbing of the apoptotic bodies occur indiscriminately from the apoptotic cells and can contain fragmented nuclei, proteins, DNA, miRNA to name a few. The blebbing of the microvesicles follow an orderly pattern: trafficking of cellular molecules to the plasma membrane that result in protrusion of the cell’s surface, budding then detaching from the membrane. Exosomes are the smallest, with sizes falling between 30 and 150 nm, and are generated within the cells through the endolysosomal pathway ().
Figure 1. Biogenesis and release of extracellular vesicles, both microvesicles and exosomes (A). The contents of the exosomes are shown as well (B).
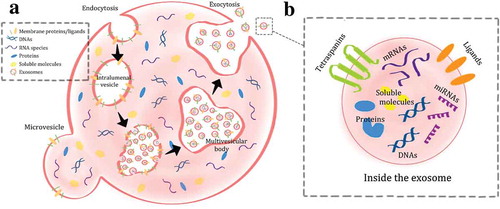
The pathway begins at the plasma membrane, where the membrane folds inward and then pinches off an empty intraluminal vesicle [Citation5,Citation6,Citation8,Citation22–Citation26]. The process of endocytosis continues on the membrane of the intraluminal vesicle, creating smaller vesicles known as exosomes. The empty intraluminal vesicle then matures into a multivesicular body, where exosomes accumulate. The multivesicular body can either fuse with the cell’s plasma membrane and release those exosomes, or mature further into a lysosome, one of the many essential organelles of a cell [Citation22–Citation26]. This endolysosomal pathway leading to the generation and release of exosomes is regulated by a well-defined mechanism such as the endosomal sorting complex required for transport (ESCRT) or the Rab proteins [Citation22–Citation26]. At the same time, the exosomes encapsulate the MSCs’ cytoplasmic contents of proteins, DNA and RNA.
Isolation of extracellular vesicles
There are many ways of isolating and purifying EVs from the CM, each with its own pros and cons. The methods can include the ultracentrifugation, precipitation, immunoaffinity, size exclusion chromatography and ultrafiltration [Citation25,Citation27–Citation29]. Ultracentrifugation is considered the most efficient way of obtaining EVs from samples through several centrifugation steps of increasing speed. Advantages include low cost and the ability to spin large volumes, but biggest disadvantage is that the pellet may contain non-EV contaminants (i.e. lipoprotein complexes or cellular debris) since the method does not specifically discriminate between the EVs from other particles (i.e. cellular debris), especially of similar densities (i.e. lipoprotein complexes) [Citation30]. Additional steps are usually added in the process to obtain a more homogenous population such as density gradient, use of a column or ultrafiltration [Citation25,Citation27–Citation29]. Precipitation is the use of a precipitating agent to induce the clumping of EVs that is usually sedimented by centrifugation. It is considered a concentration method, hence the starting volume can be low, but the introduction of a synthetic material to the sample may not be ideal for downstream functional applications [Citation25,Citation27–Citation29]. Immunoaffinity is an isolation method that utilise the immobilised antibodies to capture the EVs containing a specific ligand. Immunoaffinity is commonly used as a purification step because it is not compatible with large sample volumes and samples with low EVs must be concentrated first [Citation25,Citation27–Citation29]. Size exclusion chromatography and ultrafiltration have similar working principles, where the EVs are separated based on size and that is achieved with Sepharose beads or membrane filter although each method have its own advantages and disadvantages [Citation25,Citation27–Citation29]. Size exclusion chromatography can reduce the EV aggregates that are common with other isolation protocols but the resulting EV samples are very dilute, requiring a second step of re-concentration. Ultrafiltration utilises force to push the sample through the filter and is often used in combination with other techniques as it is more time efficient than centrifugation methods; however, there is the potential loss of EVs due to trapping in the pore membrane or non-specific binding of EVs to the membrane (). While each isolation technique comes with its own limitations, there are ways to overcome those limitations and recommendations have been proposed previously [Citation25,Citation27–Citation29].
Figure 2. Common methods in isolating the extracellular vesicles. Ultracentrifugation is the method often utilised in experiments (A). Precipitation involves the use of an agent that allow for the pelleting of EVs (B). Ultrafiltration uses a filter to separate the EVs from the other molecules based off pore size in the filter (C). Immunoaffinity uses antibody to capture the EVs based on their surface markers (D). Size exclusion chromatography separate the EVs from the non-EVs molecules with a packed column of certain materials (E).
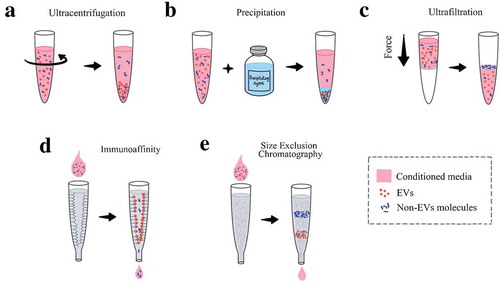
MSC-derived exosomes
MSC-derived exosomes (MSC-exosomes) have been used as a treatment for diseases and disorders of many systems such as the cardiovascular, neurological, musculoskeletal and immune systems [Citation5,Citation6,Citation8,Citation31]. Rats with traumatic brain injury exhibited significant functional recovery, increased neurite remodelling, angiogenesis and neurogenesis after treatment with exosomes [Citation32]. They can ameliorate liver fibrosis, exert protective effects against acute liver injury and may enhance the anti-tumour effect against hepatocellular carcinoma [Citation18]. MSC-exosomes have also been found to inhibit the migration of inflammatory cells, thus providing a benefit for the autoimmune disease uveitis [Citation33]. Plenty of studies have shown their therapeutic use; however, one caveat is that the generated exosomes are not a uniform population [Citation34,Citation35]. Their composition can vary depending on many factors such as the culture environment, isolation procedures and their cellular origin, thus leading to a variety of cytokines, DNA and RNA species being encapsulated by the exosomes. First, the purity of the exosomes must be established based off the isolation method. Polymeric precipitation of the exosomes would require some further purification [Citation18,Citation32] or the ultracentrifugation of exosomes may need some additional filtering [Citation33]. Different isolation or purification methods can also yield heterogeneous MSC-exosomes, making it difficult to compare proteomic studies between them. Second, the composition of exosomes is closely associated with the cellular health and condition of the secreting cells as different molecular signatures are found between exosomes derived from healthy, ageing, and diseased cells or their growth environment(s) [Citation36–Citation38]. Nevertheless, considering the complex production pathway, exosomes generated from MSCs in a well-defined culture environment could be a powerful therapeutic agent by themselves for cell-free therapy and circumvent the issue of immune rejection that can occur in live cell-based therapies.
Potential approaches to improve MSC-exosome yield and efficacy
A Good Manufacturing Practice-compliant protocol had been developed for obtaining MSC-derived EVs [Citation39]. Although MSC-derived EVs show promising application for regenerative therapies, their use is often largely limited by very low yield in routine culture conditions, especially the MSC-exosomes. Thus, there is a great need to develop approaches to improve their yield without sacrificing their functionality while, ultimately, enhancing their efficacy as well. A study showed exosomes isolated from neuron-CM-contained small RNA that were internalised into astrocytes to improve its signalling in amyotrophic lateral sclerosis mice model [Citation36]. Another study showed MSCs exposed to ischemic brain extracts had increased levels of miR-133b in its released exosomes [Citation38]. Therefore, refining the culture condition of MSCs will significantly influence not only the production yield but also the efficacy of MSC-exosomes in consideration to the proteomic and genomic complexities of exosomes [Citation40–Citation44]. The culture condition alone may not be enough to address the additional limitations on the efficacy of MSC-exosomes though. Exosomes derived from different cell types may have preferential targeting towards certain cell types based on their membrane composition thus imparting a differential effect on body systems [Citation37,Citation41,Citation45]. For example, oligodendroglia EVs are preferentially taken up by microglia [Citation36]. This could be addressed by exploiting the nature of exosomes as natural carriers of miRNA or other molecules by treating them as drug delivery vehicles as their counterparts, liposomes or extracellular nanovesicles, are currently utilised [Citation40,Citation42,Citation45,Citation46]. To exemplify this, modified exosomes containing therapeutic cargo are currently under use in clinical trials [Citation46].
Three-dimensional extracellular matrix-based scaffolds
MSCs are usually cultured on two-dimensional (2D) plastic tissue culture dishes and flasks, limiting the surface area for cell growth as well as cell–cell and cell–extracellular matrix interaction in the living environment, which does not truly represent the in vivo conditions. Native extracellular matrix (ECM) can be utilised to provide a three-dimensional (3D) environment for cell attachment and growth while synthetic biomaterial scaffolds can be engineered to mimic the ECM structure and functions. The native ECM can be developed by decellularising tissues to achieve a heterogeneous composition of extracellular molecules left from decellularised tissues. Cells can bind to those molecules via surface receptors, providing a direct interaction that could potentially influence their functionality. Functional EVs can also be derived from native ECM and promote cell functions [Citation47,Citation48]. The native ECM can come in the forms of a hydrogel, a sheet or a porous scaffold and they can be utilised for MSC culture to generate the EVs [Citation49] ().
Figure 3. The different forms of an extracellular matrix and their interactions with the cells. The tissue culture treated plastic surface is the most common surface for culturing the MSCs (A). The ECM sheet can be in form of decellularised native ECM or an artificial matrix, which can include different oriented fibres (B). A hydrogel can be manufactured from the decellularised ECM or a chemical mixture for culturing seeded or encapsulated MSCs (C). The porous scaffold is the most similar to the native environment of the MSCs, perhaps allowing the most suitable culture environment (D).
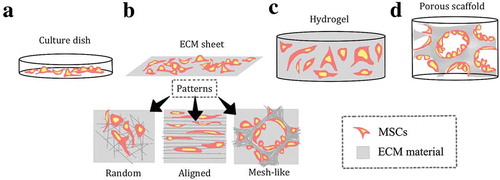
Scaffold structure could influence the functions of seeded cells. When a hydrogel, a porous scaffold of the alginate solution (derived from brown algae) and a tissue culture plastic surface were compared, it was found that the porous scaffold allowed the highest concentration of cytokines and growth factors produced by bone marrow MSCs (BM-MSCs) compared to the other surfaces [Citation49]. Concentrations of cytokines basic fibroblast growth factor 2, insulin-like growth factor, hepatocyte growth factor and leukaemia inhibitory factor were significantly higher in the CM derived from the porous scaffold compared to CMs derived from hydrogel and tissue culture surface. Such structure could also influence EV secretion and possibly their therapeutic potential since the MSCs are known to be responsive to the environmental stimuli. Similarly, it was shown that the Avitene Ultrafoam collagen hemostat caused the BM-MSCs to release twice the number of exosomes compared to the plastic surface culture based on the protein assay [Citation32]. Although the isolation method used in this study was precipitation with no further purification, the concept itself may be beneficial to finding a way to increase the MSC-exosome yield. Moreover, those exosomes derived from the 3D condition had greater therapeutic effects on the rat model of traumatic brain injury, causing superior cognitive functional recovery when directly injected into rat brains. Additionally, the matrix scaffold could also be used as a delivery vehicle for exosomes to achieve local and prolonged delivery to the wound area [Citation32]. In a diabetic rat model, the chitosan wound dressing was used as an exosome delivery vehicle over a course of 6 days, allowing for acceleration of re-epithelialisation, angiogenesis and collagen maturity of the wound sites [Citation32].
Unlike the native ECM, the synthetic matrices do not possess the functional molecules, but they can be modified by adding molecules in order to make more of a bioactive artificial ECM. This modification will also circumvent the potential issues of native matrices such as batch-to-batch or donor-to-donor variations. A variety of advanced engineering approaches have been used to produce native ECM mimicking structures. For example, an ECM mimicking nanofibrous scaffold could be created by electrospinning from polymer blends of polylactic acid (PLA) and polycaprolactone (PCL) [Citation31]. At the same time, such a scaffold could be potentially functionalised by presenting specific functional ligands to improve production of MSC-exosomes [Citation31]. Another advantage of the synthetic scaffold is the control over their structural pattern and composition. The fibres could be produced in random, aligned or mesh-like patterns, and these different patterns were shown to affect MSCs in terms of their secretome, such as increasing the production of anti-inflammatory and pro-angiogenic factors [Citation50]. Concentration of molecules prostaglandin E2, inducible nitric oxide synthases, tumour necrosis factor-stimulated gene 6 protein, transforming growth factor beta 1, hepatocyte growth factor and vascular endothelial growth factor increased significantly on the oriented fibres compared to the microplate [Citation50]. The production of EVs as an important part of secretome may be reflected by this approach as well. The composition of the synthetic scaffold could be adjusted to improve the production of EVs in MSCs. It was shown that by adding nitric oxide (NO) to a chitosan scaffold, the resulting nitric oxide-releasing chitosan scaffold caused placenta MSCs to release more exosomes with pro-angiogenic and pro-migratory factors compared to the normal human placenta MSCs [Citation47]. Specifically, vascular endothelial growth factor concentration and microRNA 126 expression levels were higher in the exosomes from the nitric oxide stimulated placenta MSCs. The ischemic muscle tissue was found to be extensively protected when those exosomes were used as a treatment in the murine hind limb ischemia model. Further studies are needed to discover more well-defined molecules to functionalise the synthetic scaffold that could induce MSCs to generate more defined populations of EVs with specific biological functions.
Large-scale cell expansion methods
As research continues to foray into utilising the 3D environment for cell culture, there also emerges the potential of expanding the cells within a small volume. By making use of ECM, the limited surface area can be maximised for its EV yield, but the number may still not be enough for large-scale needs for clinical use. Large volumes of media would be required to get a sizable number of vesicles from the MSC-CM that would meet clinical standards. Both the microcarriers and hollow-fibre bioreactors have larger surface area and are currently used for large-scale expansion of MSCs in a 3D environment [Citation51–Citation57] ().
Figure 4. The schematics of spinner flask containing microcarriers containing the cells (A) as well as the hollow-fibre bioreactor and its cross section with cells inside (B).
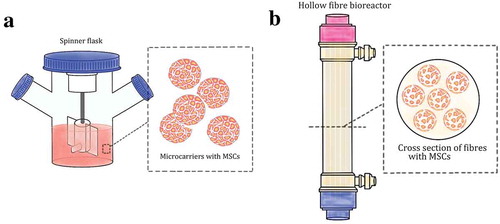
The microcarriers are tiny beads with many varying features such as material, pore size, and surface charge [Citation53]. The cells, adhered on microcarriers, are grown in spinner flasks that hold a pair of plastic baffles in an X formation propelled by a magnetic field, creating a dynamic environment. The flasks also come in different sizes, ranging from 100 mL to 3 L and are placed on stir plates within an incubator where gas exchange occurs by either the loosened side caps or by a gas line through the holes on the side caps. Using this method, the final cell yield is quite high and can be achieved within a shorter incubation time. MSCs cultured using this method show no change in differentiation capability and maintain a normal karyotype [Citation52–Citation55]. The CM collected from the suspension culture was shown to contain higher levels of secretory molecules, which increased the cell proliferation and neural differentiation when injected directly to the hippocampal dentate gyrus of rat brains [Citation55]. The CM also enhanced both the survival and differentiation rates of human neural precursor cells in culture [Citation52]. A drawback to this method is that the cells are much more metabolically active, thus more nutrients are consumed, and more wastes are produced, requiring frequent change of culture media and passaging. Nevertheless, this could be one of the promising methods to increase MSC-exosomes production with less culture media.
The hollow-fibre bioreactor is based on culturing the cells in the hollow and semi-permeable fibres [Citation51,Citation56,Citation57]. The fibres are tube-like and approximately 200 microns in diameter. They are sealed in a glass cylinder with inputs and outputs where media, waste and gas flow through the cylinder in one direction. The exchange of necessities that the cells require is achieved with the porous barriers of the fibres. Similar to the spinner flask, the hollow-fibre bioreactor can also achieve a higher cell yield in a short incubation time. Additional advantages would be less labour, as a computer could maintain the necessities, and less risk of contamination, as there is a significant decrease with open procedures. Studies have been completed to ensure that the hollow-fibre bioreactor would meet the Good Manufacturing Practice standards and the requirements of any clinical trial protocol [Citation51,Citation56]. By scaling up the cell culture properly, the exosome yield could increase without significantly compromising their functionality.
Utilising the biology behind the extracellular vesicle biogenesis
By utilising our current knowledge on the regulation of EV biogenesis, we can manipulate certain components that could lead to increased yield, particularly the exosomes via the endolysosomal pathway. By changing the intracellular milieu, we can potentially manipulate the production of exosomes to increase the final yield. For example, we can look at the cellular components involved in beginning, middle and/or end of the endolysosomal pathway, starting with the P2X7 receptors on the membrane. The activation of P2X7 receptors (P2X7R) in pulses on the membrane can trigger a number of cellular functions such as membrane blebbing, sorting of endosomal contents and fusion with the multivesicular body to release the exosomes [Citation58]. Another receptor of interest can be the soluble NSF attachment protein receptors (SNARES) which are required for fusion of the multivesicular body to the plasma membrane [Citation26,Citation59–Citation61]. There are also multipass membrane proteins that could regulate the exosome release such as the tumour suppressor activated pathway-6 (TSAP6), which is a p53-inducible gene. This was confirmed when TSAP6-null mice showed a severe compromised exosome secretion and their p53-dependent secretory pathway abrogated [Citation62,Citation63]. This pathway is also a substrate of a protein that provides negative feedback to exosome secretion, rhomboid domain containing 1 (RHBDD1) protein [Citation64]. The inactivation of the RHBDD1 protein prevented the cleavage of transmembrane proteins, products of TSAP6. These proteins are part of the exosome trafficking activity, perhaps through mediation of movement of the multivesicular body to the plasma membrane, therefore, preventing their cleavage could allow for more exosomes to be released. The modulating lipids may be of interest as well, such as the phospholipase D2 gene (PLD2). What PLD2 does is breaking down phosphatidylcholine into choline and phosphatidic acid, which is one of the important lipid secondary messengers involved in endocytosis and exocytosis processes. A study found that the overexpression of PLD2 led to twice the number of exosomes released from the cells while the knockdown of PLD2 led to twice the amount lower than exosomes released [Citation65]. Another component of the EV biogenesis that could be of interest is the multivesicular body fusion with the plasma membrane and its regulatory molecules such as the Rab protein family along with their GTPases [Citation66]. The Rab GTPases are known for their roles in membrane trafficking activity, which are part of the endosomal system. With the inhibition of the Rab35 protein, the cell has an accumulation of the endosomal vesicles and an impaired secretion mechanism [Citation66]. Evidently, the exosome secretion is compromised as well. Perhaps finding another release mechanism, such as Rab27a/b [Citation67], to compensate for the impaired secretion mechanism would allow for those accumulated EVs to be secreted out of the cells, leading to an increased yield.
Genetic packaging of the extracellular vesicles
By utilising our current knowledge on contents of the EVs especially exosomes, we can improve their efficacy such as changing what is packaged into them. This modification can be achieved by overexpression of certain proteins or have certain RNAs packed within the exosomes.
Sequences such as DNA or RNA have been shown to display neuroprotective or angiogenic properties. They can be packed inside exosomes or directed to the exosomes [Citation9,Citation68–Citation74]. For example, protein kinase b (Akt) has been shown to display angiogenic properties so when the AKT1 gene was transfected into MSCs, the exosomes derived from Akt-modified MSCs promoted endothelial cell proliferation, migration and new blood vessel formation in the rat model of myocardial infarction [Citation69]. Apart from activation of particular genes, overexpression of certain genes that are already present in MSCs also provides some benefits. For example, the hypoxia inducible factor-1α (HIF-1α) has been shown to have angiogenic functions and is present in MSCs [Citation9]. Upon the overexpression of HIF-1α, Jagged1 was found to be more abundant in exosomes derived from the same MSCs, leading them to be pro-angiogenic as well. This finding was proven when they were used as a treatment for the murine model of ischemia. Aside from the DNA sequences, RNA sequences could serve as powerful therapeutic agents. Certain miRNAs have been overexpressed in MSCs, causing an increase in their levels within exosomes and in turn enhancing their therapeutic potential. For example, the miR-30b and miR-126 were overexpressed in MSCs, and the exosomes derived from the culture were shown to promote angiogenesis in various ischemia models and in the diabetic wound model [Citation18,Citation32]. The exosomes derived from BM-MSCs with miR-133b overexpression were shown to significantly promote functional recovery in the rat model of stroke [Citation72]. There are also non-coding RNAs present in the MSC-exosomes that have regulatory roles in reparation of the damaged tissues [Citation68].
Conclusion
MSC-exosomes are currently a hot topic as much research is being conducted regarding their use as a treatment for a myriad of diseases and disorders. There are many advantages of using EVs, such as being a cell-free alternative therapy, providing easier administration for patients and possibly requiring less time for recovery. There are certain limitations though, one of which is the isolation and purification of the EVs. The resulting products remain heterogeneous, containing non-EVs contaminants or too little vesicles for treatment. The mechanism on how the EVs function as paracrine remains elusive as more research is looking into their specific contents and functions. With that being said, any treatment using exosomes would need to be evaluated extensively for safety and efficacy before clinical trials [Citation75]. A way to meet the potentially high demand for exosomes derived from different sources would also need to be developed. We herein have described ways to manipulate both the outside (extracellular) environment and the inside (intracellular) components of MSCs to increase the quantity of exosomes and/or to improve their quality, which will eventually lead to generating a uniform population of exosomes that can potentially be used for future clinical applications.
Acknowledgement
The authors thank N. Kreutzberg for assistance in editing.
Disclosure statement
No potential conflict of interest was reported by the authors.
Additional information
Funding
References
- Caplan AI. Mesenchymal stem cells. J Orthop Res. 1991 Sep;9(5):1–11. PubMed PMID: 1870029.
- Hass R, Kasper C, Bohm S, et al. Different populations and sources of human mesenchymal stem cells (MSC): a comparison of adult and neonatal tissue-derived MSC. Cell Commun Signal. 2011 May 14;9:12. PubMed PMID: 21569606; PubMed Central PMCID: PMCPMC3117820.
- Caplan AI. Mesenchymal stem cells: time to change the name! Stem Cells Transl Med. 2017 Jun;6(6):1445–1451. PubMed PMID: 28452204; PubMed Central PMCID: PMCPMC5689741.
- Caplan AI, Correa D. The MSC: an injury drugstore. Cell Stem Cell. 2011 Jul 8;9(1):11–15. PubMed PMID: 21726829; PubMed Central PMCID: PMCPMC3144500.
- Rani S, Ryan AE, Griffin MD, et al. Mesenchymal stem cell-derived extracellular vesicles: toward cell-free therapeutic applications. Mol Therapy: Journal Am Soc Gene Ther. 2015 May;23(5):812–823. PubMed PMID: 25868399; PubMed Central PMCID: PMCPMC4427881. eng.
- Gnecchi M, Danieli P, Malpasso G, et al. Paracrine mechanisms of mesenchymal stem cells in tissue repair. Methods Molecular Biol (Clifton, NJ). 2016;1416:123–146. PubMed PMID: 27236669; eng.
- Liang X, Ding Y, Zhang Y, et al. Paracrine mechanisms of mesenchymal stem cell-based therapy: current status and perspectives. Cell Transplant. 2014;23(9):1045–1059. PubMed PMID: 23676629; eng.
- Wang A, Brown EG, Lankford L, et al. Placental mesenchymal stromal cells rescue ambulation in ovine myelomeningocele. Stem Cells Transl Med. 2015 Jun;4(6):659–669. PubMed PMID: 25911465; PubMed Central PMCID: PMCPMC4449103. eng.
- Gonzalez-King H, Garcia NA, Ontoria-Oviedo I, et al. Hypoxia inducible factor-1alpha potentiates jagged 1-mediated angiogenesis by mesenchymal stem cell-derived exosomes. Stem Cells (Dayton, Ohio). 2017 Jul;35(7):1747–1759. PubMed PMID: 28376567; eng.
- Salomon C, Ryan J, Sobrevia L, et al. Exosomal signaling during hypoxia mediates microvascular endothelial cell migration and vasculogenesis. PLoS One. 2013;8(7):e68451. PubMed PMID: 23861904; PubMed Central PMCID: PMCPMC3704530.
- Xue C, Shen Y, Li X, et al. Exosomes derived from hypoxia-treated human adipose mesenchymal stem cells enhance angiogenesis through the PKA signaling pathway. Stem Cells Dev. 2018 Apr 1;27(7):456–465. PubMed PMID: 29415626.
- Cardenas-Aguayo Mdel C, Kazim SF, Grundke-Iqbal I, et al. Neurogenic and neurotrophic effects of BDNF peptides in mouse hippocampal primary neuronal cell cultures. PloS one. 2013;8(1):e53596. PubMed PMID: 23320097; PubMed Central PMCID: PMCPMC3539976. eng.
- Liu C, Chan CB, Ye K. 7,8-dihydroxyflavone, a small molecular TrkB agonist, is useful for treating various BDNF-implicated human disorders. Transl Neurodegener. 2016 Jan 06;5:2. 09/30/received12/29/accepted. PubMed PMID: PMC4702337.
- Massa SM, Yang T, Xie Y, et al. Small molecule BDNF mimetics activate TrkB signaling and prevent neuronal degeneration in rodents. J Clin Invest. 2010 May;120(5):1774–1785. PubMed PMID: 20407211; PubMed Central PMCID: PMCPMC2860903. eng.
- Jia Y, Shi X, Xie Y, et al. Human umbilical cord stem cell conditioned medium versus serum-free culture medium in the treatment of cryopreserved human ovarian tissues in in-vitro culture: a randomized controlled trial. Stem Cell Res Ther. 2017 Jun 24;8(1):152. PubMed PMID: 28646900; PubMed Central PMCID: PMCPMC5482969. eng.
- Lee CY, Kang JY, Lim S, et al. Hypoxic conditioned medium from mesenchymal stem cells promotes lymphangiogenesis by regulation of mitochondrial-related proteins. Stem Cell Res Ther. 2016 Mar 11;7:38. 08/17/received02/15/revised02/22/accepted. PubMed PMID: PMC4788827. .
- Ho JCY, Lai W-H, Li M-F, et al. Reversal of endothelial progenitor cell dysfunction in patients with type 2 diabetes using a conditioned medium of human embryonic stem cell-derived endothelial cells. Diabetes Metab Res Rev. 2012;28(5):462–473.
- Gong M, Yu B, Wang JC, et al. Mesenchymal stem cells release exosomes that transfer miRNAs to endothelial cells and promote angiogenesis. Oncotarget. 2017 Jul 11;8(28):45200–45212. PubMed PMID: WOS:000405504600020; English.
- Mathew SA, Chandravanshi B, Bhonde R. Hypoxia primed placental mesenchymal stem cells for wound healing. Life Sci. 2017 Aug 01;182:85–92.
- Kholia S, Ranghino A, Garnieri P, et al. Extracellular vesicles as new players in angiogenesis. Vascul Pharmacol. 2016 Nov;86:64–70. PubMed PMID: 27013016.
- Burrello J, Monticone S, Gai C, et al. Stem cell-derived extracellular vesicles and immune-modulation. Front Cell Dev Biol. 2016;4:83. PubMed PMID: 27597941; PubMed Central PMCID: PMCPMC4992732.
- Eitan E, Suire C, Zhang S, et al. Impact of lysosome status on extracellular vesicle content and release. Ageing Res Rev. 2016 Dec;32:65–74. PubMed PMID: WOS:000390623400007; English.
- Escudero CA, Lazo OM, Galleguillos C, et al. The p75 neurotrophin receptor evades the endolysosomal route in neuronal cells, favouring multivesicular bodies specialised for exosomal release. J Cell Sci. 2014 May 01;127(Pt 9):1966–1979. PubMed PMID: 24569882; PubMed Central PMCID: PMCPMC4004974. eng.
- Marote A, Teixeira FG, Mendes-Pinheiro B, et al. MSCs-derived exosomes: cell-secreted nanovesicles with regenerative potential. Front Pharmacol. 2016;7:231. PubMed PMID: 27536241; PubMed Central PMCID: PMCPMC4971062.
- Nawaz M, Camussi G, Valadi H, et al. The emerging role of extracellular vesicles as biomarkers for urogenital cancers. Nat Rev Urol. 2014 Dec;11(12):688–701. PubMed PMID: WOS:000345908700006; English.
- Urbanelli L, Magini A, Buratta S, et al. Signaling pathways in exosomes biogenesis, secretion and fate. Genes (Basel). 2013 Mar 28;4(2):152–170. PubMed PMID: 24705158; PubMed Central PMCID: PMCPMC3899971.
- Coumans FAW, Brisson AR, Buzas EI, et al. Methodological guidelines to study extracellular vesicles. Circ Res. 2017 May 12;120(10):1632–1648. PubMed PMID: 28495994.
- Mateescu B, Kowal EJ, van Balkom BW, et al. Obstacles and opportunities in the functional analysis of extracellular vesicle RNA - an ISEV position paper. J Extracell Vesicles. 2017;6(1):1286095. PubMed PMID: 28326170; PubMed Central PMCID: PMCPMC5345583.
- Ramirez MI, Amorim MG, Gadelha C, et al. Technical challenges of working with extracellular vesicles. Nanoscale. 2018 Jan 18;10(3):881–906. PubMed PMID: 29265147.
- Gyorgy B, Szabo TG, Pasztoi M, et al. Membrane vesicles, current state-of-the-art: emerging role of extracellular vesicles. Cell Molecular Life Sci: CMLS. 2011 Aug;68(16):2667–2688. PubMed PMID: 21560073; PubMed Central PMCID: PMCPMC3142546. eng.
- Hao D, Xiao W, Liu R, et al. Discovery and characterization of a potent and specific peptide ligand targeting endothelial progenitor cells and endothelial cells for tissue regeneration. ACS Chem Biol. 2017 Apr 21;12(4):1075–1086. PubMed PMID: 28195700; eng.
- Tao SC, Guo SC, Li M, et al. Chitosan wound dressings incorporating exosomes derived from microRNA-126-overexpressing synovium mesenchymal stem cells provide sustained release of exosomes and heal full-thickness skin defects in a diabetic rat model. Stem Cells Transl Med. 2017 Mar;6(3):736–747. PubMed PMID: 28297576; PubMed Central PMCID: PMCPMC5442792. eng.
- Bai L, Shao H, Wang H, et al. Effects of mesenchymal stem cell-derived exosomes on experimental autoimmune uveitis. Sci Rep. 2017 Jun 28;7(1):4323. PubMed PMID: 28659587; PubMed Central PMCID: PMCPMC5489510. eng.
- Lasser C, Jang SC, Lotvall J. Subpopulations of extracellular vesicles and their therapeutic potential. Mol Aspects Med. 2018 Apr;60:1–14. PubMed PMID: 29432782; eng.
- Lai RC, Tan SS, Yeo RW, et al. MSC secretes at least 3 EV types each with a unique permutation of membrane lipid, protein and RNA. J Extracell Vesicles. 2016;5:29828. PubMed PMID: 26928672; PubMed Central PMCID: PMCPMC4770866.
- Pegtel DM, Peferoen L, Amor S. Extracellular vesicles as modulators of cell-to-cell communication in the healthy and diseased brain. PubMed PMID: PMC4142037 Philosophical Trans Royal Soc B: Biol Sci. 2014;3691652:20130516.
- Yáñez-Mó M, Siljander PRM, Andreu Z, et al. Biological properties of extracellular vesicles and their physiological functions. J Extracellular Vesicles. 2015 May 14;4. 12/22/received02/24/revised03/10/accepted doi:10.3402/jev.v4.27066. PubMed PMID: PMC4433489
- Xin H, Li Y, Chopp M. Exosomes/miRNAs as mediating cell-based therapy of stroke [Review]. Front Cell Neurosci. 2014 2014 Nov 10; 8(377): doi:10.3389/fncel.2014.00377. English
- Pachler K, Lener T, Streif D, et al. A good manufacturing practice-grade standard protocol for exclusively human mesenchymal stromal cell-derived extracellular vesicles. Cytotherapy. 2017 Apr;19(4):458–472. PubMed PMID: 28188071.
- Luan X, Sansanaphongpricha K, Myers I, et al. Engineering exosomes as refined biological nanoplatforms for drug delivery [Review]. Acta Pharmacologica Sinica. 2017;38:754. 04/10/online.
- Willis GR, Kourembanas S, Mitsialis SA. Toward exosome-based therapeutics: isolation, heterogeneity, and fit-for-purpose potency. Front Cardiovasc Med. 2017 Oct 09;4:63. 07/10/received09/25/accepted. PubMed PMID: PMC5640880.
- Ferguson SW, Wang J, Lee CJ, et al. The microRNA regulatory landscape of MSC-derived exosomes: a systems view. Sci Rep. 2018;8(1):1419.
- Bagno L, Hatzistergos KE, Balkan W, et al. Mesenchymal stem cell-based therapy for cardiovascular disease: progress and challenges. Mol Therapy: Journal Am Soc Gene Ther. 2018 Jul 5;26(7):1610–1623. PubMed PMID: 29807782; PubMed Central PMCID: PMCPMC6037203. eng.
- Cha JM, Shin EK, Sung JH, et al. Efficient scalable production of therapeutic microvesicles derived from human mesenchymal stem cells. Sci Rep. 2018 Jan 19;8(1):1171. PubMed PMID: 29352188; PubMed Central PMCID: PMCPMC5775399. eng.
- Kim HY, Kumar H, Jo M-J, et al. Therapeutic efficacy-potentiated and diseased organ-targeting nanovesicles derived from mesenchymal stem cells for spinal cord injury treatment. Nano Letters. 2018 2018 Jul 11. DOI:10.1021/acs.nanolett.8b01816.
- Cheng L, Zhang K, Wu S, et al. Focus on mesenchymal stem cell-derived exosomes: opportunities and challenges in cell-free therapy. Stem Cells Int. 2017 Dec 19;2017:6305295. 08/11/received11/05/revised11/22/accepted. PubMed PMID: PMC5749272.
- Du W, Zhang K, Zhang S, et al. Enhanced proangiogenic potential of mesenchymal stem cell-derived exosomes stimulated by a nitric oxide releasing polymer. Biomaterials. 2017 Jul;133:70–81. PubMed PMID: 28433939; eng.
- Huleihel L, Hussey GS, Naranjo JD, et al. Matrix-bound nanovesicles within ECM bioscaffolds. Sci Adv. 2016 Jun;2(6):e1600502. PubMed PMID: 27386584; PubMed Central PMCID: PMCPMC4928894.
- Qazi TH, Mooney DJ, Duda GN, et al. Biomaterials that promote cell-cell interactions enhance the paracrine function of MSCs. Biomaterials. 2017 Sep;140:103–114. PubMed PMID: 28644976; eng.
- Su N, Gao PL, Wang K, et al. Fibrous scaffolds potentiate the paracrine function of mesenchymal stem cells: a new dimension in cell-material interaction. Biomaterials. 2017 Oct;141:74–85. PubMed PMID: 28667901; eng.
- Hanley PJ, Mei ZY, Durett AG, et al. Efficient manufacturing of therapeutic mesenchymal stromal cells with the use of the quantum cell expansion system. Cytotherapy. 2014 Aug;16(8):1048–1058. PubMed PMID: WOS:000338819200003; English.
- Panchalingam KM, Jung S, Rosenberg L, et al. Bioprocessing strategies for the large-scale production of human mesenchymal stem cells: a review. Stem Cell Res Ther. 2015 Nov 23;6:225. doi:10.1186/s13287-015-0228-5. PubMed PMID: 26597928; PubMed Central PMCID: PMCPMC4657237.
- Petry F, Smith JR, Leber J, et al. Manufacturing of human umbilical cord mesenchymal stromal cells on microcarriers in a dynamic system for clinical use. Stem Cells Int. 2016. doi:10.1155/2016/4834616. PubMed PMID: WOS:000373501800001; English
- Rafiq QA, Coopman K, Nienow AW, et al. Systematic microcarrier screening and agitated culture conditions improves human mesenchymal stem cell yield in bioreactors. Biotechnol J. 2016 Mar;11(4):473–486. PubMed PMID: 26632496; PubMed Central PMCID: PMCPMC4991290. eng.
- Teixeira FG, Panchalingam KM, Assuncao-Silva R, et al. Modulation of the mesenchymal stem cell secretome using computer-controlled bioreactors: impact on neuronal cell proliferation, survival and differentiation. Sci Rep-Uk. 2016 Jun 15;6:27791. PubMed PMID: WOS:000384559600001; English.
- Lechanteur C, Briquet A, Giet O, et al. Clinical-scale expansion of mesenchymal stromal cells: a large banking experience. J Transl Med. 2016 May 20;14(1):145. PubMed PMID: 27207011; PubMed Central PMCID: PMCPMC4875672.
- Yan IK, Shukla N, Borrelli DA, et al. Use of a hollow fiber bioreactor to collect extracellular vesicles from cells in culture. Methods Molecular Biol (Clifton, NJ). 2018;1740:35–41. PubMed PMID: 29388134; eng.
- Qu Y, Dubyak GR. P2X7 receptors regulate multiple types of membrane trafficking responses and non-classical secretion pathways. Purinergic Signal. 2009 Feb 03 05/26/received;5(2):163–173. . PubMed PMID: PMC2686822.
- Colombo M, Raposo G, Thery C. Biogenesis, secretion, and intercellular interactions of exosomes and other extracellular vesicles. Annu Rev Cell Dev Biol. 2014;30:255–289. PubMed PMID: 25288114.
- Fader CM, Sanchez DG, Mestre MB, et al. TI-VAMP/VAMP7 and VAMP3/cellubrevin: two v-SNARE proteins involved in specific steps of the autophagy/multivesicular body pathways. Biochim Biophys Acta. 2009 Dec;1793(12):1901–1916. PubMed PMID: 19781582.
- Rao SK, Huynh C, Proux-Gillardeaux V, et al. Identification of SNAREs involved in synaptotagmin VII-regulated lysosomal exocytosis. J Biol Chem. 2004 May 7;279(19):20471–20479. PubMed PMID: 14993220.
- Lespagnol A, Duflaut D, Beekman C, et al. Exosome secretion, including the DNA damage-induced p53-dependent secretory pathway, is severely compromised in TSAP6/Steap3-null mice. Cell Death Differ. 2008 Nov;15(11):1723–1733. PubMed PMID: 18617898; eng.
- Yu X, Harris SL, Levine AJ. The regulation of exosome secretion: a novel function of the p53 protein. Cancer Res. 2006 May 01;66(9):4795–4801. . PubMed PMID: 16651434; eng.
- Wan C, Fu J, Wang Y, et al. Exosome-related multi-pass transmembrane protein TSAP6 is a target of rhomboid protease RHBDD1-induced proteolysis. PloS one. 2012;7(5):e37452. PubMed PMID: 22624035; PubMed Central PMCID: PMCPMC3356283. eng.
- Laulagnier K, Grand D, Dujardin A, et al. PLD2 is enriched on exosomes and its activity is correlated to the release of exosomes. FEBS letters. 2004 Aug 13;572(1–3):11–14. PubMed PMID: 15304316; eng.
- Hsu C, Morohashi Y, Yoshimura S, et al. Regulation of exosome secretion by Rab35 and its GTPase-activating proteins TBC1D10A-C. J Cell Biol. 2010 Apr 19;189(2):223–232. PubMed PMID: 20404108; PubMed Central PMCID: PMCPMC2856897. eng.
- Ostrowski M, Carmo NB, Krumeich S, et al. Rab27a and Rab27b control different steps of the exosome secretion pathway. Nat Cell Biol. 2010 Jan;12(1):19–30. PubMed PMID: 19966785.
- Fatima F, Ekstrom K, Nazarenko I, et al. Non-coding RNAs in mesenchymal stem cell-derived extracellular vesicles: deciphering regulatory roles in stem cell potency, inflammatory resolve, and tissue regeneration. Front Genet. 2017;8:161. PubMed PMID: 29123544; PubMed Central PMCID: PMCPMC5662888.
- Ma J, Zhao Y, Sun L, et al. Exosomes derived from AKT-modified human umbilical cord mesenchymal stem cells improve cardiac regeneration and promote angiogenesis via activating platelet-derived growth factor D. Stem Cells Transl Med. 2017 Jan;6(1):51–59. PubMed PMID: 28170176; PubMed Central PMCID: PMCPMC5442756. eng.
- Malik SZ, Motamedi S, Royo NC, et al. Identification of potentially neuroprotective genes upregulated by neurotrophin treatment of CA3 neurons in the injured brain. J Neurotrauma. 2011 Mar;28(3):415–430. PubMed PMID: 21083427; PubMed Central PMCID: PMCPMC3057196. eng.
- Ti DD, Hao HJ, Fu XB, et al. Mesenchymal stem cells-derived exosomal microRNAs contribute to wound inflammation. Sci China Life Sci. 2016 Dec;59(12):1305–1312. PubMed PMID: WOS:000390669100012; English.
- Xin H, Li Y, Liu Z, et al. MiR-133b promotes neural plasticity and functional recovery after treatment of stroke with multipotent mesenchymal stromal cells in rats via transfer of exosome-enriched extracellular particles. Stem Cells (Dayton, Ohio). 2013 Dec;31(12):2737–2746. PubMed PMID: 23630198; PubMed Central PMCID: PMCPMC3788061. eng.
- Mayourian J, Ceholski DK, Gorski PA, et al. Exosomal microRNA-21-5p mediates mesenchymal stem cell paracrine effects on human cardiac tissue contractility. Circ Res. 2018 Mar 30;122(7):933–944. PubMed PMID: 29449318.
- Mathiyalagan P, Sahoo S. Exosomes-based gene therapy for microRNA delivery. Methods Mol Biol. 2017;1521:139–152. PubMed PMID: 27910046; PubMed Central PMCID: PMCPMC5502074.
- Lener T, Gimona M, Aigner L, et al. Applying extracellular vesicles based therapeutics in clinical trials - an ISEV position paper. J Extracell Vesicles. 2015;4:30087. PubMed PMID: 26725829; PubMed Central PMCID: PMCPMC4698466.