ABSTRACT
Post-staining of extracellular vesicles (EVs) with lipid-anchored fluorophores (LAFs) such as PKH67 is a widely used strategy for studying EVs but it is associated with several pitfalls. The pitfalls discussed in this commentary are related to LAF labelling of non-EV species due to (1) lipoprotein contamination in EV samples, (2) desorption of the LAF reporters from vesicles into proteins and lipoproteins in blood and serum, and (3) the capability of the amphiphilic LAF compounds to form EV-like particles. Awareness of these challenges and developing solutions to overcome these are important to ensure that we make relevant interpretations when using LAFs to track EVs.
Extracellular vesicles (EVs) have gained considerable attention over the last decade due to their targeting potential and long-range cell-to-cell communication properties [Citation1,Citation2], as well as their proposed clinical potential as disease biomarkers [Citation3,Citation4] and drug delivery systems [Citation5–Citation7]. These spherical biological nanoparticles (~30–1000 nm) are comprised of a lipid membrane containing membrane-bound proteins, and an aqueous core capable of carrying biological cargo, such as nucleic acids, proteins and other biomolecules, and are secreted from most types of cells into most types of bodily fluids [Citation8–Citation10]. A wide range of in vitro and in vivo techniques are used to gain insight into the biological functions of EVs, including flow cytometry, confocal microscopy and in vivo fluorescence-detection techniques. While these techniques can reveal information about the internalization of EVs by cells or tissues, they also rely on fluorescent reporters associated to the EVs. One widely used method to achieve this is by post-inserting lipid-anchored fluorophores (LAFs) into the EV membrane.
When LAFs are post-inserted into EVs, the outcome is typically LAF-labelled EVs and free LAFs in solution. To remove free LAFs (that could pose a challenge to EV-based uptake studies) from the LAF-labelled EVs various types of physical-based methods are used, including spin filtration, size-exclusion chromatography, ultracentrifugation and density gradient centrifugation. However, more complicated challenges beyond the presence of free LAFs after staining the EVs exist. A challenge that is far less known and appreciated in the field, is the concomitant labelling of non-EV particles in addition to EVs [Citation11,Citation12]. Importantly, the danger of labelling non-EV particles remains even when purified stained vesicles samples are used [Citation13]. These not-so-known pitfalls related to unintended LAF labelling of non-EV species including LAF dissociation from vesicles were recently reported [Citation13]. Thus, I find it timely and very important to highlight and bring together these essential staining issues in an integrated manner and provide some tentative solutions to overcome these challenges. I would also like to stress that the presence of non-EV LAF-labelled particles in EV-based uptake and biodistribution studies that rely on the LAF-fluorescence will likely distort the outcome of the experiments and lead to wrong conclusions about the targeting properties and biological fate of EVs.
Several studies have highlighted the high degree of lipoprotein contamination in ostensibly purified EV samples obtained from plasma [Citation14,Citation15]. The similarity in physical properties between EVs and different types of lipoproteins [Citation16], and the ~6 orders of magnitude higher concentration of lipoproteins relative to EVs in human plasma [Citation17] commonly lead to high lipoprotein contamination in EV isolates from plasma when using physical-based isolation methods. Likewise, EV isolates from cell culture medium are also prone to lipoprotein contamination when serum (incl. EV-depleted serum) has been present during the EV production. Thus, the typical EV isolate likely contains lipoproteins that are comprised of a lipid monolayer at their surface and can, therefore, compete with the EVs for incorporating the hydrophobic LAF labels. This fact is indeed a challenge because LAF labelling is also used to investigate the cellular uptake of various lipoprotein particles, including very low-density lipoproteins (VLDL) [Citation18], low-density lipoproteins (LDL) [Citation19] and high-density lipoproteins (HDL) [Citation20]. The unintended LAF labelling of the different types of bio-nanoparticles including lipoproteins in an apparently pure EV sample [Citation11] is shown in path (a) of .
Figure 1. Schematic illustration of the proposed paths of the lipid-anchored fluorophore (LAF) used for labelling and tracking EVs that are discussed in this commentary.
(a) LAF labelling of non-EV serum components, that are often present in EV isolates, in addition to EV labelling. (b) LAF labelling of pure EVs. (c) Dissociation of LAFs from EVs into serum components. (d) Formation of LAF particles that exhibit low fluorescence due to self-quenching.
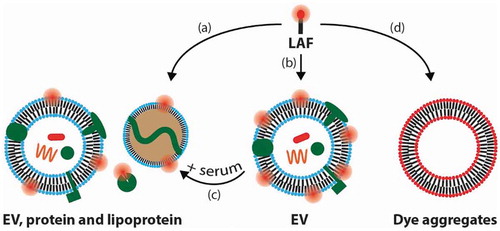
In an ideal case, it would be possible to obtain 100% pure samples of EVs labelled with the LAF of choice (path (b) in ). However, in this case, one would still face challenges when studying the functional characteristics of the EVs, e.g. in an internalization experiment. The inherent dynamics of lipids [Citation21,Citation22] can lead to LAF spill over from EVs into lipoproteins and plasma/serum proteins including albumin (albumin contains multiple hydrophobic binding pockets [Citation23]) when the purified LAF-labelled EVs are exposed to a biological environment, such as serum-containing growth medium or blood (path (c) in ). We recently showed that highly stable artificial vesicles (liposomes) labelled with commonly used LAFs desorb a significant amount of their LAF content into other plasma components and that the propensity of the LAF to desorb from liposomes correlated with the degree of cell staining in a liposome uptake study [Citation13]. Such LAF desorption is also expected from EVs due to their similarity with liposomes. Several physicochemical parameters dictate to which degree the LAF desorption will take place including (1) the ratio between the total EV membrane area and the total “competing” membrane area from the serum proteins, lipoproteins and cells, (2) the activation barrier of the LAF to leave the EV and (3) the partitioning of the LAF to EVs versus non-EV species.
When the LAF-labelled EV sample also contains LAF-labelled lipoproteins either due to lipoprotein contamination of the isolated EV sample or due to the desorption of LAFs from EVs into the lipoproteins after labelling, the interpretation of EV uptake experiments relying on the fluorescence from the dynamic LAF reporters becomes practically impossible. Are we looking at uptake of EVs, lipoproteins or both species? Along these lines, it should be noted that the receptors which facilitate cholesterol influx from lipoproteins into cells are overexpressed by most tumour cells [Citation20]. Thus in a cancer setting, an area that the majority of the EV field is focused on, lipoprotein-based LAF accumulation interpreted as EVs is a major issue. Further, many of the lipoprotein receptors are naturally expressed in cells such as endothelial cells and monocytes/macrophages – which are widely studied for their ability to take up or scavenge EVs.
The amphiphilic nature of LAFs, like bare phospholipids, drives the capability of the LAFs themselves to form nanoparticles in solution (path (d) in ). A new study showed that PKH26, a commonly used LAF to label EVs form EV-sized PKH26 nanoparticles [Citation12]. In addition, we observed that di-8-ANEPPS (LAF), which has been used to stain EVs, forms vesicular-like particles with similar sizes (100 nm) to EVs, when mixed with phosphate-buffered saline (manuscript in preparation). LAF particles can potentially act as fluorescent ticking “bombs” in an apparently purified EV sample because they are likely to be self-quenched – the close proximity between the LAF compounds in a LAF-particle can damp the fluorescence brightness of each of the individual LAF compounds. Conversely, when these LAF particles are exposed to blood, serum and cells, the LAF compounds may desorb from the LAF particles (by a similar mechanism to the one shown in (path c)) and stain serum components and cells in a non-specific fashion. This will lead to an increase in the fluorescence signal from the LAFs due to dequenching and thereby complicate the tracking of the actual LAF-labelled EVs. Several EV studies have applied cell staining protocols to stain EVs. In many of these cases, a huge excess of LAF compared to EV lipids are used because the total amount of EV lipids (or total EV surface) is much lower when compared to cells. The huge excess of LAF can both lead to the formation of LAF particle artefacts but also alter the overall structure and function of the EVs if the EVs end up hosting a significant amount of LAF in their membrane. On this note, the LAF particles will not be removed by SEC or spin filtration, when these traditional methods are applied to remove non-EV-bound LAF compounds.
I suggest the following solutions to partly overcome these not-so-known challenges shown in . Path (a): rather than using a single purification step based on a physical method that separates species according to size (size-exclusion chromatography), density (density gradient centrifugation) or both (centrifugation) that has proven to be inefficient [Citation14,Citation15], I have previously suggested employing a two-step procedure based on size and density separation to reduce the lipoprotein contamination in EV isolates [Citation16]. Recent studies have with some success applied this two-step purification approach [Citation24]. Another potential strategy would be to use chemical/biological-based methods, such as immuno-precipitation. However, the lack of a universal EV biomarker currently renders this approach challenging too. Paths (a) and (d): it might be beneficial (time- and yield-wise) to stain the EV sample prior to the two-step EV-purification so that biological as well as free and aggregated LAF contaminants will be simultaneously removed. In cases when only a single-step purification scenario is required or possible, I would recommend an improved (high-resolution) SEC step compared to standard SEC, which should be efficient in removing the most abundant lipoproteins including HDL and LDL from the larger sized EVs. However, in this case the pure LAF particles (if present) could co-elute with EVs. Path (c): regarding the desorption of LAF from LAF-labelled EVs into plasma components, I recommend using highly lipophilic LAF labels, i.e. lipid-anchors containing two long alkyl/acyl chains since they interact more strongly with the EV membrane than their less lipophilic counterparts. The downside of using highly lipophilic compounds is their strong tendency to form aggregates (LAF particles) and hence their presence needs to be evaluated. The process of post-inserting highly lipophilic compounds into EVs in aqueous environment is a challenge in itself that is not discussed in this commentary. The less lipophilic LAFs (e.g. short chained acyl, alkyl or cholesterol anchored fluorophore compounds) may be easier to post-insert into EVs and to subsequently remove the free monomeric or micellar LAFs. However, they complicate things in a medium with a large reservoir of lipid surfaces beyond the EVs including lipoproteins and cells that can compete for the low partitioning LAFs. Thus, in the case of the less lipophilic LAFs one will be confronted with the easy-in/easy-out dynamic. To evaluate to what extent within the relevant time frame a specific LAF is dissociating from the LAF-labelled EVs in a biological environment, I propose to use the high-resolution SEC analysis that our laboratory recently reported [Citation13].
To avoid the issues related to the dynamics of LAFs, chemical staining of the less dynamic transmembrane-bound EV-proteins could improve the specific staining of EVs. However, this strategy could in some cases impair the functionality of the EVs including their targeting properties that are typically thought to be mediated by the membrane-bound proteins. A completely different solution to overcoming the LAF-related problems that is already used is the transfection of cells to produce EVs containing a genetically encoded fluorescent reporter, such as CD63-eGFP. Although this approach can be specific, it may only “label” a subpopulation of the EVs and the transfection/labelling efficiency is low [Citation25]. Additionally, this method is relatively laborious and is readily applicable mainly to EVs produced from cultured cells. Another approach to inducing the parental cells to release fluorescently labelled EVs is using a cytoplasmic dye, such as 5,6-carboxy-succinimidyl-fluoresceine ester (CFSE). However, this approach can lead to detrimental effects on the function of immune cells [Citation26] likely due to the impairment of protein functions caused by the protein-cytoplasmic dye conjugation.
As discussed above, the concerns related to the fluorophore-reporters used to track EVs are many. Additionally, the fluorescence-based techniques used to track these EVs/fluorophore-reporters including confocal and flow cytometry are limited by their inability to quantify what proportion of the total EV population is internalized in cells. If the actual percentage of EVs that are taken up by cells is low, the concerns about non-EV LAF-labelled particle uptake in these studies seem to be even more relevant.
This commentary highlights not-so-known pitfalls related to the commonly used fluorophore-labelling of EVs and provides tentative solutions to reduce the presence of non-EV LAF-labelled particles for EV tracking studies. We need to fully address and solve these challenges to properly use LAF-labelled EVs to evaluate their targeting and biological fate, so that we can reveal the true biological functions of EVs and exploit their proposed clinical applications as a useful diagnostic tool and drug delivery system. Additionally, this work illustrates that the EV field would clearly benefit from a stronger collaboration between biologists and chemists. Multifaceted expertise is needed to address the intricate demands posed by EVs to ensure the integrity of experimental designs and thus, the validity of any conclusions about EVs.
Acknowledgments
The author thanks Kasper B. Johnsen, Paul Kempen, Johann M. Gudbergsson, Katrine Jønsson and Ewa and Andzrej Witkowski for critical reading of the manuscript.
Disclosure statement
No potential conflict of interest was reported by the author.
Additional information
Funding
References
- Peinado H, Alečković M, Lavotshkin S, et al. Melanoma exosomes educate bone marrow progenitor cells toward a pro-metastatic phenotype through MET. Nat Med. 2012;18:883–891.
- Hoshino A, Costa-Silva B, Shen T-L, et al. Tumour exosome integrins determine organotropic metastasis. Nature. 2015;527:329–335.
- Properzi F, Logozzi M, Fais S. Exosomes: the future of biomarkers in medicine. Biomark Med. 2013;7:769–778.
- Shah R, Patel T, Freedman JE. Circulating extracellular vesicles in human disease. N Engl J Med. 2018;379:958–966.
- Johnsen, KB, Gudbergsson JM, Skov MN, et al. A comprehensive overview of exosomes as drug delivery vehicles - endogenous nanocarriers for targeted cancer therapy. Biochim Biophys Acta Rev Cancer. 2014;1846:75–4.
- Johnsen, KB, Gudbergsson JM, Duroux M, et al. On the use of liposome controls in studies investigating the clinical potential of extracellular vesicle-based drug delivery systems - a commentary. J Control Release. 2018;269:10–14.
- Lener, T, Gimona M, Aigner L, et al. Applying extracellular vesicles based therapeutics in clinical trials - an ISEV position paper. J Extracell Vesicles. 2015;4:30087.
- Colombo M, Raposo G, Théry C. Biogenesis, secretion, and intercellular interactions of exosomes and other extracellular vesicles. Annu Rev Cell Dev Biol. 2014;30:255–289.
- Van Niel G, D’Angelo G, Raposo G. Shedding light on the cell biology of extracellular vesicles. Nat Rev Mol Cell Biol. 2018;19:213–228.
- Yáñez-Mó, M, Siljander PR, Andreu Z, et al. Biological properties of extracellular vesicles and their physiological functions. J Extracell Vesicles. 2015. DOI:10.3402/jev.v4.27066.
- Takov K, Yellon DM, Davidson SM. Confounding factors in vesicle uptake studies using fluorescent lipophilic membrane dyes. J Extracell Vesicles. 2017;6:1388731.
- Puzar Dominkus P, Stenovec M, Sitar S, et al. PKH26 labeling of extracellular vesicles: characterization and cellular internalization of contaminating PKH26 nanoparticles. Biochim Biophys Acta. 2018;1860:1350–1361.
- Münter R, Kristensen K, Pedersbæk D, et al. Dissociation of fluorescently labeled lipids from liposomes in biological environments challenges the interpretation of uptake studies. Nanoscale. 2018;10:22720–22724.
- Yuana Y, Levels J, Grootemaat A, et al. Co-isolation of extracellular vesicles and high-density lipoproteins using density gradient ultracentrifugation. J Extracell Vesicles. 2014;3:doi: 10.3402/jev.v3.23262. eCollection 2014.
- Sodar BW, Kittel Á, Pálóczi K, et al. Low-density lipoprotein mimics blood plasma-derived exosomes and microvesicles during isolation and detection. Sci Rep. 2016;6:24316.
- Simonsen JB. What are we looking at? Extracellular vesicles, lipoproteins, or both? Circ Res. 2017;121:920–922.
- Johnsen KB, Gudbergsson JM, Andresen TL, et al. What is the blood concentration of extracellular vesicles? Implications for the use of extracellular vesicles as blood-borne biomarkers of cancer. Biochim Biophys Acta Rev Cancer. 2019;1871:109-116.
- Hussain, M. M., F. R. Maxfield, J. Mas-Oliva et al. Clearance of chylomicron remnants by the low-density-lipoprotein receptor-related protein-alpha-2-macroglobulin receptor. J Biol Chem. 1991;266:13936–13940.
- Sawamura T, Kume N, Aoyama T, et al. An endothelial receptor for oxidized low-density lipoprotein. Nature. 1997;386:73–77.
- Acton S, Rigotti A, Landschulz KT, et al. Identification of scavenger receptor SR-BI as a high density lipoprotein receptor. Science. 1996;271:518–520.
- McLean LR, Phillips MC. Kinetics of phosphatidylchohne and lysophosphatidylcholine exchange between unilamellar vesicles. Biochemistry. 1984;23:4624–4630.
- Nakano M, Fukuda M, Kudo T, et al. Determination of interbilayer and transbilayer lipid transfers by time-resolved small-angle neutron scattering. Phys Rev Lett. 2007;98. DOI:10.1103/PhysRevLett.98.238101.
- Larsen MT, Kuhlmann M, Hvam ML, et al. Albumin-based drug delivery: harnessing nature to cure disease. Mol Cell Ther. 2016. DOI:10.1186/s40591-016-0048-8
- Karimi N, Cvjetkovic A, Jang SC, et al. Detailed analysis of the plasma extracellular vesicle proteome after separation from lipoproteins. Cell Mol Life Sci. 2018;75:2873–2886.
- Sutaria DS, Badawi M, Phelps MA, et al. Achieving the promise of therapeutic extracellular vesicles: the devil is in details of therapeutic loading. Pharm Res. 2017;34:1053–1066.
- van der Vlist EJ, Nolte-’T Hoen ENM, Stoorvogel W, et al. Fluorescent labeling of nano-sized vesicles released by cells and subsequent quantitative and qualitative analysis by high-resolution flow cytometry. Nat Protoc. 2012;7:1311–1326.