ABSTRACT
Objectives
Since the initial release of biosimilars 18 years ago, regulations for their licensing have changed considerably; however, there is no global consensus on these regulations. Establishing harmonized regulatory guidelines for the approval of biosimilars with support from the ICH, an independent, non-profit association under Swiss law, will significantly enhance the affordability of biological drugs.
Methods
Regulatory guidelines from the Food and Drug Administration (FDA), European Medicines Agency (EMA), Medicines and Healthcare products Regulatory Agency (MHRA), and World Health Organization (WHO) were analyzed for historical changes and elements critical to the safety and efficacy of biosimilars.
Results
Analysis of all EMA and FDA filings show that none of the animal testing and clinical efficacy testing failed because animals do not have the required receptors to initiate pharmacologic responses, and efficacy studies cannot be statistically powered to conclude any results. New analytical technologies will enable good biosimilarity determination, avoiding both tests.
Conclusion
Scientifically based ICH guidelines that remove redundant studies will reduce development costs, improve safety, and allow global drug distribution based on single compliance. These guidelines are particularly necessary for emerging countries lacking the expertise and resources to evaluate biosimilar filings.
Background
The International Council for Harmonization (ICH), formerly the International Conference on Harmonization (also known as ICH), held inaugural assembly meetings on 23 October 2015, to establish the ICH as an international association and legal entity under Swiss law [Citation1]. This foundation has a 25-year track record of successfully delivering harmonized guidelines for global pharmaceutical development and regulation, and it has long recognized the need to harmonize various guidelines. In the 1960s and 70s, the laws, rules, and standards for reporting and analyzing data on the safety, quality, and efficacy of new pharmaceutical goods in most countries were greatly expanded, regardless of whether the countries had previously implemented product registration procedures. In addition, the industry has been expanding internationally, and new global markets are being developed. However, because of differences in the technical standards between nations, many time-consuming and costly test procedures must be duplicated to market new products internationally. Regulations must be rationalized and harmonized because of concerns related to the rising cost of healthcare, escalating costs of research and development, and the requirement to rapidly make safe and effective new treatments available to patients. The founding members of the ICH are the EU, the US, Japan, and the UK (added post-Brexit on 16 June 2022) [Citation2]). The founding industry members were the European Federation of Pharmaceutical Industries (EFPI) and Japanese Pharmaceutical Manufacturer’s Association (JPMA), and the Pharmaceutical Research and Manufacturers of America (PhRMA). The standing regulatory members are Health Canada and Swissmedic, and 20 regional legislative authorities are also members.
The ICH guidelines provide extensive details on the quality of biological drugs. For instance, the ICHQ5E guidelines ensure the maintenance of product quality upon transfer of a biological process. This can establish a foundation for a new guideline, where the emphasis is shifted from the pre-approval cycle. We propose a new overarching guideline proposed by ICH that encompasses all other applicable guidelines and a step-by-step development program that does not include animal and efficacy testing. The ICH guideline will align better with recent changes proposed by EMA and MHRA, but as an independent organization, it will not be bound to any jurisdiction. Compliance with this new overarching guideline will be a global requirement, including with World Health Organization (WHO) guidelines, which should be merged with this guideline.
The WHO has provided advice to 194 countries [Citation3], including regulatory approval of biosimilars, but this advice remains incomplete and questioned for its scientific validity [Citation4,Citation5]. Various regional guidelines have also been developed [Citation6], such as in India [Citation7], where extensive animal toxicology and token efficacy testing are mandated. The ICH guidelines will fulfill these advising roles more effectively than the WHO guidelines.
Therapeutic proteins are produced using recombinant engineered biological agents, bacteria, and mammalian cells and are named biological drugs. A new biological drug is approved based on characterization, safety, and efficacy testing results. In addition, a biosimilar candidate is approved based on its similarity to a reference product to ensure that there is ‘no clinically meaningful difference’ [Citation8].
The first biosimilar guideline was introduced and approved by EMA in 2006 [Citation9]. The FDA proposed the biosimilar approval guidelines in 2009 [Citation10]. Last year, as the Brexit transition period ended, the MHRA published its first comprehensive guideline on 14 May 2022 [Citation11] that differs from all other guidelines by providing clear recommendations on the requirements for animal and clinical efficacy studies.
The regulatory agencies have 18 years of experience in approving and using biosimilars and have published hundreds of reports summarizing the safety and efficacy of these agents. A strong consensus has emerged [Citation12,Citation13] stating that significant amendments to the approval guidelines for biosimilars are needed not only to reduce the current cost of USD 100–300 million [Citation14] but also to enhance the safety of these products, which may be jeopardized when using studies to justify non-compliance with more sensitive studies like analytical assessment and clinical pharmacology profiling. Furthermore, reducing development costs is essential to widening access to biosimilars [Citation15,Citation16], which comprise only nine more than 150 biosimilar candidates in the US and 14 in the EU. Once the testing requirements are harmonized, many smaller companies can enter the market to fulfill the need for biosimilars.
In early years, reference product companies attempted to block the entry of biosimilars into the market; however, these efforts were unsuccessful [Citation17], and they have instead used a strategy of heavily discounting and monopolizing the market by blocking the supply of other products to distributors [Citation18]. Regarding the ‘patent dance,’ wherein a biosimilar developer must present its dossier to a reference product company to determine how to litigate, a new law [Citation19] now requires the reference product company to list patents with the FDA where litigation is imminent. This has dramatically reduced the risk of patent litigation. As a result, only a few litigations are pending, most recently against aflibercept, trastuzumab, and natalizumab [Citation20].
Biosimilarity can be determined based on a combination of analytical testing, biological assays, and clinical pharmacology comparisons but not on animal pharmacology, toxicology, or efficacy testing in patients. Therefore, unnecessary testing is tantamount to subject abuse and must be prohibited rather than merely discouraged [Citation21].
Animal testing
Animals have been frequently used in biomedical research. The early Greek physician-scientists Aristotle (384–322 BC) and Erasistratus (304–258 BC) conducted experiments on living animals. Galen (129–199/217 AD), who was influential in the history of medicine and Greek physician and practiced in Rome, also performed animal experiments to evaluate their anatomy, physiology, pathology, and pharmacology. Ibn Zuhr (Avenzoar), an Arab physician practicing in Moorish, Spain, in the 12th century, began using animals in experiments to test surgical techniques before applying these methods to human patients [Citation22]. Recently, the testing of drugs in animals has been criticized, as these studies now appear to offer low value, most notably for biological drugs. Unlike the reactive chemical groups in chemical drugs, which can interact with numerous tissues to induce a harmful response, biological drugs’ pharmacological response and toxicity depend on their receptor-binding properties. Therefore, biological drugs are not likely to elicit pharmacological or toxicological responses [Citation23]. Hence, the FDA recommends that animal testing be waived, even for new biological drugs [Citation24].
The method used for testing also contributed to the lack of relevance of toxicological findings in animals to those in humans. For instance, animal testing methods typically require administration of a large dose to elicit a toxic response. However, within these dose ranges, the responses are not linear; thus, any difference attributed to the lack of demonstrable pharmacology by biological drugs in animals cannot be observed. However, considerable futile animal testing is still being conducted for biosimilars, as demonstrated by the most recent FDA and EMA filings [Citation25].
Organoids, organs-on-chips, and in silico modeling can be used rather than animal testing models to develop more precise and dependable scientific techniques when necessary. Non-clinical in vivo testing has also been replaced by in vitro assays over the past ten years to accommodate the changes in animal protection legislation. These actions can reduce animal use. Additionally, these strategies align with the Regulatory Science Strategy of the EMA for 2025, which aims to develop a more flexible regulatory environment that supports human and veterinary health [Citation26].
The results of animal toxicology studies may be misleading if used to justify differences in impurities, post-translational modifications, or antibody reactions. To date, all biosimilar applications have included animal data [Citation27]; in many instances, the FDA has ignored these data in the review process, mainly if these data were used to justify any variability in the analytical assessment [Citation25].
The EMA and FDA have approved over 130 biosimilars [Citation28,Citation29]. The regulatory filings showed that none of these products were toxic in animals, confirming that animal testing is of little value in supporting the claim of biosimilarity [Citation30]. These observations and conclusions typically have strong scientific reasoning, but the sponsors are concerned that the study findings will eventually be disproven, resulting in a costly delay in market access.
There is remarkable awareness of the utility of animal testing for biosimilars. However, this may soon be a moot point, as the US Senate is considering a bill to prohibit the testing of biosimilars in animals. In the Biologics Price Competition and Innovation Act (BPCIA), section (bb) was amended from “(bb) animal studies (including the ‘assessment of toxicity’ to ‘an assessment of toxicity (which may rely on, or comprise, a study or studies described in item (aa) or (cc)); (aa) is an analytical assessment and (cc) in clinical testing.’ In addition, Senator Lujan of New Mexico proposed a bill in the senate to remove all references to animal testing [Citation31]. Other such bills are imminent and will lead to large amendments to the BPCIA.
Clinical efficacy
Clinical efficacy testing of new drugs is essential; however, regulatory authorities have criticized the adoption of testing protocols. For example, Dr. Janet Woodcock, the previous acting commissioner of the FDA and one of the longest-serving leaders of the Communicable Disease Emergency Response CDER) Program of the FDA has stated: ‘Why should we put patients through all these different trials just to check a box.’ The FDA also questioned this concept and stated that clinical efficacy testing is ‘broken’ [Citation32] and that real-world evidence should be promoted, as outlined in the 21st Century Cure Act [Citation33].
The clinical efficacy testing to support establishing biosimilarity is the least sensitive when testing products with highly similar analytical and clinical pharmacology profiles. The reasons for these outcomes include a low study power, requirement for a larger number of subjects than that used to approve the reference product, arbitrarily accepting a clinical difference, inability to evaluate the clinical response, nonlinearity of responses, and patient population variation. In addition, the logic of evaluating biosimilar candidates in only one efficacy study when the biosimilar product may have several indications with different mechanisms reduces such testing to merely ‘checking a box’ [Citation34], as stated by Dr. Woodcock.
Clinical efficacy studies have never demonstrated clinically significant differences between a biosimilar and its reference product or resulted in the withdrawal or recall of the reference of its biosimilar product from the market. These data are available in 96 European Public Assessment Report files and 39 approval documents obtained from the EMA [Citation35] and FDA [Citation36]. None of the 141 studies reported on clinicaltrials.gov failed to demonstrate the difference between the reference product and its proposed biosimilar product [Citation37]. In some instances, the data were reanalyzed to declare equivalence, and immunogenicity was reported in one case [Citation38,Citation39]. Additionally, from 2002 to 2022, 435 randomized controlled clinical trials were listed in the PubMed database, revealing no clinically significant difference between biosimilar candidates and their reference product and its reference product [Citation37].
The Bayesian hypothesis provides a more straightforward argument for how the aforementioned observations determine the sensitivity of clinical efficacy testing [Citation40], wherein the posterior probability determines the probability of the correctness of an observed outcome. With almost 100% posterior probability demonstrating equivalence, either the tested products are biosimilar or the study design is inadequate to determine the difference. In both instances, these tests are redundant.
In October 2022, the FDA established a pharmaceutical research program in which switching testing of biosimilars could be waived to allow the interchangeable status of biosimilars, and real-world evidence could replace the clinical testing of biosimilars. These are important steps announced by one of the most conservative regulatory agencies and provide insight into the future of biosimilar testing [Citation41].
The main reason for removing clinical efficacy testing is not to reduce the cost of development but to reduce ethical and safety concerns. Ethical concerns arise from the universal belief that healthy subjects should not be unnecessarily exposed to testing, as codified in the US 21 CFR 320.25(a)(13), which states that ‘No unnecessary human testing should be performed’ [Citation42]. In addition, risks can be attributed to using human subjects for studies that will not add any value to the evaluation of biosimilar products [Citation41,Citation42].
Proposed guideline structure
Several ICH guidelines provide scientific support for the development of biological products and should be included in the proposed overarching ICH guidelines adopted by regional agencies. In addition, ICH offers a long list of guidelines for monitoring the quality of biological products, which will become part of the overarching guidelines of the ICH [Citation43] to enable global harmonization [Citation6].
The structure of the overarching ICH guidelines is as follows. illustrates the changes in the approval process.
Figure 1. Current step-by-step approach for establishing biosimilarity (left) and the proposed plan (right). Source: Food and Drug Administration.
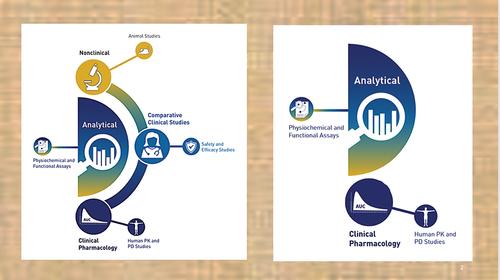
Scope. These guidelines are expected to serve as global compliance documents. Regional agencies must draft ICH guidelines. Full compliance is also expected.
Definition. A biosimilar product has the same safety, efficacy, mode of action, dose, frequency, route, and concentration (strength) as the reference product. The following products are considered.
Proteins, polypeptides, and products and derivatives that contain them, such as conjugates, drug-antibody conjugates, fixed-combination products, or any other product that was first approved in a full Biologics License Applications (BLA). Proteins and polypeptides can be purified and characterized using analytical methods and developed using recombinant or non-recombinant cell culture expression systems. An example of a non-recombinant cell culture expression system is the production of Botox.
Alpha-amino acid polymers comprising 40 or fewer amino acids are considered peptides rather than proteins. Glucagon, liraglutide, nesiritide, teriparatide, and teduglutide are peptides. A peptide is regulated as a chemical drug and copied as a generic drug.
It does not apply to other product categories, such as proteins and polypeptides that have been removed from tissues and bodily fluids.
Vaccines are considered biological products and are approved by the FDA under its CBER division, whereas CBER handles therapeutic proteins, and the concept of biosimilars applies only to CDER products. Newer mRNA vaccines are chemical products, and no consensus has been established regarding whether they can be chemical drugs [Citation44]. Theoretically, mRNA vaccines can be considered chemical drugs, and the ICH should not be concerned with how these products are classified by the FDA but should follow the EU classification [Citation45].
Reference Product. This biological product is first approved using a complete dossier in one of the four ICH original member countries (the US, EU, UK, and Japan). If the reference product has been registered in multiple member countries using the same dossier, the product should be secured from any of these countries. Only one reference source is used in the study. The supply of the reference product must be adequately documented, along with the shipping details, to ensure that the product is delivered without adverse exposure. The lowest-strength products should be selected for testing when several products with various strengths are available. Multiple batches of reference products should be directly sourced from appropriate markets with different companies. This requirement enables biosimilar developers to identify reference product variability, reflecting manufacturing variability. These steps are costly, and reference product companies have attempted to block access to their products. ICH guidelines cannot be applied because of a lack of jurisdictional authority. The reference product batches should be tested within their permitted shelf lives and maintained under the recommended (label) storage conditions. Testing batches that have been stored for a long time (e.g., frozen at-80°C) or beyond their designated shelf life may occasionally be possible if reliable data are available showing that the storage conditions do not affect the relevant quality attributes, as demonstrated in sample analysis. The age of the reference product batches (relative to their expiry dates) at the testing time must be documented during the analysis.
Characterization. The reference product is characterized using the techniques described in ICH Q6B. However, newer technologies are routinely introduced, and developers should select the most analytically sensitive methods. These characterizations include the determination of physicochemical properties, biological activity, immunochemical properties (if any), purity, impurities, contaminants, and quantity. As the quality attributes of a reference product vary between batches, it is essential to establish the ranges of these variations to allow for similar variability in biosimilar candidates. These variations are either process-related (manufacturing system) or product-related (expression system). Generally, variations in product-related attributes are not resolved, leading to the requirement for different expression systems. Process-related attributes should be modified by adjusting upstream and downstream steps, not justified by any nonclinical or clinical testing.
Impurity Profile. A biosimilar may have fewer impurities in terms of type and amount but no unmatched impurity in any amount because the impurity differences cannot be justified in safety studies unless prior reported scientific data are available to support the safety of unmatched impurities. Unmatched or higher impurities can be removed or reduced during the downstream process, but these steps may affect the yield.
Expression System. The expression system determines the product-related critical quality attributes (CQAs), including primary and secondary structures, tertiary structures, conformational stabilities, oligosaccharide patterns, glycopeptide mapping, monosaccharide/sialic acid content, size variants, charge variants, linked proteins, and product-associated variants, which are further subdivided from the primary structure (HCD). The expression system should be in the same class as that used to express the reference product, although this is not required by the FDA or EMA. The developers are also advised to select steady expression systems; typically, high-yielding cell lines produce more variability in the properties of the product. Therefore, the cell lines should be qualified according to ICH Q5D.
Primary Amino Acid Sequences. The primary amino acid sequence should be identical to the sequence found in the reference product in a side-by-testing and not from any public database or pharmacopeial listing. Some differences in the amino acid sequence are acceptable such as terminal lysine group differences. The N- and C-terminal amino acid sequences, free SH groups, and disulfide bridges should be compared appropriately. Any modifications or truncations should be quantified, and any intrinsic or expression system-related variability should be described. Any detected differences between the biosimilar and the reference medicinal product should be justified concerning the micro-heterogeneous pattern of the reference medicinal product (e.g., C-terminal lysine variability).
Post-translational Modifications (PTMs). The presence and extent of post-translational modifications (e.g., glycosylation, oxidation, deamidation, truncation) should be appropriately characterized. If present, carbohydrate structures should be thoroughly compared, including the overall glycan profile, site-specific glycosylation patterns, and site occupancy. The presence of glycosylation structures or variants not observed in the reference product would require appropriate justification, with particular attention to non-human structures (non-human linkages, sequences, or sugars). The PTMs increase the functional diversity of the proteome via the covalent addition of functional groups or proteins, proteolytic cleavage of regulatory subunits, or degradation of total proteins. These modifications include phosphorylation, glycosylation, ubiquitination, nitrosylation, methylation, acetylation, lipidation, and proteolysis and influence most aspects of normal cell biology and pathogenesis. Therefore, identifying and understanding PTMs is critical for optimizing the downstream and upstream manufacturing processes to match the PTM profile with the reference product. However, matching the PTM profile is labor-intensive and requires state-of-the-art analytical tools.
Non-enzymatic PTMs. Non-enzymatic PTMs include oxidation, phosphorylation, sulfation, acetylation, methylation, and hydroxylation products obtained during manufacturing. Liquid chromatography is preferred for characterizing PTMs and quantifying the related molecular variants and impurities.
Other Variabilities. Examples of heterogeneities introduced during the production, administration, and storage of biological products include size-based heterogeneities (aggregates, fragments, and subvisible/visible particles), charge-based heterogeneities (acidic and basic variants), and other product modifications (such as reduced, oxidized, glycated, and misfolded proteins).
During the production process of biosimilars, protein hydrophobic patches unfold because of environmental changes, resulting in aggregation or fragmentation that might alter the immunogenic property of the product. As a result, protein loss because of interactions in the stationary phase and salt-induced aggregation or dissociation is common during size-exclusion chromatography analysis. To evaluate the size distribution quantitatively, sedimentation velocity-analytical ultracentrifugation, a matrix-free substitute for size-exclusion chromatography, can be used.
Charge variations are proteo-forms that originate in various colloidal matrices (such as culture media, in-process buffers, or formulations) during different manufacturing process phases and show changing charges. Cation exchange chromatography is best suited for adjusting charge variants.
Stability. Accelerated and stress-stability investigations are required to determine the degradation profiles and enable direct assessment of the structural similarity of the biosimilar candidate with its reference product. These studies support analytical similarity assessment since the nature of molecular breakdown depends on the structure. The ICH Q5C and Q1A(R) should be considered. The long-term degradation profiles should be comparable to similar profiles of the reference product. The appearance of unmatched impurities between the biosimilar products and its reference product during the lifecycle of the biosimilar product indicates differences that should be monitored for adverse events.
Process Qualification. Upstream and downstream processes must be validated before evaluating the analytical similarity of the biosimilar candidate with its reference product. Bridging studies are required to validate changes in the production size; once clinical pharmacology studies are completed, no batch size change is allowed. The developer may conduct the bridging studies under ICHQ5E, but only after the biosimilar product has been licensed or approved. Process-related variations or residuals include cell substrates, host cell proteins, host cell DNA, cell cultures, and downstream processing residuals. The preferred methods for HCP and HCD detection and quantitation are enzyme-linked immunosorbent assays and real-time or quantitative polymerase chain reactions. However, because the variants are components of the release specification, they are not examined during the drug-substance qualification phase.
Release Specification. Tests for sterility, endotoxins, microbiological limits, container volume, uniformity of dosage units, and acceptable particulate matter are covered in the general monographs of the pharmacopeia, and these standards can be used to demonstrate compliance. Additionally, particulate matter may require comparative testing because it can be responsible for inducing immunogenic responses and are evaluated in release specification tests.
CQAs. The characteristics of the reference product that can significantly impact safety and efficacy are established and can be based on expression systems. Reference product characterization allows for the establishment of CQA for release and analytical assessment in the comparator mode. Specifications are set before analytical assessment. The reference product is characterized using appropriate testing techniques to identify its physicochemical characteristics, biological activity, immunochemical characteristics, purity, and contaminants. The lots used during the development phase can be used as test lots. However, initial clinical trials testing many pharmacokinetic (PK)/pharmacodynamic (PD) studies must include at least one. In addition, all test methods must be validated or verified if drawn from pharmacopeia. Injectable products are allowed to vary based on inevitable variabilities, such as ±3% protein content, no more than 3% impurity, no single impurity of more than 1%, or ±15% for potency testing. Pharmacopeial specifications for qualification of the dosage form, such as sterility, fill volume, delivered volume, and physical properties, are not tested for comparison purposes.
Formulation. The formulations of biosimilars can differ from those of reference products; however, these differences can only involve their inactive components. Despite variations in the constituent composition, a formulation with the same or fewer inactive ingredients is recommended unless intellectual property constraints exist. No novel excipients should be present in formulations with no history of use as reference products. Because the delivery form may have a different configuration or components, human factor studies are conducted without assumptions regarding the reference product. This precaution is necessary to avoid unexpected outcomes that are not worth the risk, as many formulation options are compatible with every biosimilar. The suitability of the formulation should be demonstrated in terms of the integrity, activity, and potency of the active ingredient as well as its stability, compatibility (i.e., how it interacts with excipients, diluents, and packaging materials), and compatibility. For example, suppose the primary packaging in contact with the product is different in its chemical properties, such as glass versus plastic. In that case, additional safety studies are required to ensure the absence of unexpected leaching of the packaging components into the product. The FDA has recently raised this issue, and detailed compatibility studies are required. Developers are advised to choose a primary packaging material that is identical in composition to the reference product to avoid the necessary studies to defend the differences. All excipients should be free of animal products.
Analytical assessment
Test Methods. Critical products and process-related variants are compared with the reference product to enable suitably but not necessarily validated methods, as some test methods cannot be fully validated. Analytical methods must be sensitive, qualified, and sufficiently discriminatory to detect possible differences. The methods used to assess the quality attributes for the batch release can also be used for analytical assessment, as detailed in the ICH guidelines (ICH Q2A, Q2B, Q5C, and Q6B), where appropriate. In addition, robust data requires the application of suitable orthogonal methods.
Number of Batches. Based on the anticipated variability, three batches of the reference product are adequate to confirm a higher-order structure. Development lots can be used for analytical assessments, but at least one at-scale Current Good Manufacturing Practice (cGMP)P is required. This lot is used for clinical testing, and regulatory filing will require a bridging study with at least three process performance qualification lots. There is discord among the agencies regarding statistical modeling; but if it is used then to assure a power of 0.18 in a t-test of the 3Sigma approach (μref-3σref and μref + 3σref), at least ten batches are required.
Data Evaluation. Non-quantitative data outputs, such as spectroscopy charts, can be compared visually. The ICH guideline Q5E and FDA guidelines provide more details, and statistical testing is gaining acceptance by the EU and WHO.
Reference Standards. To avoid confusion with the reference product, developers created a reference standard for conducting analytical assessments. For biological assays and physicochemical testing of succeeding lots, an in-house primary reference material is a suitably described sample created by the manufacturer from a representative lot or lots against which an in-house working reference material is calibrated. It is the only reference material used as the reference and working reference materials. Publicly available reference standards (e.g., Ph.D.) cannot be used as reference products to demonstrate biosimilarity. However, these standards can be used to qualify and standardize methods. No specification in any monograph for drug substances or products can be used to establish the specification of a reference product or biosimilar candidate. However, these test methods can be used for verification.
Functional Assays. Analytical and in vitro functional assays should be performed to identify CQA. Functional experiments should be pertinent to the potential mode of action for all therapeutic indications, including those examining apoptosis, complement-dependent cytotoxicity, antibody-dependent cellular phagocytosis, and cytotoxicity. A biological property should be considered applicable to the mode of action unless sufficient evidence is presented. Functional tests (antibody-dependent cell-mediated cytotoxicity, cellular phagocytosis, and complement-dependent cytotoxicity) are not required for reference products that primarily target soluble antigens.
Pharmacokinetic and Pharmacodynamic. These studies are an extension of analytical assessments that reflect how the body sees the molecule and vice versa and are required even when a product is administered intravenously. PK studies are required to assess the extent and strength of receptor binding, which may change the PK parameters, such as the distribution volume and clearance. These studies are necessary for all biosimilars, even if they are not administered via the parenteral route, such as biological drugs injected into the eye. PK/PD studies are conducted to compare rather than characterize the reference product profile and biosimilar candidate profile. This comparison can be performed in a restricted population to reduce inter- and intra-subject variability, thereby reducing the study size. All studies should conform to the standards associated with bioequivalence testing. Ideally, PK experiments should be planned and powered to demonstrate equivalence to the reference product in healthy volunteers. Crossover or parallel studies should be supported by strong designs. Although a crossover strategy is superior in identifying changes, it may not be suitable for reference products that induce strong immune responses or have long half-lives. If suitable population PK or PK/PD models for the reference product are available in the literature, modeling and simulation should be considered to optimize the study design, such as selecting the most sensitive dose(s), study population, and sample size to analyze PK differences. Linear (nonspecific) clearance and nonlinear (target-mediated) clearance should be considered through dosage selection and evaluation of partial areas under the curve. Body weight adjustments or other factors (such as subject sex) to be employed in the statistical analysis of parallel-group experiments should be predefined in the statistical analysis strategy. The equivalence margins must be pre-specified; an interval of 80.00–125.00% is generally acceptable. PK trials should demonstrate the equivalence of the primary PK parameters, typically the AUC0-∞ and Cmax. If the extrapolated portion of the AUC0-∞ comprises >20% of the total AUC0-∞ in >20% of the observations, the study’s validity should be re-evaluated. Root-cause analysis is necessary if a PK study is unsuccessful (i.e., 90% confidence intervals for the main PK parameters do not fall entirely within the prespecified acceptance limits) to modify the planning and execution of a new PK study. In most cases, the cause of study failure is subject variability, which can be reduced by selecting narrow criteria for qualification regarding sex and age. A PK trial can be used to test PD parameters, and descriptive results should be provided to support the findings of biosimilarity.
Immunogenicity. B cells and T-cells are activated to trigger immune responses. B cells are the lymphocyte population that transforms into plasma cells that produce and release antibodies in large quantities. These antibodies are immunogenic. Differences in the antibodies will not be concerning if the immunogenicity profile is different but does not affect the disposition.
Consequently, the FDA is waiting to test the immunogenicity of insulin if the product meets other analytical assessment qualifications [Citation46]. Almost all biological therapeutic proteins trigger an immunological response, developing anti-drug antibodies (ADA). Most ADAs against therapeutic monoclonal antibodies exert neutralizing effects by targeting the antigen-binding region of the therapeutic monoclonal antibody.
Nonetheless, fully human antibodies can be highly immunogenic according to the nature of the ADA response. Technically, ADA detection is complex, and all tests have limitations, including a limited ability to detect ADA in the presence of medicine because of the creation of immunological complexes (IC), which can lead to an underestimation of the incidence of ADAs. Enhanced assays that can break apart drug-ADA ICs have increased the number of ADA patients. Because of the wide variability in ADA assays, the immunogenicity of various compounds determined in different studies cannot be compared. If the ADA titer (i.e., concentration) is sufficiently high and long-lasting, the development of drug-ADA ICs can drastically modify the PK and directly decrease the medication efficacy. Although a clinical nonresponse is likely to be observed in patients with high ADA titers, free medication concentrations may still be high enough to exert effects in patients with low ADA titers. ADA also increases the risk of adverse events, including hypersensitivity reactions. ADA is present before a clinically apparent adverse reaction, demonstrating its predictive significance. Algorithms are currently available to guide therapeutic decisions in clinical practice and assist in developing safer and more cost-effective therapeutic strategies. These algorithms integrate therapeutic drug monitoring and immunogenicity information into the clinical evaluation of patients administered biologics [Citation47,Citation48]. Patients may already show a low titer of antibodies that cross-react with specific therapeutic antibodies and develop ADAs during therapy. These effects also depend on the design of the therapeutic drugs for immunogenicity [Citation49–54]. Hence, although immunogenicity testing may be redundant in certain situations, patients should be diagnostically monitored for the development of ADAs. During PK trials, data on immunogenicity and safety should be collected. Evaluation of ADA production rate, kinetics, and impact on PK (and PD) using a predetermined group study of ADA-negative and ADA-positive participants are some of these options for predicting adverse events. Although they would not replace immunogenicity assessment in PK trials, in vitro immunogenicity assays may enhance functional and analytical assessments. Short-term immunogenicity analyses may not reflect real-world experiences with biologics, including those with biosimilars. Particularly, rare ADA-related adverse events may not be detected in the premarketing phase because of the limited size of the exposed population and greater scrutiny of patient care in the clinical trial setting. Therefore, immunogenicity should be monitored in pharmacovigilance and risk management plans that also monitor other drug reactions.
Naming. Biosimilars should have a unique brand name enabling post-market pharmacovigilance.
Label. The label must state all risks associated with the reference product, present the same indications, and be formatted and detailed without exception, as described in this guidance. Once a biosimilar candidate is shown to be highly similar to the reference product, all indications granted to the reference product are allowed, provided they are not protected by market exclusivity or patents. A developer may not request fewer or more indications. The content and format of the label must comply with the FDA guidance [Citation55].
Substitution. Biosimilars can be substituted or interchanged with reference products or other approved biosimilars by using the same reference product. This conclusion assumes that biosimilars have an immunogenicity profile that is highly similar to that of a reference product. Although inter-patient differences are expected, if the specific product is known not to affect its disposition kinetics as a function of immunogenicity, concerns are reduced, even though safety concerns that may alter the general response, such as anaphylaxis, remain and require individual patient monitoring.
Pediatrics. No pediatric compliance studies are required for biosimilars.
Human Factor Studies. These are required to ensure that the correct dose is administered to patients. However, if the administration device used is very similar to that used for the reference product, the need for these studies is waived. If a device is covered under a patent, a tactic often used by innovators, other devices can be used. Of concern is the contact with the primary container, which is likely to be USP Class 1. In addition, no such studies are required when a healthcare professional administers a product.
Risk Management Plan. The risk management plan for a biosimilar product is the same as that for the reference product. Furthermore, the brand name and batch number must ensure precise biosimilar traceability. This is critical for ensuring patient safety [Citation56,Citation57]. The adverse event database in the EU and the FDA guides such instances.
Regulatory procedures
Overarching guidelines for ICH are expected to be adopted globally, enabling developers to register their products across many jurisdictions based on the same data. However, several additional considerations are required to expedite the entry of biosimilars.
Suppose a product is approved in one of the ICH countries [Citation58]. In that case, its approval should be automatic and subject to submitting a copy of the dossier that resulted in the authorization. This applies to both new and biosimilar biologics. Prescription information should conform to the label of the original license and not be modified in a local jurisdiction. The registration of these products does not require proof of tolerance in the local population. This is identical to the requirements for the country of origin. No efficacy testing is performed in the local population because these studies can never fail and waste resources.
For products approved in non-ICH countries, the regional agency should adopt an EMA evaluation system using external rapporteurs. This is not an admission of the lack of qualification of an agency to perform evaluation, but rather a means of harmonizing the quality of the dossier and giving a fair chance to the developers to assure safety and efficacy. The rapporteur submits the report to the agency and sponsor to decide whether to accept the submission. The EMA offers a list of rapporteurs who can include experienced reviewers. The developer can rebut the evaluation and challenge the findings to make them fair and transparent. The same is true for Current Good Manufacturing Practice compliance, which must be demonstrated by third-party auditors.
Conclusion
Biosimilars are among the safest categories of biological products. However, as a new class of drugs, the regulatory guidelines established to demonstrate their comparable safety and efficacy have resulted in complex and costly pathways proposed by major regulatory agencies, wherein redundant testing, such as animal and efficacy studies, are routinely required. Although the EMA, MHRA, and FDA have relaxed many testing requirements, regulatory agencies lacking the expertise to evaluate the registration submission have insisted on continuing stricter compliance with EU or FDA guidelines. This practice has limited the development of indigenous biosimilars because of its high costs and time. The guidance presented in this study will allow for faster biosimilar approval without compromising safety or efficacy. The ICH is an independent authority that should be able to create universal guidelines for approving biosimilars.
Although many ICH guidelines are routinely used worldwide, biosimilar development guidelines can expedite the entry of biosimilars based on compliance with one guideline. This guideline will also permit global registrations of products developed and sold anywhere in the world. However, the success of this approach depends on the willingness of regional agencies to adopt it. For example, the FDA and EMA have regulatory systems unlikely to change soon; in contrast, an ICH-compliant dossier for a biosimilar product may gain wider acceptance. Moreover, adopting ICH guidelines by regional regulation associations such as the Gulf Cooperation Council and the association of MENA countries or LATAM members would significantly impact healthcare globally. Therefore, it would be desirable for leading countries, such as Saudi Arabia, Brazil, and India, to take the lead in this program.
Now, the responsibility lies with the ICH; a formal request has been submitted to the ICH by the authors, and we hope this publication will draw the interest of stakeholders and agencies alike.
Disclosure statement
No potential conflict of interest was reported by the author(s).
Additional information
Funding
References
- ICH. https://www.ich.org/page/mission Cited 2022 Jul 10)
- UK Government. UK joins ICH. https://www.gov.uk/government/news/mhra-joins-international-partnerships-to-set-global-standards-for-medicines-and-medical-devices-regulation–2 (Accessed 2022 Jul 10
- WHO Guidelines on Evaluation of Biosimilars. Replacement of Annex 2 of WHO Technical Report Series, No. 977 https://www.who.int/publications/m/item/guidelines-on-evaluation-of-biosimilars.WHO. (Cited 2022 Jul 10)
- Niazi S The WHO Biosimilar guidance is based on weak science. https://www.centerforbiosimilars.com/view/who-biosimilar-guidance-is-based-on-weak-science. Cited 2022 Jul 10)
- Niazi S Opinion: one step forward, half step back. https://www.centerforbiosimilars.com/view/opiniononestepforwardhalfastepbackforwhobiosimilarguidance; (Accessed on 2022 Jun 8).
- Global Biosimilar Guidelines. https://www.gabionline.net/reports/Guidelines-for-biosimilars-around-the-world (Accessed 2022 Jul 10
- CDSCO, India. https://cdsco.gov.in/opencms/resources/UploadCDSCOWeb/2018/UploadAlertsFiles/BiosimilarGuideline2016.pdf; Accessed on 2022 Jun 8)
- FDA. Biosimilars and Interchangeable Biosimilars. [cited 2022 Aug 24]. https://www.fda.gov/drugs/biosimilars/biosimilar-and-interchangeable-products
- EMA Centrally Approved Biosimilars. https://www.ema.europa.eu/en/medicines/field_ema_web_categories%253Aname_field/Human/ema_group_types/ema_medicine/field_ema_med_status/authorised-36/ema_medicine_types/field_ema_med_biosimilar/search_api_aggregation_ema_medicine_types/field_ema_med_biosimilar; (Cited Jun 8, 2022).
- US Congress. Title VII—improving access to innovative medical therapies subtitle a—biologics price competition and innovation. US Congress. Available online: https://www.fda.gov/media/78946/download accessed on 2022 Mar 23)
- MHRA. Biosimilar Guidance. https://www.gov.uk/government/publications/guidance-on-the-licensing-of-biosimilar-products/guidance-on-the-licensing-of-biosimilar-products; (Accessed on 2022 Jun 8).
- Niazi SK. The Coming of Age of Biosimilars: a Personal Perspective. Biologics. 2022;2(2):107–12. [cited 2022 Aug 24].
- Niazi SK. Biosimilars: a futuristic fast-to-market advice to developers. Expert Opin Biol Ther. 2022;22(2):149–155.
- Chen Y, Monnard A, Jorge Santos Da S An Inflection Point for Biosimilars, McKinsey & Co. cited 2021 Oct 12]. Available from 2021 Oct 12: https://www.mckinsey.com/industries/life-sciences/our-insights/an-inflection-point-for-biosimilars (Accessed on 2022 Jun 8).
- Blackstone EA, Joseph PF. The economics of biosimilars. Am Health Drug Benefits. 2013 Sep;6(8):469–478. PMID: 24991376; PMCID: PMC4031732.
- Khalifeh I, et al. Adherence, Persistence, and Expenditures for High-Cost Anti-Inflammatory Drugs in Rheumatoid Arthritis: an Exploratory Study Volume 25 Issue (4). J Manag Care Spec Pharm. 2019 Apr;25(4):461–467.
- Pfizer Press Release. https://www.pfizer.com/news/press-release/press-release-detail/pfizer_goes_to_court_to_allow_competition_for_biologics_and_expand_options_for_patients (Accessed 2022 Jul 10
- Tactics to block biosimilars. https://www.biopharmadive.com/news/fda-ftc-biosimilar-competition-consumer-antitrust-laws/573745/ (Accessed 2022 Jul 10
- US Government Biosimilar Bills. https://rules.house.gov/sites/democrats.rules.house.gov/files/BILLS-116HR133SA-RCP-116-68.pdf (Accessed 2022 Jul 10
- Biosimilar Patent Litigation status. [cited 2022 Aug 14]. https://www.bigmoleculewatch.com/bpcia-patent-litigations/
- Niazi S. End Animal Testing for Biosimilar Approvals. Science. 2022;377(66020):162–163.
- Hajar R. Animal testing and medicine. Heart Views. 2011;12(1):42. PMID: 21731811; PMCID: PMC3123518.
- Norman GV. Limitations of animal studies for predicting toxicity in clinical trials: is it time to rethink our current approach? Jacc. 2019;4(7):845–854.
- Avila AM, Bebenek I, Bonzo JA, et al. An FDA/CDER perspective on nonclinical testing strategies: classical toxicology approaches and new approach methodologies (NAMs). Regul Toxicol Pharmacol. 2020;114(104662):0273–2300.
- Niazi S Analysis of FDA-licensed biosimilars–time for paradigm shift. https://www.centerforbiosimilars.com/view/analysis-of-fda-licensed-biosimilars-time-for-a-paradigm-shift Cited 2022 Jul 10)
- EMA Regulatory Science to 2025 https://www.ema.europa.eu/en/documents/regulatory-procedural-guideline/ema-regulatory-science-2025-strategic-reflection_en.pdf Cited 2022 Jun 8
- Moore TJ, et al. Assessment of availability, clinical testing, and US Food and Drug Administration review of biosimilar biologic products. JAMA Intern Med. 2020 Accessed on 2022 Jun 8. DOI:10.1001/jamainternmed.2020.3997
- FDA. biosimilar Product Information. [cited 2022 Aug 24]. https://www.fda.gov/drugs/biosimilars/biosimilar-product-information
- Biosimilars Approved in Europe. https://www.gabionline.net/biosimilars/general/biosimilars-approved-in-europe (Accessed 2022 Jul 10
- Niazi S Debate over animal toxicology studies. https://www.centerforbiosimilars.com/view/opinion-the-debate-over-animal-toxicology-studies (Accessed 2022 Jul 10
- Niazi SK, Contributor No Animal Testing of Biosimilars—US Congress Begins Amendment to BPCIA https://www.centerforbiosimilars.com/view/contributor-no-animal-testing-of-biosimilars-us-congress-begins-amendment-to-bpcia. Cited 2022 Jul 10)
- Woodcock. https://www.biopharmadive.com/news/fdas-woodcock-the-clinical-trial-system-is-broken/542698/ (Accessed 2022 Jul 10
- US Congress. https://www.govinfo.gov/content/pkg/STATUTE-98/pdf/STATUTE-98-Pg1585.pdf (Accessed 2022 Jul 10
- Woodcock J, on clinical trial status. https://endpts.com/fdas-janet-woodcock-the-clinical-trials-system-is-broken/ (Accessed 2022 Jul 10
- Biosimilar EPAR Program EMA. https://www.ema.europa.eu/en/search/search/field_ema_web_categories%253Aname_field/Human/search_api_aggregation_ema_medicine_types/field_ema_med_biosimilar?search_api_views_fulltext=epar%20biosimilar (Accessed 2022 Jul 10
- FDA. Biosimilars Approval Documents. https://www.accessdata.fda.gov/scripts/cder/daf/index.cfm Cited 2022 Jul 10)
- Clinical Trial Database. https://clinicaltrials.gov/ct2/results?term=biosimilar&age_v=&gndr=&type=&rslt=With&Search=Apply (Accessed 2022 Jul 10
- Schiestl M, Ranganna G, Watson K, et al. The Path Towards a Tailored Clinical Biosimilar Development. BioDrugs. 2020 Jun;34(3):297–306.
- Biosimilar Clinical Trials @PubMed. https://pubmed.ncbi.nlm.nih.gov/?term=biosimilar+clinical+trial). Cited 2022 Jul 10)
- Niazi S. Scientific Rationale for Waiving Clinical Efficacy Testing of Biosimilars. Drug Des Devel Ther. 2022;16:2803–2815. PMID: 36043044; PMCID: PMC9420434.
- FDA. Biosimilar Science and Research. https://www.fda.gov/drugs/biosimilars/biosimilars-science-and-research (Accessed 2022 Jul 10
- FDA. Bioequivalence and Bioavailability Testing. https://www.accessdata.fda.gov/scripts/cdrh/cfdocs/cfcfr/CFRSearch.cfm?fr=320.25 (Accessed 2022 Jul 10
- ICH Quality Guidelines. https://www.ich.org/page/quality-guidelines. (Accessed 2022 Jul 10.
- Niazi S Biosimilar mRNA Vaccines. https://www.centerforbiosimilars.com/view/biosimilar-mrna-vaccines-part-1-regulatory-revolution- (Accessed 2022 Jul 10
- FDA. Innovations for Biological Products. https://www.fda.gov/news-events/fda-voices/ensuring-innovation-and-competition-biologics-leads-more-timely-products-patients. (Accessed 2022 Jul 10
- FDA. Clinical Immunogenicity Concerns. Insulin https://www.fda.gov/media/133014/download Cited 2022 Jul 10)
- FDA Multiple Clinical Endpoints. https://www.fda.gov/media/162416/download Cited 2022 Jul 10)
- Garcês S, Demengeot J, Puig L, et al. Adverse Reactions to Biologics. Curr Probl Dermatol. 2018;53:37–48.
- Atiqi S, Hooijberg F, Loeff FC, et al. Immunogenicity of TNF-Inhibitors. Front Immunol. 2020;11:312. PMID: 32174918; PMCID: PMC7055461.
- Vaisman-Mentesh A, Rosenstein S, Yavzori M, et al. Molecular Landscape of Anti-Drug Antibodies Reveals the Mechanism of the Immune Response Following Treatment With TNFα Antagonists. Front Immunol. 2019;10:2921. PMID: 31921180; PMCID: PMC6930160.
- Benucci M, Grossi V, Manfredi M, et al. Laboratory monitoring of biological therapies in rheumatology: the role of immunogenicity. Ann Lab Med. 2020;40(2):101–113. PMID: 31650726; PMCID: PMC6822010.
- Parikh CR, Ponnampalam JK, Seligmann G, et al. Impact of immunogenicity on clinical efficacy and toxicity profile of biologic agents used for treatment of inflammatory arthritis in children compared to adults. Ther Adv Musculoskelet Dis. 2021;13:1759720X211002685. PMID: 34188697; PMCID: PMC8212384.
- EMA Immunogenicity Considerations. https://www.ema.europa.eu/en/documents/presentation/presentation-immunogenicity-therapeutic-antibodies-gertjan-wolbink_en.pdf (Accessed 2022 Jul 10
- Garcês S, Demengeot J, Benito-Garcia E. The immunogenicity of anti-TNF therapy in immune-mediated inflammatory diseases: a systematic review of the literature with a meta-analysis. Ann Rheum Dis. 2013;72(12):1947–1955.
- FDA. Biosimilars Labeling Requirements. https://www.fda.gov/media/96894/download Cited 2022 Jul 10)
- EudraVigilence-European database of suspected adverse drug reaction reports. https://www.adrreports.eu/en/index.html Cited 2022 Jul 10)
- FDA Adverse Events Reporting System (FAERS) https://fis.fda.gov/sense/app/95239e26-e0be-42d9-a960-9a5f7f1c25ee/sheet/7a47a261-d58b-4203-a8aa-6d3021737452/state/analysis (Accessed 2022 Jul 10
- Word Health Organization List of SRA countries. https://www.who.int/initiatives/who-listed-authority-reg-authorities/SRAs (Accessed 2022 Jul 10