ABSTRACT
Background
Exercise is recommended for all patients with COPD. Evidence for its benefit is considerably weaker in the more severe stages of the disease. The aim of this study was to investigate whether high-intensity interval training could improve exercise capacity, pulmonary hemodynamics and cardiac function in patients with severe COPD and hypoxemia.
Methods
Stable patients with COPD GOLD stage III or IV and hypoxemia were included. They underwent extensive cardiopulmonary testing including right heart catheterization, lung function tests, echocardiography and 6-minute walk test before and after completion of 10 weeks of high-intensity interval training performed with supplemental oxygen. Primary endpoint was change in pulmonary artery pressure measured by right heart catheterization.
Results
Ten patients with very severe airflow obstruction, mean FEV1 28.7% predicted and mean FEV1/VC 0.39 completed the exercise programme. Pulmonary artery pressure remained unchanged following the intervention (26,3 mmHg vs. 25,8 mmHg at baseline, p 0.673). Six-minute walk distance improved by a mean of44.8 m (p 0.010), which is also clinically significant. We found marginally improved left ventricular ejection fraction on echocardiography (54.6% vs 59.5%, p 0.046).
Conclusion
High-intensity interval training significantly improved exercise capacity while pulmonary hemodynamics remained unchanged. The improvement may therefore be due to mechanisms other than altered pulmonary artery pressure. The increase in ejection fraction is of uncertain clinical significance. The low number of patients precludes firm conclusions.
Introduction
COPD is increasingly recognized as a multi-organ disease with extrapulmonary manifestations such as heart, muscle and skeletal dysfunctions [Citation1]. Patients with COPD, especially those with hypoxemia, are prone to developing right ventricular (RV) dysfunction and pulmonary hypertension (PH), the former even in the absence of significant PH [Citation2]. Both PH and exercise limitation are among the factors related to increased morbidity and mortality in COPD [Citation3–5]. Exercise capacity has been shown to be a better predictor of mortality than lung function [Citation6]. Exercise training is a cornerstone in pulmonary rehabilitation and is recommended in international guidelines for patients with COPD irrespective of severity [Citation7–10]. Evidence for its benefit is, however, considerably weaker for the more severe stages of the disease and for patients with chronic respiratory failure [Citation10].
Thus, there is a lack of data on the benefits of physical exercise including high-intensity interval training (HIIT) in patients with severe COPD and concomitant hypoxemia. In particular, there are no previous studies on the effect of HIIT on pulmonary hemodynamics including pulmonary artery pressure (PAP). It is not even established whether HIIT has beneficial or harmful effects on pulmonary hemodynamics in these patients. We hypothesized that HIIT would be effective in improving pulmonary hemodynamics and exercise capacity and examined the effects of a 10-week exercise programme on pulmonary circulation, exercise capacity and cardiac function in a group of patients with severe COPD and hypoxemia. The primary outcome was change in PAP measured by right heart catheterization (RHC). Secondary outcomes included changes in other pressure measurements from RHC as well as cardiac output and pulmonary vascular resistance, and changes in cardiac function evaluated by echocardiography, exercise capacity, lung function and quality of life.
Materials and Methods
Subjects and study design
Clinically stable patients with COPD and severe or very severe airflow obstruction, i.e. GOLD grades III or IV, and hypoxemia were recruited from the outpatient clinic (). Hypoxemia was defined as either previously having qualified for long-term oxygen therapy (LTOT) according to established criteria [Citation11] or demonstrating a significant desaturation defined as a drop of >4% to below 90% [Citation12] on a standardized 6-minute walk test (6MWT). For practical purposes, patients had to live within an hour from the hospital where the exercise programme was performed on an outpatient basis.
Figure 1. The recruitment process
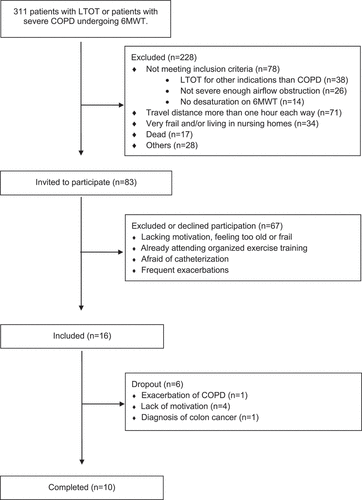
Exclusion criteria were 1) exacerbation of COPD or use of systemic corticosteroids in the 6 weeks prior to enrolment, 2) medical conditions that prohibited exercise on a treadmill, 3) already attending organized exercise training or participation in a pulmonary rehabilitation programme in the last 3 months.
All participants were examined by a physician, received verbal and written information and signed informed consent before undergoing any testing. Their medication was unchanged throughout the study period. Patients were tested both at baseline and after the exercise intervention. Baseline demographics and respiratory physiology are presented in the left panel of . Further baseline characteristics are presented in the appendix ().
Table 1. Baseline characteristics
The study was approved by the regional ethics committee (2013/2129) and registered in clinicaltrials.gov (NCT03092622).
Exercise training
Groups of 2–4 patients exercised 3 times a week over a 10-week period by walking on a treadmill (Sportsmaster T300 or T610, Nordic Sportsmaster AS, Nesbru, Norway). Each session consisted of 15 minutes warm up, 4 × 4 minutes intervals with a 3-minute active rest period between each interval and a 5-minute cool-down period. All participants received oxygen (Linde Integrated Valve (LIV®) cylinders, Linde plc, Guildford, UK) at a flow rate of 6 L/minute via a facemask (OxyMaskTM, Southmedic, Ontario, Canada). Heart rate and Borg score were recorded at the end of each interval with an aim of heart rate approximately 90% of the predicted maximum and Borg score 5–7 on the modified Borg scale [Citation13]. During the 3-minute active rest phase, the intensity was lowered to aim for a heart rate of 50–60% of the predicted maximum. The speed and/or incline were gradually and individually increased to maintain the desired intensity throughout the exercise programme. A respiratory physiotherapist supervised every session to give instructions, encouragement and ensure the safety of participants. A respiratory physician was also available throughout. Patients who failed to attend on more than 3 occasions (i.e. >10% of the sessions) were excluded from further participation.
Pulmonary function tests
Spirometry and diffusing capacity of the lung for carbon monoxide (DLCO) (MasterScreen Pneumo, CareFusion, Yorba Linda, CA, USA) were performed by trained personnel according to international standards [Citation14,Citation15]. All listed FEV1 values are post bronchodilator.
Exercise tests
These were performed at baseline and within a week from completion of HIIT.
6MWT: The track consisted of two cones placed 25 m apart in a long corridor. The test was otherwise performed according to the current standards [Citation16]. All patients were familiar with the test and setup from previous visits to the clinic. For patients already on LTOT, the test was performed with their previously prescribed oxygen equipment and flow. Patients without home-oxygen performed the test breathing room air.
Incremental shuttle walk test (ISWT): Two cones placed 9 m apart made up a 10-metre looped course. This test has a pre-set and incremental pace where subjects need to reach the next cone within a specific time represented by bleeps from an audio recording. The test was stopped when subjects were unable to continue or did not reach within half a metre of the cone for a second successive time as described in guidelines [Citation16]. ISWT was performed with supplemental oxygen at a flow rate of 6 L/minute for all patients. Participants underwent two tests, minimum 30 minutes apart, and the best test was used as reference.
Right heart catheterization
RHC was performed at baseline and 3–5 days after completion of the exercise intervention, using a 7 French Swan-Ganz thermodilution catheter (Edwards Lifescience 131 F7) with Edwards closed injectate delivery system for cold injectate (93,600) for thermodilution measurements. For hemodynamic recordings, we used Siemens Sensis Recording system for cath. labs. Pressure curves, pressures and cardiac output were recorded/computed using Siemens Axion Sensis XP system. Pressures/pressure curves were recorded during expiration and registered in the following positions: right atrium, RV, pulmonary artery and pulmonary wedge position. Cardiac output was measured using thermodilution technique, five measurements were made, the lowest and highest values discarded and the average of the remaining three used.
Echocardiography
All the participants underwent echocardiography (Vivid E9, GE, Vingmed, Horten, Norway) on the same days as RHC. Datasets were analysed offline (EchoPac 202, GE, Vingmed) by one independent observer blinded to clinical and exercise information. Left ventricular (LV) ejection fraction was assessed by the Simpson biplane method. LV dysfunction was defined as ejection fraction <54% in females and 52% in males [Citation17]. RV function and dimensions were assessed by fractional area change, spectral pulsed wave tissue Doppler imaging derived tricuspid lateral annular systolic velocity wave (S`), tricuspid annular plane systolic excursion (TAPSE), RV basal and midcavity diameter [Citation18]. RV wall thickness was obtained from the subcostal window by 2-dimensional echocardiography in end diastole and hypertrophy defined as RV free wall >5 mm. RV dysfunction was defined as fractional area change ≤40%, TAPSE <17 mm, S` <9.5 cm/sec [Citation17]. RV dilation was defined as RV basal diameter >41 mm.
Quality of life
Both COPD Assessment Test (CAT) and St. George's Respiratory Questionnaire for COPD (SGRQ-C) have been previously validated [Citation19,Citation20] and exist in officially translated Norwegian versions. SGRQ-C is a shorter version of SGRQ with scores that are comparable [Citation20]. Permission to use both CAT and SGRQ-C has been officially granted.
Blood tests
This was performed using standard venepuncture prior to RHC and analyzed for troponin T, N-terminal pro b-type natriuretic peptide and high-sensitivity c-reactive protein (hsCRP).
Statistical analysis
Baseline data are presented as mean (standard deviation) unless otherwise specified. Subjects served as their own controls for the statistical analysis. We used either paired t-test or Wilcoxon’s signed rank test depending on whether or not the normality assumption was considered met after assessing Q–Q plots and Shapiro–Wilk test. Results are given with p-value and 95% confidence interval (CI) unless otherwise stated. The threshold for statistical significance was set at p <0,05. For statistical analysis, we used IBM SPSS Statistics, version 26.
Accurate power calculations were challenging since this was the first study of its kind. Previous catheterization studies in patients with severe COPD, albeit methodologically different, had shown a mean PAP of 26–27 mmHg [Citation21,Citation22]. We estimated a 10% change in the primary endpoint and also regarded a change of this magnitude necessary to be clinically important. With 80% power, we projected a sample size of 12 patients for the intended study model. A calculated dropout rate of 20% thus led to the aim of including a total of 15 patients.
Results
Subjects and study design
Ten of the 16 included subjects completed the exercise programme with a mean attendance of 29.1 sessions. Four of the six participants who did not complete the exercise programme were LTOT-users. Demographics, medication and lung function are presented in the right panel of .
Pulmonary function tests
Participants had very severe airflow obstruction at baseline with mean FEV1 28.7% of predicted. Dynamic lung volumes and DLCO remained unchanged following HIIT (data not shown).
Exercise tests
Following HIIT the 6MWD () improved significantly by 44.8 m (p 0.010, CI 13.7–75.8). ISWT improved by 14.5 m, which was not significant (). One patient did not perform post-intervention exercise tests due to hip pain after a fall.
Table 2. Comparison of exercise tests, questionnaires and blood tests before and after the exercise intervention
Right heart catheterization
In our study population, the baseline PAP was mildly elevated with a mean PAP (mPAP) of 25.8 mmHg (10.3). Two patients had mPAP >35 mmHg, which is defined as severe PH in COPD [Citation23]. Capillary wedge pressure was within normal range with a mean value of 8.7 mmHg (4.6). RV pressures were elevated, primarily the systolic pressure with a mean value of 41.7 mmHg (16). Pulmonary vascular resistance was elevated at 297.4 dynes × sec × cm−5 (90.1), which is equivalent to approximately 3.7 Wood units. Total pulmonary resistance was elevated at 434.5 dynes × sec × cm−5 (106.6), equivalent to approximately 5.4 Wood units. Cardiac output and cardiac index were in the lower range of normal with mean values of 4.59 L/min (1.0) and 2.69 (0.47), respectively.
Overall, PAP and the other parameters measured by RHC remained unchanged after the exercise intervention (). However, in which depicts each individual patient’s mPAP before and after the exercise intervention, one can observe that for the six patients with elevated baseline mPAP >20 mmHg [Citation24] there was a trend towards reduced mPAP after the exercise intervention. The opposite can be observed for those with a normal mPAP at baseline, where three out of the four patients with mPAP ≤20 mmHg had increased values post intervention. Both of these observations are, however, not statistically significant.
Table 3. Comparison of parameters from right heart catheterization and echocardiography before and after the exercise intervention
Echocardiography
At baseline, RV function assessed by fractional area change, TAPSE and S` was within normal range with mean values of 41.3% (5.4), 22.3 mm (4.0) and 12.3 cm/sec (3.6), respectively. RV basal diameter was above normal at 42.3 mm (8.1), indicating mild dilatation. Patients had LV ejection fraction of 54.4% (2.6).
After HIIT, we observed no changes in the RV parameters (). However, LV ejection fraction increased significantly (Z = −1.992, p = 0.046) with the median value improving from 54.6% to 59.5%.
Some echocardiographic measurements could not be obtained due to technically challenging sonographic conditions.
Quality of life
Patients had considerable symptoms at baseline with a mean CAT-score of 20 (6.3) and SGRQ-C total score of 52.8 (13.2). We observed no significant changes after the intervention ().
Blood tests
Changes in cardiac markers and hsCRP were not significant ().
Discussion
This is, to our knowledge, the first study where RHC is used to evaluate pulmonary hemodynamics, including PAP, in response to HIIT in patients with severe COPD and hypoxemia. Our main findings were as follows: 1) HIIT is effective and improves exercise capacity as evaluated by 6MWT in patients with COPD and hypoxemia, 2) PAP and other parameters from RHC remained unchanged after HIIT and 3) LV ejection fraction improved in response to HIIT.
Our findings suggest that exercise training in elderly patients with severe COPD, concomitant hypoxemia and co-morbidities seems feasible and effective, although a recent meta-analysis reported that severity of disease was related to a lessened effect of aerobic training [Citation25]. The improvement in exercise capacity in our study is not only statistically, but also clinically significant as the minimal important difference in 6MWD is reported to be 30 m [Citation16]. Direct measurement of oxygen uptake at maximal exercise using cardiopulmonary exercise tests is considered the gold standard measure of cardiopulmonary fitness [Citation26]. This is, however, difficult to perform in patients dependent on supplemental oxygen. 6MWT elicit a peak oxygen uptake similar to cardiopulmonary exercise tests [Citation16]. Furthermore, the 6MWT have lower ventilatory requirements and may therefore be better tolerated in patients with severe COPD [Citation16]. ISWT, in contrast, did not improve significantly. Some of the frail, elderly participants seemed limited by the walking speed required for this test rather than breathlessness, which potentially could underestimate their functional capacity. A similar interpretation has also been made in an earlier report of heart failure patients with severe disease and disability comparing 6MWT and ISWT [Citation27]. Despite improvement in exercise capacity assessed by 6MWT, we did not observe changes in questionnaires pertaining to symptoms and quality of life.
Since PH in COPD is associated with worse prognosis, increased admission rates to hospital and reduced exercise capacity [Citation3,Citation4,Citation28], a reduction in PAP could be postulated as being beneficial. In our study, subjects had on average mildly elevated mPAP at baseline and only two patients exhibited severe PH. This is consistent with other studies, e.g. two larger catheterization studies also examining patients with severe COPD [Citation21,Citation22]. In a study of patients with PH of other causes than COPD, namely PH groups 1 and 4, a rehabilitation programme had effect on pulmonary hemodynamics, including a 7,3% reduction in mPAP [Citation29]. Patients with primary pulmonary arterial hypertension and chronic thromboembolic PH might, however, have different pathophysiological mechanisms for developing and alleviating PH compared to patients with COPD, suggested for instance by the recommendation of targeted medical therapy for selected patients in PH groups 1 and 4, but not for PH secondary to lung disease [Citation23]. Furthermore, these patients had a higher mPAP at baseline with a mean value of almost 40 mmHg and the comprehensive rehabilitation programme was also longer, spanning over a total of 15 weeks [Citation29]. One reason for the absence of effects on pulmonary hemodynamics after HIIT in our patients could be the mildly elevated PAP at baseline where only 6 out of 10 patients had elevated PAP. It is noteworthy that, although not statistically significant, 5 of the 6 patients with increased mPAP at baseline had decreased or unchanged values post intervention, while 3 of 4 patients with normal mPAP at baseline had increased values after the intervention. It is therefore possible to hypothesise that the intervention is beneficial for those with pulmonary hypertension and may be harmful for those with normal mPAP at baseline. Especially the latter of these interpretations is however controversial and also speculative. A larger study group will be necessary to explore this hypothesis.
It is also important to scrutinize the exercise intervention. The optimal mode of exercise for patients with COPD is unclear. Moderate continuous training has not shown better effects than interval training [Citation30]. For patients with severe COPD, hypoxemia can be a limiting factor in achieving the desired exercise intensity [Citation31]. Interval training with supplemental oxygen was therefore chosen for our patients. There is, similarly, no consensus on the optimum length of pulmonary rehabilitation [Citation8,Citation10]. Six to twelve weeks is recommended [Citation10], but longer programmes may have more sustained benefits. A recent systematic review [Citation25] suggests improved peak oxygen uptake for exercise programmes >12 weeks duration. Ultimately, the effects on pulmonary hemodynamics have to our knowledge never been studied in the current manner before and we cannot exclude that a longer intervention phase is necessary to observe changes in these parameters.
The improvement in exercise capacity in our study cannot be explained by changes in pulmonary hemodynamics or lung function and is therefore likely due to other mechanisms. Ejection fraction has previously been shown to improve after interval training in e.g. heart failure [Citation32] and moderate COPD [Citation33], and the improvement found in our study may be a mechanism explaining the increased exercise capacity. However, echocardiography in patients with COPD and hyperinflation is known to be difficult [Citation34] and the observed increase in ejection fraction, although statistically significant, is of uncertain clinical significance. In addition, as no statistical correction was made for multiple comparisons, this result should be interpreted with further caution. Peripheral adaptation of skeletal muscle [Citation35], which could be due to e.g. improved skeletal muscle oxidative capacity [Citation36], an increase in type I and IIa muscle fibres [Citation37], or a combination of the two or other factors, may also be an explanation for the improved functional performance. On a cellular level, it could be speculated that one linking mechanism might be endothelial dysfunction, which has been implicated in the pathophysiology and attenuation of several extrapulmonary effects in animal [Citation38] and human studies [Citation39]. The exact mechanisms for improvement in exercise capacity in our study remain unclear.
The main strength of this study is its novelty in using RHC, the gold standard investigation, to evaluate effects on pulmonary circulation after an exercise intervention in subjects with COPD and hypoxemia.
A limitation is the small sample size and the risk of type II error. We experienced a drop-out rate higher than expected, which may partly be due to the invasiveness of the procedures. Inclusion of two or more patients to meet the projected sample size in the power calculations would not have altered the outcome. It would presumably only have served to increase precision i.e. by narrowing the CI. Where the effect size was around 10%, like for the 6MWD, the sample size was sufficient to indicate significant effects.
The patients received supplemental oxygen with a flow of 6 L/minute during the training interventions. The administration of oxygen may be considered an intervention in itself. The potential effect of supplemental oxygen is, however, difficult to quantify in this study.
Selection bias is an issue, but candidates already attending organized exercise training on a regular basis or had participated in pulmonary rehabilitation during the last 3 months before enrolment were excluded.
There was no control group, but we considered it neither ethical nor feasible for a control group to undergo RHC twice without a treatment intervention. Furthermore, changes in the natural progression of the disease were unlikely to occur during the short intervention phase.
We examined a heterogeneous group with regard to phenotype, co-morbidities, and baseline oxygen requirements. This heterogeneity of a real-life population could be viewed as a strength as well as a limitation.
For future research, it would be interesting to perform a similar study focusing on COPD-patients with PH and also consider a longer intervention phase. Preferably, the number of patients should be large enough to study both patients with normal mPAP and elevated mPAP separately. Our study might serve as pilot study in the sense that an effect size of 10% might be unrealistic with regard to PAP in this patient population. Addition of muscle biopsies and measures of endothelial function and systemic inflammation could possibly further elucidate some of the underlying mechanisms at work in these patients.
The clinical implications of this study might support that HIIT in selected patients with severe COPD and concomitant hypoxemia is feasible and effective with regard to exercise capacity and without obvious harmful effects on the pulmonary circulation.
Conclusion
HIIT led to a significant improvement in 6MWD in this study population of COPD-patients with very severe airflow obstruction and concomitant hypoxemia. PAP and other pulmonary hemodynamic measurements assessed by RHC remained unchanged. However, it is not possible to draw firm conclusions from our study due to the low number of patients and therefore lack of power. Still, we consider this study relevant as it may generate further hypotheses and facilitate the planning of future studies. It has indicated the importance of separately studying patients with normal and in particular elevated PAP and may also be of help in power calculations for future studies. Our study might thus serve as proof of concept for a larger study focusing on patients with COPD and pulmonary hypertension,
Acknowledgments
We thank the following units at St. Olavs University Hospital: Pulmonary Medicine Outpatient Clinic, Clinical Research Facility, Cardiac Catheterization laboratory and Echocardiographic laboratory.
Disclosure statement
The authors report no conflict of interest.
Additional information
Funding
Notes on contributors
Lars Aakerøy
Lars Aakerøy, MD is a Consultant in Respiratory Medicine at St. Olavs University Hospital and a PhD-candidate at the Norwegian University of Science and Technology.
Ester Alfer Nørstebø
Ester Alfer Nørstebø, PT, is a specialist respiratory physiotherapist at St. Olavs University Hospital.
Karen Marie Thomas
Karen Marie Thomas, PT, is a specialist respiratory physiotherapist at St. Olavs University Hospital.
Espen Holte
Espen Holte, MD, PhD, is a Consultant cardiologist at St Olavs University Hospital and researcher at the Department of Circulation and Medical Imaging, Norwegian University of Science and Technology.
Knut Hegbom
Knut Hegbom, MD, is a specialist in cardiology with a special interest in interventional cardiology. He is a Consultant cardiologist and Head of the Cardiac Catheterization laboratory at St. Olavs University Hospital.
Eivind Brønstad
Eivind Brønstad, MD, PhD, is a Consultant in Respiratory Medicine at St. Olavs University Hospital and Associate Professor at the Norwegian University of Science and Technology.
Sigurd Steinshamn
Sigurd Steinshamn, MD, PhD, is a Consultant in Respiratory Medicine at St Olavs University Hospital and Professor at the Norwegian University of Science and Technology. His areas of interest include COPD, exercise training and sleep-related respiratory disorders.
References
- Barnes PJ, Celli BR. Systemic manifestations and comorbidities of COPD. Eur Respir J. 2009 May;33(5):1165–11.
- Hilde JM, Skjørten I, Grøtta OJ, et al. Right ventricular dysfunction and remodeling in chronic obstructive pulmonary disease without pulmonary hypertension. J Am Coll Cardiol. 2013 Sep 17;62(12):1103–1111.
- Kessler R, Faller M, Fourgaut G, et al. Predictive factors of hospitalization for acute exacerbation in a series of 64 patients with chronic obstructive pulmonary disease. Am J Respir Crit Care Med. 1999 Jan;159(1):158–164.
- Oswald-Mammosser M, Weitzenblum E, Quoix E, et al. Prognostic factors in COPD patients receiving long-term oxygen therapy. Importance of pulmonary artery pressure. Chest 1995 May;107(5):1193–1198.
- McGhan R, Radcliff T, Fish R, et al. Predictors of rehospitalization and death after a severe exacerbation of COPD. Chest. 2007 Dec;132(6):1748–1755.
- Oga T, Nishimura K, Tsukino M, et al. Analysis of the factors related to mortality in chronic obstructive pulmonary disease: role of exercise capacity and health status. Am J Respir Crit Care Med. 2003 Feb 15;167(4):544–549.
- Global Initiative for Chronic Obstructive Lung Disease. Global strategy for the diagnosis, management, and prevention of chronic obstructive pulmonary disease (2020 report). Fontana: Global Initiative for chronic obstructive lung disease; 2020. cited 2020 28 Apr]. Available from: https://goldcopd.org/wp-content/uploads/2019/12/GOLD-2020-FINAL-ver1.2-03Dec19_WMV.pdf
- Spruit MA, Singh SJ, Garvey C, et al. An official American Thoracic Society/European Respiratory Society statement: key concepts and advances in pulmonary rehabilitation. Am J Respir Crit Care Med. 2013 Oct 15;188(8):e13–64.
- National Institute for Health and Care Exellence. Chronic obstructive pulmonary disease in over 16s: diagnosis and management [NG 115]. London: National Institute for Health and Care Excellence; 2019. [cited 2020 May 5] Available from https://www.nice.org.uk/guidance/ng115.
- Bolton CE, Bevan-Smith EF, Blakey JD, et al. British Thoracic Society guideline on pulmonary rehabilitation in adults. Thorax. 2013 Sep;68(Suppl 2):ii1–30.
- Hardinge M, Annandale J, Bourne S, et al. British Thoracic Society guidelines for home oxygen use in adults. Thorax. 2015 Jun;70(Suppl 1):i1–43.
- Wedzicha JA. Domiciliary oxygen therapy services: clinical guidelines and advice for prescribers. Summary of a report of the Royal College of Physicians. J R Coll Physicians Lond. 1999 Sep-Oct;33(5):445–447.
- Borg GAV. Psychophysical bases of perceived exertion. Med Sci Sports Exercise. 1982;14(5):377–381.
- Miller MR, Hankinson J, Brusasco V, et al. Standardisation of spirometry. Eur Respir J. 2005 Aug;26(2):319–338.
- Graham BL, Brusasco V, Burgos F, et al. ERS/ATS standards for single-breath carbon monoxide uptake in the lung. Eur Respir J. 2017;49:1.
- Holland AE, Spruit MA, Troosters T, et al. An official European Respiratory Society/American Thoracic Society technical standard: field walking tests in chronic respiratory disease. Eur Respir J. 2014 Dec;44(6):1428–1446.
- Lang RM, Badano LP, Mor-Avi V, et al. Recommendations for cardiac chamber quantification by echocardiography in adults: an update from the American Society of Echocardiography and the European Association of Cardiovascular Imaging. J Am Soc Echocardiogr. 2015 Jan;28(1):1–39.e14.
- Leren IS, Saberniak J, Haland TF, et al. Combination of ECG and Echocardiography for Identification of Arrhythmic Events in Early ARVC. JACC Cardiovasc Imaging. 2017 May;10(5):503–513.
- Jones PW, Harding G, Berry P, et al. Development and first validation of the COPD Assessment Test. Eur Respir J. 2009 Sep;34(3):648–654.
- Meguro M, Barley EA, Spencer S, et al. Development and validation of an improved, COPD-specific version of the St. George Respiratory Questionnaire. Chest. 2007 Aug;132(2):456–463.
- Scharf SM, Iqbal M, Keller C, et al. Hemodynamic characterization of patients with severe emphysema. Am J Respir Crit Care Med. 2002 Aug 1;166(3):314–322.
- Thabut G, Dauriat G, Stern JB, et al. Pulmonary hemodynamics in advanced COPD candidates for lung volume reduction surgery or lung transplantation. Chest. 2005 May;127(5):1531–1536.
- Galiè N, Humbert M, Vachiery JL, et al. 2015 ESC/ERS Guidelines for the diagnosis and treatment of pulmonary hypertension: the Joint Task Force for the Diagnosis and Treatment of Pulmonary Hypertension of the European Society of Cardiology (ESC) and the European Respiratory Society (ERS): endorsed by: association for European Paediatric and Congenital Cardiology (AEPC), International Society for Heart and Lung Transplantation (ISHLT). Eur Heart J. 2016 Jan 1;37(1):67–119.
- Simonneau G, Montani D, Celermajer DS, et al. Haemodynamic definitions and updated clinical classification of pulmonary hypertension. Eur Respir J. 2019;53:1.
- Ward TJC, Plumptre CD, Dolmage TE, et al. Change in V˙O(2peak) in response to aerobic exercise training and the relationship with exercise prescription in people with COPD: a systematic review and meta-analysis. Chest. 2020 Jul;158(1):131–144.
- Poole DC, Jones AM. Measurement of the maximum oxygen uptake Vo(2max): vo(2peak) is no longer acceptable. J Appl Physiol (1985). 2017 Apr 1;122(4):997–1002.
- Pulz C, Diniz RV, Alves AN, et al. Incremental shuttle and six-minute walking tests in the assessment of functional capacity in chronic heart failure. Can J Cardiol. 2008 Feb;24(2):131–135.
- Sims MW, Margolis DJ, Localio AR, et al. Impact of pulmonary artery pressure on exercise function in severe COPD. Chest. 2009 Aug;136(2):412–419.
- Ehlken N, Lichtblau M, Klose H, et al. Exercise training improves peak oxygen consumption and haemodynamics in patients with severe pulmonary arterial hypertension and inoperable chronic thrombo-embolic pulmonary hypertension: a prospective, randomized, controlled trial. Eur Heart J. 2016 Jan 1;37(1):35–44.
- Beauchamp MK, Nonoyama M, Goldstein RS, et al. Interval versus continuous training in individuals with chronic obstructive pulmonary disease–a systematic review. Thorax. 2010 Feb;65(2):157–164.
- Gloeckl R, Marinov B, Pitta F. Practical recommendations for exercise training in patients with COPD. Eur Respir Rev. 2013 Jun 1;22(128):178–186.
- Wisløff U, Støylen A, Loennechen JP, et al. Superior cardiovascular effect of aerobic interval training versus moderate continuous training in heart failure patients: a randomized study. Circulation. 2007 Jun 19;115(24):3086–3094.
- Brønstad E, Tjonna AE, Rognmo Ø, et al. Aerobic exercise training improves right- and left ventricular systolic function in patients with COPD. Copd. 2013 Jun;10(3):300–306.
- Arcasoy SM, Christie JD, Ferrari VA, et al. Echocardiographic assessment of pulmonary hypertension in patients with advanced lung disease. Am J Respir Crit Care Med. 2003 Mar 1;167(5):735–740.
- Sala E, Roca J, Marrades RM, et al. Effects of endurance training on skeletal muscle bioenergetics in chronic obstructive pulmonary disease. Am J Respir Crit Care Med. 1999 Jun;159(6):1726–1734.
- Maltais F, LeBlanc P, Simard C, et al. Skeletal muscle adaptation to endurance training in patients with chronic obstructive pulmonary disease. Am J Respir Crit Care Med. 1996 Aug;154(2 Pt 1):442–447.
- Whittom F, Jobin J, Simard PM, et al. Histochemical and morphological characteristics of the vastus lateralis muscle in patients with chronic obstructive pulmonary disease. Med Sci Sports Exerc. 1998 Oct;30(10):1467–1474.
- Bowen TS, Aakerøy L, Eisenkolb S, et al. Exercise Training Reverses Extrapulmonary Impairments in Smoke-exposed Mice. Med Sci Sports Exerc. 2017 May;49(5):879–887.
- Polverino F, Laucho-Contreras ME, Petersen H, et al. A pilot study linking endothelial injury in lungs and kidneys in chronic obstructive pulmonary disease. Am J Respir Crit Care Med. 2017 Jun 1;195(11):1464–1476.
Appendix
Table A1. Supplementary baseline characteristics of the 16 included patients