ABSTRACT
Introduction
Thoracic ultrasound (TUS) has proven useful in the diagnosis, risk stratification and monitoring of disease progression in patients with coronavirus disease 2019 (COVID-19). However, utility in follow-up is poorly described. To elucidate this area, we performed TUS as part of a 12-month clinical follow-up in patients previously admitted with COVID-19 and correlated findings with clinical assessment and pulmonary function tests.
Methods
Adult patients discharged from our hospital following admission with COVID-19 during March to May 2020 were invited to a 12-month follow-up. Enrolled patients were interviewed regarding persisting or newly developed symptoms in addition to TUS, spirometry and a 6-min walk test. Patients were referred to high-resolution computed tomography (HRCT) of the lungs if suspicion of pulmonary fibrosis was raised.
Results
Forty patients were enrolled in the study of whom had 13 developed acute respiratory distress syndrome (ARDS) during admission. Patients with ARDS were more prone to experience neurological symptoms at follow-up (p = 0.03) and showed more B-lines on TUS (p = 0.008) but did not otherwise differ significantly in terms of pulmonary function tests. Four patients had pathological findings on TUS where subsequent diagnostics revealed that two had interstitial lung abnormalities and two had heart failure. These four patients presented with a significantly lower diffusing capacity of lung for carbon monoxide (p=0.03) and 6-min walking distance (p=0.006) compared to the remaining 36 patients without ultrasound pathology. No significant difference was observed in spirometry values of % of predicted FEV1 (p=0.49) or FVC (p=0.07). No persisting cardiovascular pathology was observed in patients without ultrasonographic pathology.
Conclusion
At 12-month after admission with COVID-19, a follow-up combining TUS, clinical assessment, and pulmonary function tests may improve the selection of patients requiring further diagnostic investigations such as HRCT or echocardiography.
Introduction
Severe acute respiratory syndrome coronavirus 2 (SARS-CoV-2) is highly contagious and often affects the respiratory organs, causing symptoms of varying severity, ranging from simple cough to severe pneumonia or even acute respiratory distress syndrome (ARDS) requiring intensive care treatment [Citation1]. Although the extensive rollout of SARS-CoV-2 vaccines and the emergence of new SARS-CoV-2 variants have resulted in reduced risk of severe disease progression, there are still patients who deteriorate rapidly from limited symptoms to acute respiratory failure with ARDS [Citation2]. Close monitoring is therefore still essential for early detection of clinical aggravation, ideally with access to rapid and repeated thoracic imaging. However, intrahospital transportation to chest computed tomography (CT) or non-supine chest X-ray is both time-consuming and increases risk of virus transmission. As such, thoracic ultrasound (TUS), a thoracic imaging modality with high diagnostic accuracy within a variety of respiratory conditions, quickly gained the attention of researchers [Citation1,Citation3]. TUS excels as a mobile, fast, and cost-efficient imaging approach ideal for frequent repeated imaging, which is able to readily detect dynamic changes in lung parenchyma and pleura as observed in relation to pulmonary edema or ARDS [Citation4,Citation5]. Several recent studies have evaluated the utility of TUS for diagnosis, risk stratification, and monitoring of disease progression in patients with coronavirus disease 2019 (COVID-19) [Citation6–12].
As TUS is also able to detect chronic interstitial and pleural affection, visualized as the so-called interstitial syndrome or pleural irregularity which correspond to ground glass opacities or pulmonary fibrosis on a high-resolution CT (HRCT), TUS might also play a role in the clinical follow-up of patients with COVID-19 [Citation13–15]. Indeed, recent comprehensive meta-analytic data, predating the omicron variant, of patients admitted with COVID-19 found that approximately half of COVID-19 survivors retain pulmonary abnormalities on chest CT or develop some degree of pulmonary fibrosis with a predominance in patients with severe course of COVID-19 [Citation16,Citation17]. As such, it is feasible that addition of TUS to the pulmonary physiology follow-up strategy recommended by the European Respiratory Society may improve selection of patients requiring referral to chest HRCT [Citation18].
To elucidate the potential of TUS in this setting, the findings from TUS performed as part of a 12-month clinical follow-up of patients admitted with COVID-19 were correlated with prevalence of persisting symptoms, pulmonary function tests, and available chest imaging, and stratified by ARDS during admission to assess if this patient group was more prone to exhibit long-term TUS changes.
Methods
Setting and patient population
This study was conducted at South Danish Center for Interstitial Lung Diseases, an outpatient clinic under the Department of Respiratory Medicine at Odense University Hospital (OUH) in Denmark, which acts as a tertiary referral center for interstitial lung diseases (ILDs) for inhabitants of the Region of Southern Denmark (RSD) with a population of 1.22 million [Citation19]. In the beginning of the epidemic, all patients requiring hospital admission for COVID-19 in the RSD, who were clinically stable for transportation, were transferred to a dedicated COVID-19 unit at OUH for further treatment. All patients who were ≥18 years and had a positive real-time polymerase chain reaction analysis for SARS-CoV-2 (i.e. tracheal secretion or pharyngeal swab) were included in a study cohort previously described by Madsen et al. [Citation20]. Diagnosis of ARDS during admission was established using the Berlin criteria [Citation21]. In this study, we invited all patients from the cohort discharged in the period of March–May 2020 to a 12-month follow-up appointment.
Twelve-month clinical follow-up
Patients who accepted our invitation were subject to a comprehensive interview regarding persisting or newly developed symptoms in addition to TUS, and pulmonary function test. If suspicion of interstitial affection was raised based on the investigations, the patient was referred to HRCT.
Pulmonary function tests
Spirometry including forced expiratory volume in 1 sec (FEV1) and forced expiratory capacity (FVC), diffusion capacity of the lung for carbon monoxide adjusted for hemoglobin (DLCOc) and 6-min walk test (6MWT) including 6-min walk distance (6MWD) were performed, whenever it was possible for the patient to complete the given test. All tests were performed in accordance with internationally accepted standards [Citation22–24].
Thoracic ultrasound
The TUS examinations were conducted using a LOGIQ E9 (General Electric, Boston, Massachusetts, USA) with a 1–6 MHz curved array probe. All investigations were done by one of four respiratory physicians certified in TUS by the regional center for healthcare education with more than 200 previous TUS examinations each. The investigators were blinded to the medical history of the participants. We used a 14-zone protocol validated for assessment of patients with respiratory symptoms and diseases in different settings often utilized for detection of pneumothorax, pleural effusion, interstitial syndrome, and subpleural consolidations [Citation25–27]. The protocol was supplemented with the Mongodi scoring system, which is a validated tool for assessing loss of aeration in anterior and lateral zones of patients with severe pneumonia and ARDS [Citation28]. In the present study, we supplemented the protocol with the six posterior zones as all patients were able to sit upright at follow-up.
Between 1 and 4 points are given in each zone based on TUS findings, resulting in a total score between 8 and 32 points for the anterolateral Mongodi approach and between 14 and 56 points for the modified 14-zone Mongodi approach.
Each point was defined according to the following ():
Figure 1. TUS findings and corresponding score. a) The pleural line (PL) appears normal. No B-lines or consolidations are present corresponding to a score of 1. b) Multiple B-lines (B) originating from the pleural line. The B-lines involve less than 50% of the pleural line, corresponding to a score of 2. c) Multiple confluent B-lines (CB) are present. More than 50% of the pleural line is involved corresponding to a score of 3. d) A small subpleural consolidation (C) is present just below the pleural line, corresponding to a score of 4. Image courtesy of Falster et al. [Citation11].
![Figure 1. TUS findings and corresponding score. a) The pleural line (PL) appears normal. No B-lines or consolidations are present corresponding to a score of 1. b) Multiple B-lines (B) originating from the pleural line. The B-lines involve less than 50% of the pleural line, corresponding to a score of 2. c) Multiple confluent B-lines (CB) are present. More than 50% of the pleural line is involved corresponding to a score of 3. d) A small subpleural consolidation (C) is present just below the pleural line, corresponding to a score of 4. Image courtesy of Falster et al. [Citation11].](/cms/asset/b9cb8093-0068-4362-bcab-a11e4603da1a/zecr_a_2257992_f0001_b.gif)
−1 point: <3 B-lines
−2 points: ≥3 B-lines occupying ≤ 50% of the pleural line
−3 points: ≥3 B-lines occupying > 50% of the pleural line
−4 points: Lung consolidation present.
Any TUS examinations with at least one zone showing ≥ 3 B-lines, pleural effusion, subpleural consolidation or a pneumothorax was considered abnormal.
Indications of pulmonary sequelae
On the basis of all assessments performed at the follow-up appointment (with the exception of TUS), patients were stratified into six groups defined on the basis of presence of perceived or objectively demonstrated pulmonary sequelae. These comprised:
Interstitial lung sequelae (results suggestive of interstitial lung affection) supported by radiology with or without restrictive lung function)
Chronic obstructive pulmonary disease/emphysematous sequelae (obstructive lung function pattern with or without radiology findings suggestive of airway disease)
Asthmatic sequelae (clinical symptoms and pulmonary physiology indicating variable airway obstruction or inflammation)
Combination of the above groups
Patients experiencing chronic airway symptoms such as dyspnea and/or cough, but pulmonary function tests or radiology were incompatible with any specific disease
Other.
Patients were referred for HRCT if the findings at follow-up were compatible with interstitial lung sequelae due to interstitial lung affection. However, HRCT was not mandatory for allocation to this group if previous chest x-ray or conventional chest CT had shown findings of interstitial lung involvement.
Statistical analysis
All statistical analyses were performed in GraphPad Prism 9.0.0. (GraphPad Software, San Diego, California USA). Normality was assessed using the Shapiro–Wilk test. Normally distributed data is presented as mean ± standard deviation (SD) and compared with the Student’s t-test. Non-normally distributed data is presented as median with interquartile range (IQR) and compared with a Mann–Whitney U-test. χ2-test or Fisher’s exact test was used for comparing categorical data. Yates correction was applied to the χ2-test when at least one table of a cell had a count smaller than five. P-values <0.05 were considered statistically significant. Pearson correlation coefficient was used to assess correlation between continuous variables. Interpretation of correlation coefficients was conducted in accordance with the work of Schober and colleagues [Citation29].
Results
Baseline characteristics
Upon invitation of 92 patients, 11 declined, 31 did not respond, and 10 had died, resulting in the inclusion of 40 patients with a mean of 393 ± 18 days between discharge and follow-up. The baseline characteristics of the included patients and the 52 patients who did not attend follow-up were obtained from the Madsen cohort and were generally comparable () [Citation20]. Further, distance to the hospital was similar between groups. Sixty-five percent of the study population were male with a mean age of 60.4 ± 13.6 years, and a median BMI of 27.9 (IQR: 25.1–31.3).
Table 1. Baseline characteristics of patients stratified by follow-up attendance.
Thirteen out of 40 patients developed ARDS during their hospital stay and had a median length of admission of 15 days (IQR: 9–31), which was significantly longer than the 4 (IQR: 3–8) days in the 27 non-ARDS patients (p < 0.001) (). Further, ARDS-patients were more prone to receive supplemental oxygen at time of admission (p = 0.01), were more likely to be transferred to an intensive care unit (ICU) (p < 0.001) and had a higher BMI (p = 0.047) but did not otherwise differ significantly from the remaining study participants.
Table 2. Baseline characteristics of included patients stratified by ARDS during admission.
Clinical follow-up
The most common complaints at 12-month follow-up were cerebral symptoms (45%) (encompassing sensation of fatigue, irritability, depressive symptoms, increased need for sleep, or anosmia), weight gain (38%), and perceived dyspnea (25%) (). While cerebral complaints were more common in patients with previous ARDS (69%) than in those without (33%) (p = 0.033), no difference in prevalence of other complaints were observed between groups. Spirometry results were overall within normal ranges, revealing a mean FEV1 of 101 ± 18% of predicted with no difference between groups (p = 0.32). FVC was 108 ± 18% of predicted and FEV1/FVC was 75.9 ± 18. Likewise, no difference was observed between groups with and without ARDS during admission, (p = 0.43) and (p = 0.42) for FVC and FEV1/FVC, respectively. Mean DLCOc at 85 ± 17% of predicted did also not differ between groups (p = 0.36). Mean 6MWD at follow-up was 518 ± 105 meters and saturation post-6MWT-exercise was 96% (IQR: 94–97%). We found a non-statistically significant shorter mean 6MWD for ARDS-patients (479 ± 93 meters) compared to non-ARDS-patients (538 ± 107 meters; (p = 0.05)).
Table 3. Outcomes of included patients stratified by ARDS during admission.
Based on findings during follow-up, eight patients (20%) fulfilled the criteria for any pulmonary sequelae. Four patients had sequelae not compatible with any specific pulmonary disease, three had asthmatic sequelae, and one had findings compatible with interstitial lung sequelae.
TUS findings
A full 14-zone ultrasound examination was feasible in all patients. Ultrasound examinations were unremarkable in the majority of patients, yielding median Mongodi scores at 8 (IQR: 8–8) using the standard anterolateral approach and 14 (IQR: 14–14) using the extended protocol. No statistically significant difference was observed when stratifying for presence of ARDS (p = 0.08) (). The prevalence of B-lines was overall limited but significantly lower in non-ARDS-patients (0 (IQR: 0–0)) than in ARDS-patients (0 (IQR: 0–6); p = 0.008)). We found an overall low prevalence of subpleural consolidations (10%) and pleural effusion (3%) with no difference between ARDS-groups (p = 0.33 and p = 0.43, respectively). In total, only four patients (10%) showed abnormal ultrasonographic findings which are elaborated and presented with other clinical data in .
Figure 2. Comparison of pulmonary function test results between patients with and without abnormal thoracic ultrasound findings.FEV1: Forced expiratory volume in 1 second. FVC: Forced vital capacity. DLCOc: diffusion capacity of the lung for carbon monoxide adjusted for hemoglobin. 6MWT: 6-minute walking distance.
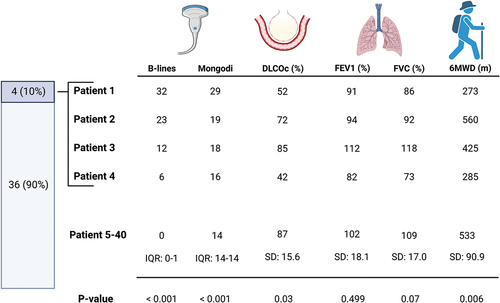
Correlation between TUS findings and other clinical parameters
The four patients with abnormal TUS investigation had significantly lower DLCOc (p = 0.03) and 6MWD (p = 0.006) than the remaining 36 patients without ultrasound pathology (). However, when analyzing all 40 patients, we observed no strong correlation between the total number of B-lines or Mongodi score and any clinical parameters at 12-month follow-up (). A moderate negative correlation was observed between Mongodi score and 6MWD (r = −0.422). Correlations between Mongodi score and DLCOc (r = −0.364) as well as between Mongodi score and Borg scale post-6MWT exercise (r = 0.368) were both weak. This also applied to correlations between total number of B-lines and 6-MWD (r = −0.360) and between total number of B-lines and DLCOc (r = −0.385), respectively. All correlations between TUS and spirometry findings were weak or negligible.
Table 4. Correlations between thoracic ultrasound findings and pulmonary function tests.
Among the eight patients fulfilling criteria of pulmonary sequelae, two had abnormal TUS findings: one patient had sequelae compatible with interstitial lung abnormalities which was confirmed by a subsequent HRCT (). The other had unspecific sequelae and a subsequent HRCT revealed no interstitial abnormalities but indicated pulmonary hypertension which was subsequently confirmed by echocardiography showing left ventricular heart failure with group 2 pulmonary hypertension. Neither of the remaining two patients with abnormal TUS fulfilled the criteria for pulmonary sequelae and their HRCTs showed stable interstitial lung abnormalities and signs of pulmonary hypertension in relation to known heart failure when compared to prior HRCT descriptions. All patients with asthmatic sequelae had normal TUS findings.
Discussion
While subjective complaints were common at 12 months COVID-19 follow-up, these were seldom of respiratory nature and objective signs of persisting pulmonary impairment were rarely observed in our cohort. Indeed, only eight patients (20%) fulfilled our pre-defined criteria of pulmonary sequelae. Of the 40 patients attending follow-up, only four (10%) had Mongodi scores above baseline 14 points, in whom spirometry values were generally within normal range but with decreased DLCOc and 6MWD. As such, though uncommon, persisting pathology on TUS one year following admission with COVID-19 may indicate interstitial lung affection or other unacknowledged cardiopulmonary pathology, such as heart failure.
The continued presence of subjective symptoms such as fatigue or breathlessness following COVID-19 infection is well described, but the duration and natural course of this long-COVID syndrome is, however, still poorly understood [Citation30,Citation31]. A recent meta-analysis describing 8,591 COVID-19 survivors reported that persistent symptoms are still common one year after discharge, for instance dyspnea or fatigue which were still experienced by 18% and 28% of patients, respectively [Citation32]. While our study pooled cerebral complaints, the prevalence of symptoms at follow-up is comparable to the findings of previous studies, suggesting a representative study population.
While the meta-analysis only reported on subjective symptoms at follow-up, other studies have described ultrasonographic findings in the period after COVID-19 hospital admission. For instance, a recent study by Espersen and colleagues supports our findings that most patients experience complete TUS resolution following discharge [Citation33]. In this study, 71 patients who had been subject to an 8-zone TUS examination of anterior and lateral zones during COVID-19-admission were re-examined at 2–3 months following discharge. Only 30% of these patients had pathological TUS findings (i.e. ≥3 B-lines in at least one zone) at time of follow-up. The authors note that these findings were more prevalent in patients who had developed ARDS during admission, which is consistent with our observations, where the three patients with the highest Mongodi scores were also diagnosed with ARDS and required ICU admission.
In our study, we only observed ≥ 3 B-lines in at least one zone in 10% of the included patients, compared to 30% at 2–3 months follow-up in the Espersen study. Although it should be kept in mind that our study has no available baseline TUS values from time of admission, limiting comparability, this could imply that complete pulmonary resolution following COVID-19 may be protracted beyond 3 months in some patients due to reversible virus-induced inflammation presenting with ground glass opacities on HRCT, or that other pathology compatible with multiple B-lines is present, for instance heart failure or consisting interstitial lung abnormalities. Indeed, of the four patients with abnormal TUS at 12-month follow-up, one exhibited HRCT findings consistent with persisting interstitial lung changes following severe COVID-19 infection, one exhibited interstitial lung abnormalities which had been acknowledged years prior to the admission with COVID-19 [Citation34] and the remaining two had left ventricular heart failure.
As such, it is plausible that remaining TUS changes 12 months following COVID-19 discharge should raise suspicion of significant pathology other than ongoing resolution of COVID-19 pneumonia. This notion is supported by the works of Giovanetti and colleagues, reporting that persisting B-lines or subpleural consolidations at 3-month follow-up were correlated with CT-findings compatible with interstitial lung abnormalities in 80% [Citation35]. Furthermore, two-thirds of these CT-findings, encompassing ground-glass opacities, subpleural bands and interstitial thickening, were described as mild and no significant difference in % of predicted FEV1/FEV, DLCO, or 6MWD was observed between patients with and without interstitial lung abnormalities. Hence, TUS findings compatible with interstitial lung affection may be anticipated in the first months following COVID-19 pneumonia and should not necessarily lead to a confirmative HRCT, especially not if other pulmonary function tests are within normal ranges. In contrast, in the few patients retaining abnormal TUS observations beyond 12 months, further diagnostic work-up may be warranted. Naturally, if the patient is already known to have ILD, heart failure or other conditions compatible with TUS findings, an individual approach should be taken.
Our findings suggest a high specificity of TUS for pulmonary sequelae following COVID-19 as the investigated 36 patients with unremarkable TUS findings had mean values of FEV1, FVC, DLCOc, and 6MWD within normal ranges. However, it should be kept in mind that asthmatic or emphysematous sequelae are not detectable by TUS which is only able to visualize pleural and parenchymal changes. This was exemplified in our study, where none of the three patients with asthmatic sequelae had abnormal TUS findings.
Lastly, it should be mentioned that prior to clinical application of TUS, physicians should complete a thorough theoretical and practical training programme with subsequent certification to minimize the risk of misinterpreting findings [Citation36,Citation37].
Limitations
Our findings suggest TUS as a valuable tool in distinguishing between presence and absence of certain lung parenchymal, pleural, and cardiovascular conditions 12 months following COVID-19, such as interstitial lung abnormalities and heart failure; however, important limitations should be considered when interpreting our results.
Mainly, as no attempts were made to adjust for test multiplicity in terms of P-values or for confounders, it is conceivable that the significant differences observed between patients who did and did not participate, patients with and without ARDS, and TUS groups, have arisen purely by chance although consistent with previous research. Further, while patient characteristics of the 40 participants were generally similar to the 52 who declined study participation or were not able to attend follow-up, especially the low performance status of our cohort from the first COVID-19 wave (i.e. spring 2020) may not be representative of the contemporary patient admitted with COVID-19, where the change in SARS-CoV-2 strain and the global vaccination effort have shifted admissions towards primarily the elderly or co-morbid population. Also, not all patients were subject to an HRCT, prohibiting sufficient calculation of sensitivity and specificity for TUS in confirming or rejecting suspicion pulmonary interstitial conditions. However, as the patients in our study without abnormal TUS findings exhibited normal lung physiology, TUS may be an important bedside add-on modality to clinical decision-making, warranting further investigations in dedicated diagnostic accuracy studies.
Conclusion
The prevalence of abnormal TUS findings 12 months following admission with COVID-19 is low. Detection of TUS findings indicating lung parenchymal, pleural, or cardiovascular conditions at 12-month COVID-19 follow-up which are not explained by already known ailments should prompt further diagnostic work-up such as HRCT or echocardiography.
Take home message
Thoracic ultrasonographic abnormalities are uncommon 12 months following admission due to COVID-19. If present, findings compatible with pulmonary or cardiovascular sequelae should prompt further diagnostic work-up such as HRCT or echocardiography.
Disclosure statement
No potential conflict of interest was reported by the author(s).
Additional information
Funding
References
- Huang C, Wang Y, Li X, et al. Clinical features of patients infected with 2019 novel coronavirus in Wuhan, China. Lancet. 2020;395(10223):497–10. Lancet Publishing Group. doi: 10.1016/S0140-6736(20)30183-5.
- Bouzid D, Visseaux B, Kassasseya C, et al. Comparison of patients infected with delta versus omicron COVID-19 variants presenting to Paris emergency departments: a retrospective cohort study. Ann Intern Med [Internet]. 2022 [cited 2023 Jan 30];175(6):831–837. PMC8941485/. doi: 10.7326/M22-0308
- Knight PH, Maheshwari N, Hussain J, et al. Complications during intrahospital transport of critically ill patients: focus on risk identification and prevention. Int J Crit Illn Inj Sci Wolters Kluwer Medknow Publications. 2015;5(4):256–264. doi: 10.4103/2229-5151.170840
- Arbelot C, Ferrari F, Bouhemad B, et al. Lung ultrasound in acute respiratory distress syndrome and acute lung injury. Curr Opin Crit Care Curr Opin Crit Care. 2008;14(1):70–74. doi: 10.1097/MCC.0b013e3282f43d05
- Frasure SE, Matilsky DK, Siadecki SD, et al. Impact of patient positioning on lung ultrasound findings in acute heart failure. Eur Heart J Acute Cardiovasc Care. 2015;4(4):326–332. doi: 10.1177/2048872614551505
- Zhou Y, Fan Q, Cavus O, et al. Lung ultrasound: predictor of acute respiratory distress syndrome in intensive care unit patients. Saudi J Anaesth Wolters Kluwer Medknow Publications. 2018;12(3):457–461. doi: 10.4103/sja.SJA_73_18
- Bonadia N, Carnicelli A, Piano A, et al. Lung ultrasound findings are associated with mortality and need for intensive care admission in COVID-19 patients evaluated in the emergency department. Ultrasound Med Biol. 2020;46(11):2927–2937. doi: 10.1016/j.ultrasmedbio.2020.07.005
- Brahier T, Meuwly J-Y, Pantet O, et al. Lung ultrasonography for risk stratification in patients with coronavirus disease 2019 (COVID-19): a prospective observational cohort study. Clinl Infect Dis Oxford Academic. 2021;73(11):e4189–e4196. doi: 10.1093/cid/ciaa1408
- Pare JR, Camelo I, Mayo KC, et al. Point-of-care lung ultrasound is more sensitive than chest radiograph for evaluation of COVID-19. West J Emerg Med. 2020;21(4):771. doi: 10.5811/westjem.2020.5.47743
- Hussain A, Via G, Melniker L, et al. Multi-organ point-of-care ultrasound for COVID-19 (PoCUS4COVID): international expert consensus. Crit Care. 2020;24(1):24. doi: 10.1186/s13054-020-03369-5
- Falster C, Jacobsen N, Wulff Madsen L, et al. Lung ultrasound may be a valuable aid in decision making for patients admitted with COVID-19 disease. Eur Clin Respir J. 2021;8(1): Taylor and Francis Ltd. doi: 10.1080/20018525.2021.1909521
- Ebrahimzadeh S, Islam N, Dawit H, et al. Thoracic imaging tests for the diagnosis of COVID-19. Cochrane Database Syst Rev InternetCochrane Database Syst Rev. 2022 [cited 2022 Dec 14];2022(6): doi: 10.1002/14651858.CD013639.pub5
- Volpicelli G, Mussa A, Garofalo G, et al. Bedside lung ultrasound in the assessment of alveolar-interstitial syndrome. Am J Emerg Med. 2006;24(6):689–696. doi: 10.1016/j.ajem.2006.02.013
- Laursen CB, Rahman NM, Volpicelli G. Thoracic ultrasound. Thoracic Ultrasound European Respiratory Society. 2018. doi: 10.1183/2312508X.erm7918
- Davidsen JR, Laursen CB, Højlund M, et al. Lung ultrasound to phenotype chronic lung allograft dysfunction in lung transplant recipients. A prospective observational study. J Clin Med. [Internet]. 2021 [cited 2023 Jan 14];10(5):1–14. doi: 10.3390/jcm10051078
- Hama Amin BJ, Kakamad FH, Ahmed GS, et al. Post COVID-19 pulmonary fibrosis; a meta-analysis study. Ann Med Surg. 2022;77:103590. doi: 10.1016/j.amsu.2022.103590
- Fabbri L, Moss S, Khan FA, et al. Parenchymal lung abnormalities following hospitalisation for COVID-19 and viral pneumonitis: a systematic review and meta-analysis. Thorax. [Internet]. 2023 [cited 2023 Jan 15];78(2):191–201. doi: 10.1136/thoraxjnl-2021-218275
- Antoniou KM, Vasarmidi E, Russell AM, et al. European respiratory Society statement on long COVID follow-up. Eur Respir J. Internet Eur Respir J . 2022 [cited 2022 Dec 1];60(2):2102174. doi: 10.1183/13993003.02174-2021
- Befolkningstal - Danmarks Statistik [Internet]. [cited 2022 Sep 1]. Available from: https://www.dst.dk/da/Statistik/emner/borgere/befolkning/befolkningstal.
- Madsen LW, Lindvig SO, Rasmussen LD, et al. Low mortality of hospitalised patients with COVID-19 in a tertiary Danish hospital setting. Int J Infect Dis. 2021;102:212–219. doi: 10.1016/j.ijid.2020.10.018
- Ranieri VM, Rubenfeld GD, Thompson BT, et al. Acute respiratory distress syndrome: the Berlin definition. JAMA. 2012;307(23):2526–2533. doi: 10.1001/jama.2012.5669
- Stanojevic S, Kaminsky DA, Miller MR, et al. ERS/ATS technical standard on interpretive strategies for routine lung function tests. Eur Respir J. [Internet]. 2022 [cited 2022 Dec 1];60(1):2101499. doi: 10.1183/13993003.01499-2021
- Graham BL, Brusasco V, Burgos F, et al. 2017 ERS/ATS standards for single-breath carbon monoxide uptake in the lung. Eur Respir J. [Internet]. 2017 [cited 2022 Dec 1];49(1):1600016. doi: 10.1183/13993003.00016-2016
- Graham BL, Steenbruggen I, Barjaktarevic IZ, et al. Standardization of spirometry 2019 update. An official American Thoracic Society and European respiratory Society technical statement. Am J Respir Crit Care Med. [Internet]. 2019 [cited 2022 Dec 1];200(8):E70–E88. doi: 10.1164/rccm.201908-1590ST
- Laursen CB, Sloth E, Lassen AT, et al. Point-of-care ultrasonography in patients admitted with respiratory symptoms: a single-blind, randomised controlled trial. Lancet Respir Med. [Internet]. 2014 [cited 2020 Dec 26];2(8):638–646. doi: 10.1016/S2213-2600(14)70135-3
- Laursen CB, Clive A, Hallifax R, et al. European respiratory Society statement on thoracic ultrasound. Eur Respir J. 2021 [cited 2022 Jul 8];57(3):2001519. doi: 10.1183/13993003.01519-2020
- Casper Falster A, Egholm G, Wiig R, et al. Diagnostic accuracy of a Bespoke Multiorgan ultrasound approach in suspected pulmonary embolism. Ultrasound Int Open. [Internet]. Georg Thieme Verlag KG . 2022 [cited 2023 Jan 30];8(02):E59–E67. doi: 10.1055/a-1971-7454
- Mongodi S, Bouhemad B, Orlando A, et al. Modified lung ultrasound score for assessing and monitoring pulmonary aeration. Ultraschall Med. 2017;38(5):530–537. doi: 10.1055/s-0042-120260
- Schober P, Schwarte LA. Correlation coefficients: appropriate use and interpretation. Anesth Analg. [Internet]. 2018 [cited 2022 Dec 14];126(5):1763–1768. doi: 10.1213/ANE.0000000000002864
- Raveendran AV, Jayadevan R, Sashidharan S. Long COVID: an overview. Diabetes Metab Syndr. [Internet]. 2021 [cited 2022 Dec 1];15(3):869–875. doi: 10.1016/j.dsx.2021.04.007
- Crook H, Raza S, Nowell J, et al. Long covid—mechanisms, risk factors, and management. BMJ. [Internet]. 2021 [cited 2022 Dec 1];374:n1648. doi: 10.1136/bmj.n1648
- Han Q, Zheng B, Daines L, et al. Long-term sequelae of COVID-19: a systematic review and meta-analysis of one-year follow-up studies on post-COVID symptoms. Pathogens. [Internet]. 2022 [cited 2022 Dec 1];11(2):269. doi: 10.3390/pathogens11020269
- Espersen C, Platz E, Alhakak AS, et al. Lung ultrasound findings following COVID-19 hospitalization: a prospective longitudinal cohort study. Respir med. [Internet]. 2022 [cited 2022 Dec 1];197:106826. doi: 10.1016/j.rmed.2022.106826.
- Hatabu H, Hunninghake GM, Richeldi L, et al. Interstitial lung abnormalities detected incidentally on CT: a position paper from the Fleischner Society. Lancet Respir Med. [Internet]. 2020 [cited 2023 Jan 30];8(7):726–737. doi: 10.1016/S2213-2600(20)30168-5
- Giovannetti G, de Michele L, de Ceglie M, et al. Lung ultrasonography for long-term follow-up of COVID-19 survivors compared to chest CT scan. Respir med. [Internet]. 2021 [cited 2022 Dec 14];181:106384. doi: 10.1016/j.rmed.2021.106384
- Pietersen PI, Madsen KR, Graumann O, et al. Lung ultrasound training: a systematic review of published literature in clinical lung ultrasound training. Crit Ultrasound J. [Internet]. 2018 [[cited 2022 Aug 5]];10(1).https://pubmed.ncbi.nlm.nih.gov/30175392/
- The thoracic ultrasound certified training programme - ERS - European respiratory Society [Internet]. [cited 2023 Apr 4]. Avaiable from: https://www.ersnet.org/education-and-professional-development/ers-certified-training-programmes/thoracic-ultrasound-certified-training-programme/