Abstract
Knowledge on the fracture properties of materials is essential to assure structural integrity and proper design of mechanical connections in timber constructions. Measurement of this property is, however, a very challenging task. The linear fracture mechanics is usually used for its assessment assisted with experimental data acquired by means of various techniques, usually of destructive nature. The cutting force is an energetic effect of splitting material, and might be therefore considered from a point of view of modern fracture mechanics. The original methodology of simultaneous determination of the fracture toughness and of the shear yield strength on the basis of cutting forces was adopted here for timber characterisation. The set of experimental data was generated in a simple cutting test by using slightly modified off the shelf instrumentation. The resulting data are the slope and intercept of linear regression function of cutting forces versus chip thickness estimated for five European wood species. Both parameters are usable for computation of fracture toughness and shear yield strength in the shear plane, separately for two anatomical directions of wood. The simplicity and reliability of this method provides a wide range of practical applications, including structural health assessment of the timber structures.
Introduction
The knowledge of the fracture toughness in construction materials is crucial for estimating the real load carrying capacity of structural members, especially these possessing defects (e.g. wood knots, cracks), flaws or geometrical irregularities such as notches (Jockwer, Steiger and Frangi Citation2013). Data regarding toughness can be used for predicting crack stability of damaged member, according to the so-called ‘damage-tolerant design’ approach. It is essential to assure structural integrity assessments and proper design of mechanical connections in timber constructions. Wood, as any other orthotropic material, is characterised by fifteen material constants, all together determining its fracture toughness. Measurement of this property, especially when combined with low yield stress is a very challenging task. The linear fracture mechanics is usually used for its assessment assisted with experimental data acquired by means of various techniques. Most of these are semi-destructive and can be applied for the fracture toughness assessment of diverse materials. The most frequent methodologies include
indentation tests,
scratch-cutting tests,
pull-out tests,
wedge splitting tests,
energy release rate methods,
near-tip displacement field analysis.
Indentations tests are based on the correlation between hardness and fracture toughness of materials. Various indentation tests have been developed, based on either dynamic or quasi-static indentation that can be performed at the micro or macro level (Kruzica et al. Citation2009). Scratch-cutting test is based on the correlation between fracture toughness and scratch resistance/hardness. Pull-out tests are frequently used to estimate interfacial fracture toughness (e.g. in fibrous materials). However, for some materials such as wood, fracture surfaces do not have the stubble-like appearance required for pull-out. Indeed, in these cases, the fracture behaviour cannot be explained by the ‘pull-out’ contribution to toughness (Akonoa and Ulm Citation2014). Wedge splitting tests, as used by Stanzl-Tschegg et al. (Citation1995) were applied for the estimation of wood fracture toughness. The energy required to initiate and propagate a crack can be measured during stable crack growth tests as in the energy release rate method proposed by Anderson (Citation2005). The near-tip displacement fields and orthotropic theory allows estimation of the fracture toughness by analysing displacement fields obtained by means of digital image correlation (Samarasinghe and Kulasiri Citation2000) or image analysis (Vasic et al. Citation2009).
Recently, several authors carried out cutting tests to determine the fracture of various materials. These works were usually conducted on the basis of the Atkins and Vincent’s theory (Citation1984) of critical fracture energy (Williams et al. Citation2010). The specific research dedicated to wood and wood-based materials were carried out by Huang et al. (Citation2000) and Beer et al. (Citation2005), among others. Theoretical background for the original method suitable of predicting fracture toughness and shear yield strength has been recently patented by Orlowski et al. (Citation2013). It was demonstrated that it is possible to simultaneously determine yield stress along the fibres direction τγ||, yield stress perpendicular to the fibres τγ⊥, fracture toughness along the fibres R|| and fracture toughness perpendicular to the fibres R⊥ after only four cutting tests. In that case, it is required to assure minimum two diverse levels of feed speed and at least two varying chip thicknesses h (m) during cutting experiments. A set of linear equation (1) for cutting force per tooth Fc in sawing is determined on the basis of such experimental results(1)
Both, slope a (N m−1) and intercept b (N), are closely related to the values of shear yield strength and fracture toughness, respectively. The method has a great potential for on-line determination of material mechanical properties, but has some limitations regarding in-field application. Furthermore, increasing a number of cutting tests may reduce the estimation error and increase the reliability of results. It was proposed therefore, to develop an alternative approach for filling the set of linear equations by performing experimental cuttings with varying feed speed. In that case, only two cutting experiments are necessary, providing high number of data points, resulting in more general and accurate modelling of Fc.
The goal of this Short Term Scientific Mission (STSM) was to develop such a novel system capable to generate set of linear equations suitable for simultaneous determination of fracture toughness and shear yield strength in field conditions. That system would be an alternative approach for assessing timber structures and can support decision of the expert regarding the maintenance. The specific challenge of this STSM was to design the experimental procedure using light-hand woodworking tools as well as to develop and test the prototype device.
Materials and methods
Portable woodworking machine
Several handy woodworking machines were considered as experimental platform, including handy electro tools, such as circular saws, routers and planers. It was assumed that the configuration of electrical planner was most promising, as the depth of cut is relatively small, assuring minimal damage for the timber members due to cutting test. The machine used for testing was Bosch PHO 20-82 (Bosch GmbH), with a single cutting edge and cutting angle of 70°. The cutting tool edge was sharp. The planer selected is light, versatile and easy for machine operation, suitable also for straightforward assessing of timber structures in-field. The instrument assures sufficient dynamics of the electrical power changes due to varying cutting conditions, enabling nearly real-time and reliable cutting forces estimation. The off the shelf machine has been upgraded by installing additional sensors providing supplementary information as regarding the cutting process
rotary encoder used for monitoring the feed speed of machine over the measured sample (connected to the PC with USB port)
fibre optic proximity probe (Keyence FS-V10) used for real-time measurement of the cutting speed by means of rotational speed of the spindle (connected to the PC through A/D conversion board)
Estimation of the cutting power
The cutting forces of the electrical planer were measured indirectly by means of the electrical active power Pe. Dedicated metre consisted of signal conditioning module MeetBox (IRS) and A/D conversion board USB-6008 (National Instruments) connected to the laptop PC. The signal acquired was processed in LabView 2013 (National Instruments) with the Electrical Power Toolbox (National Instruments).
Software for monitoring of the cutting process
The dedicated software has been developed for data acquisition, as well as for post-processing and data mining. The time-released data recorded by the system included: measurement time ti (s), depth of cut Hp (m), current cutting length di (m), tooth passing frequency ftp (Hz), active power Pe (W), apparent power (VA), reactive power (VAR), power factor cosϕ (rad). All the above data were stored in the log file and used for post-processing. The software extracted power in idling (corresponding to the preliminary phase when tool was rotating, but no energy was used for cutting), as well as determined time stamps corresponding to the cutting start and stop. The feed speed vf(ti) was computed as in equation (2) by combing information of the current cutting length di and measurement time ti
(2)
Accordingly, the feed per tooth fz was computed for each time slot ti as in equation (3)(3)
The average chip thickness was computed according to the equation (4), assuming the cutting geometry as presented in
(4) where Hp, depth of cut; τ, contact length of the tool during cutting; D, cutting diameter
1 Cutting configuration when processing wood with rotating tool; vc, cutting speed direction; vf, feed speed direction; D, cutting diameter; fz, feed per tooth; Hp, cutting depth; h, average uncut chip thickness; ϕ, angle of the tool contact during cutting; τ, contact length of the tool during cutting
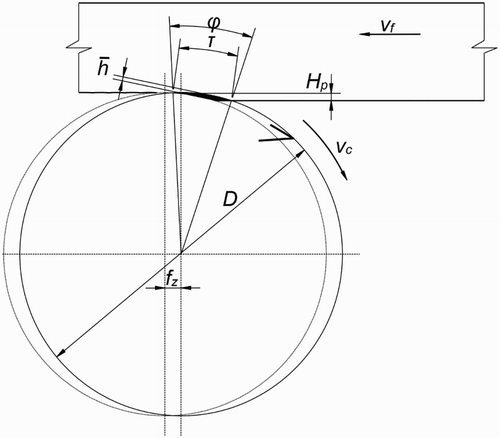
Calibration and testing of the prototype
Both, the prototype hardware and software, were extensively tested and calibrated during STSM in order to optimise the set-up and to refine the quality of information acquired. Each module, including feed speed (cutting length) encoder, rotational speed metre and electrical signals logger (current, tension and phase shift) was calibrated/validated separately. Only after assuring these proper functionalities, all the signals were combined together. The custom software assured synchronisation of the data acquisition triggering and proper structure of the generated log file. The repeatability of the system was assessed by analysing variability of the electrical power in idling. The result of multiple readings is as presented in and reveals that the range of the idling power estimated arbitrary in different cutting tests was between 316 and 330 W, with the mean value of 323 W. It was also observed that the level of the idling power slightly decreased along the experiment progress, what was associated to the increase of the machine temperature and corresponding reduction of the mechanical frictions of the mechanical components.
Cutting of experimental samples
Five wood species were selected for experimental evaluation of the cutting forces, including two softwoods: larch (Larix sp.) and spruce (Picea sp.) and three hardwoods: ash (Fraxinus sp.), beech (Fagus sp.) and oak (Quercus sp.). Samples were cut out from wood originated in Italy, in a form of planed boards 150 × 20 × 1000 mm3 (width × thickness × length, respectively) with two replicates per species. All samples were conditioned before testing in a climatic chamber (20°C, 60%RH) to the constant equilibrium moisture content. Each sample was processed at two cutting depths of 0.3 and 1.5 mm. The machined surface was radial and width of cut was corresponding to the tool length (82 mm). Any visible wood defects were intentionally omitted and not measured. The feed speed was varying randomly during the test in the range from 0 to 5 m min−1, assuming the whole cutting cycle duration be between 20 and 30 s. The experimental data were recorded with a frequency of 5 Hz and stored in the hard disk for further evaluation.
Results
Series of cutting tests were performed after system validation and calibration. An example of results obtained is presented in a and c, relating to an ash sample that was machined with low (0.3 mm) and high (1.5 mm) cutting depths. The magnitude of measured active electrical power (directly corresponding to the cutting energy consumed for the material splitting and new surface opening) was considerably higher when cutting depth was 1.5 mm. It confirms the state-of-the-art knowledge that higher cutting forces are expected while processing materials with high cutting depths. The low level of acting cutting forces was also related to the smaller average uncut chip thicknesses, again noticeably minor when cutting with Hp = 0.3 mm. The time-resolved raw experimental results were plotted on the scatter plot by regressing the cutting power against uncut chip thickness. As a result two charts for each wood species, corresponding to both experiments with varying cutting depths, were generated (b and d). It has to be stated here that such representation of experimental results is well-suited for direct determination of the coefficients necessary for solving the set of linear equations (1). In that case it become possible to determine the fracture toughness and shear yield strength values for individual timber members applying the procedure developed by Orlowski et al. (Citation2013).
3 Results of the cutting tests while machining ash (Fraxinus sp.) with a small cutting depth Hp = 0.3 mm a, b and high cutting depth Hp = 1.5 mm c, d
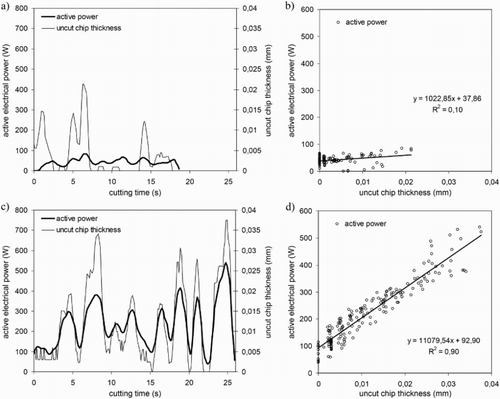
An important advantage over current methodologies is that the regression is based on a high number of experimental points, guarantying superior representation of the material characteristics variability, leading ultimately to more reliable models and conclusions. It is clear that more tests are necessary to better generalise resource properties. Nevertheless, the set of STSM results is a very promising proof of concept and is a starting point for further developments.
All the experimental results for the five investigated wood species are summarised in . Both slope and intercept coefficients of linearly regressed curves are included in the chart. It is evidenced that each wood species responded differently to the cutting. It reflects differences within fracture toughness and shear yield strengths due to genus (Sandak et al. Citation2015a), but possibly also due to provenance (Chuchała et al. Citation2014), silviculture or material ageing (Sandak et al. Citation2015b).
Summary and impact of the research
The cutting force values presented in this report are preliminary data of the pilot test. It is necessary to validate these results during additional extended experimental campaign. It was demonstrated, however, that the prototype developed within this study is capable to acquire all the information necessary for the determination of particular material properties (fracture toughness and shear yield strength) by means of the cutting test as proposed by Orlowski et al. (Citation2013). The slightly modified off the shelf electrical planer and simple data acquisition system was sufficient to achieve this goal. Even if the system worked correctly, some further modifications are foreseen in the beta version, such as enlargement of the available depth of cut in order to increase the range of the average angles of the tooth contact with a workpiece, automation of the cutting depth and of the grain angle measurements.
The results and experiences reveal high reliability of this methodology. It is expected that approach of measuring material (wood) mechanical properties with the cutting test may be a convenient and very low-destructive alternative to the recently used methodologies.
Acknowledgements
Part of this work has been conducted during Short Term Scientific Mission supported by COST Action FP1101 and within the framework of the project SLOPE receiving funding from the European Union’s Seventh Framework Programme for research, technological development and demonstration under the NMP.2013.3.0-2 (Grant number 604129) and the project BIO4ever RBSI14Y7Y4 funded within a call SIR financed by MIUR. Some results were presented on the SHATIS’15 Conference in Wroclaw, Poland. Special thanks to STSM host, Professor Kazimierz Orlowski, for his hospitality, openness, collaboration and valuable discussions. Support of Szymon Bosak during prototype development is highly appreciated.
References
- Akonoa, A. T. and Ulm, F. J. 2014. An improved technique for characterizing the fracture toughness via scratch test experiments. Wear. 313(1–2): 117–124. doi: 10.1016/j.wear.2014.02.015
- Anderson, T. S. 2005. Fracture Mechanics. Boca Raton, FL: CRC Press.
- Atkins, A. G. and Vincent, J. F. V. 1984. An instrumented microtome for improved histological sections and the measurement of fracture toughness. Journal of Materials Science Letters. 3: 310–321. doi: 10.1007/BF00729381
- Beer, P., Sinn, G., Gindl, M. and Tschegg, S. 2005. Work of fracture and of chips formation during linear cutting of particle-board. Journal of Materials Processing Technology. 159: 224–228. doi: 10.1016/j.jmatprotec.2004.05.009
- Chuchała, D., Orlowski, K. A., Sandak, A., Sandak, J., Pauliny, D. and Barański, J. 2014. The effect of wood provenance and density on cutting forces while sawing Scots pine (Pinus sylvestris L.). BioResources 9(3): 5349–5361. doi: 10.15376/biores.9.3.5349-5361
- Huang, X., Jeronimidis, G. and Vincent, J. F. V. 2000. The instrumented micro-tome cutting tests on wood from transgenic plants with modified lignification. In: Spatz H. C. H. and Speck T. (eds). Proceeding of the Third Plant Biomechanics Conference. Freiburg, Badenweiler: Georg Thieme Verlag, 475–482.
- Jockwer, R., Steiger, R. and Frangi, A. 2013. State-of-the-art review on approaches for the design of timber beams with notches. Journal of Structural Engineering. 140(3): 04013068-1-04013068-13.
- Kruzica, J. J., Kim, D. K., Koester, K. J. and Ritchie, R. O. 2009. Indentation techniques for evaluating the fracture toughness of biomaterials and hard tissues. Journal of the Mechanical Behavior of Biomedical Materials. 2: 384–395. doi: 10.1016/j.jmbbm.2008.10.008
- Orlowski, K., Sandak, J., Sandak, A. and Riggio, M. 2013. Sposób wyznaczenia odpornos´ci na pe˛kanie lub złamanie i sposo´b wyznaczenia wytrzymałos´ci na s´cinanie elementów wykonanych z materiałów ortotropowych, zwłaszcza z drewna (in Polish). Patent pending P406719 of 30.12.2013.
- Samarasinghe, S. and Kulasiri, G. D. 2000. Displacement fields of wood in tension based on image processing: part 1. Silva Fennica. 34(3): 251–259.
- Sandak, J., Sandak, A., Cantini, C. and Autino, A. 2015a. Differences in wood properties of Picea abies L. Karst. in relation to site of provenance and population genetics. Holzforschung 69(4): 385–397. doi: 10.1515/hf-2014-0061
- Sandak, A., Sandak, J. and Riggio, M. 2015b. Assessment of wood structural members degradation by means of infrared spectroscopy: an overview. doi: 10.1002/stc.1777.
- Stanzl-Tschegg, S. E., Tan, D. M. and Tschegg, E. K. 1995. New splitting test method for wood fracture characterization. Wood Science and Technology. 29: 31–50. doi: 10.1007/BF00196930
- Vasic, S., Ceccotti, A., Smith, I. and Sandak, J. 2009. Deformation rates effects in softwoods: crack dynamics with lattice fracture. Engineering Fracture Mechanics. 76: 1231–1246. doi: 10.1016/j.engfracmech.2009.01.019
- Williams, J. G., Patel, Y. and Blackman, B. R. K. 2010. A fracture mechanics analysis of cutting and machining. Engineering Fracture Mechanics. 77: 293–308. doi: 10.1016/j.engfracmech.2009.06.011