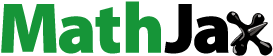
ABSTRACT
As catastrophic events become increasingly frequent, infrastructure systems face rising challenges from the dynamic environment. Enhancing the resilience of the current infrastructure system in advance has emerged as an essential strategy for catastrophe preparedness. This study explores the novel concept of resilience bonds in the context of financing flood-resilient infrastructures, using the Towyn Flood as a case study. It examines the mechanism of resilience bonds in developing infrastructure capable of withstanding floods, analysing the resilience bond value and the factors influencing its valuation. Simulation results indicate that resilience bonds can significantly extend investment resources, enhancing flood resilience and reducing unforeseen flooding risks. A sensitivity analysis provides detailed insights into how various factors impact the resilience bond's pricing model. These findings offer critical guidance for decision-makers considering the implementation of resilience bonds in flood-prone areas, highlighting their potential to improve infrastructure resilience against catastrophic events.
1. Introduction
As global temperatures continue to rise, the rate of Arctic sea ice melting is outpacing predictions by climatologists and scientists, leading to significant effects and serious attention (Kemp et al. Citation2022). These rapid climatic and hydrological shifts pose considerable risks to both society and the environment, the rising of sea level leads to a significant increase in coastal flooding frequency due to a combination of rain-induced events and an over fourfold increase in tide-induced events (Wdowinski et al. Citation2016; Woodruff, Irish, and Camargo Citation2013). As per the report from IPCC (Citation2019), the mean sea level is projected to rise between 0.24 and 0.32 m by 2050 and around 0.84 m by 2100 which will have significant and far-reaching impacts on the environment, societies and economics around the world. The year 2022 has been recorded as an unusually warm year, particularly noticeable in temperatures that have risen rapidly above the average temperatures from 1960 to 2022. Since the early 1900s, sea levels around the U.K. have risen by 18.5 cm, with a remarkable acceleration in this trend. This period has also seen a slight rise in the occurrence of heavy rainfall events (Kendon et al. Citation2023). The increasing frequency and severity of such events are linked to climate change, raising concerns about the growing risk of insurance claims and difficulties in insuring properties against flood damage. In early 2024, the U.K. experienced significant flooding due to Storm Henk, which caused around 150 million pounds of insured losses and nearly 2000 damaged properties. The heavy rainfall led to an overflow of major rivers and the government issued over 300 flood warnings. Recent decades have seen a rise in unanticipated disasters, particularly floods, leading to substantial losses. Tellman et al. (Citation2021) estimate that nearly 3% of the global population has been impacted by at least one flood event since 2000, with these individuals experiencing an average of three flooding incidents, resulting in total exposure costs between $735 and $892 million. In general, victims of weather events were mainly supported by governments through loans and grants that led to a significant burden on public resources (Brody et al. Citation2017). However, Schelfaut et al. (Citation2011) considered that the risks from catastrophic flood events cannot be eliminated, the emphasis should be on mitigating their impacts on communities and enhancing the resilience of existing flood defence.
With the increasing emphasis on resilience, the development of flood-resilient infrastructure is gaining popularity to reduce the impact of floods on coastal areas, because resilient infrastructure-based flood defence would continue to deliver its core function even during unforeseen catastrophic events. However, the U.K.’s infrastructure investment is facing uncertain economic conditions with high global inflation in recent years. For instance, the central government is the primary funder of flood and coastal erosion risk management (FCERM) in England, contributing 90% in the financial year ending 2022; Additionally, this expenditure in England has seen a rise from £777 million in 2018 to £1.063 billion in 2021 (ONS Citation2023). A government-only investment programme will, however, restrict the improvements to the infrastructure system, particularly challenging in project delivery for flood-resilient infrastructure projects. The development of infrastructure is a key factor to economies worldwide, but an ‘overinvestment’ of taxpayers’ money in infrastructure will shorten the public sector budget (Inderst Citation2016). Resilience bonds, a novel type of fixed-income bond, have recently emerged as an innovative financing tool, which is specifically aimed at financing resilient infrastructure projects that deliver positive environmental, economic, and climate benefits. These bonds are designed to meet the growing demand for investment in resilient projects, supporting current trends that highlight the necessity of such investments and release the heavy burden on public resources. The resilience bonds are debt instruments that can be issued by governments, municipalities, development banks, or other entities to raise capital from impact investors. As per Re:Focus (Citation2015), the resilience bonds integrate incentives to accelerate and expand the efforts in improving infrastructure resilience and widen the funding resources from private investors to cover the upfront costs associated with upgrading or fortifying the existing infrastructure systems. The mechanism of resilience bonds collaborate risk-sharing mechanism and financing model ensures that no single stakeholder bears the entire burden of repayment. It distributes the responsibility for reimbursing investors over time by convening stakeholders who stand to benefit from the project.
This paper addresses the increasing risks associated with catastrophic flooding due to climate change, explores alternative financial mechanisms that can provide a more sustainable, and economically viable way to enhance infrastructure resilience against flood risks. As a newly introduced financial instrument, there is limited literature exploring the application of resilience bonds. This study aims to bridge the gap in literature and practical knowledge about the application and effectiveness of resilience bonds, which can potentially release the heavy burden on public resources and attract private investment in infrastructure resilience. Through these efforts, the contribution lies in advancing the comprehension and practical implementation of resilience bonds, especially within the field of flood resilience initiatives. The proposed framework for the application of flood resilience bonds holds the potential to unlock opportunities for creating positive social impacts in the broader landscape of infrastructure development. In the context of limited financial resources for infrastructure investment, this article demonstrates the transformative role flood resilience bonds can play in addressing this pressing issue. Through a detailed case study focused on the historically severe Towyn flood of 1990, we investigate how resilience bonds can facilitate the journey towards a more resilient ecological and economic future for communities and regions faced with similar challenges. As the pioneering publication dedicated to financing resilient infrastructure projects through resilience bonds, this work serves as an incentive for stimulating interest in infrastructure investment by signalling to the market that there exists an innovative avenue for raising capital and sharing risks for infrastructure investment.
The paper is structured in nine sections which serving a specific purpose. Section one provides an introduction to the research topic and outlines the objects and contributions of this study. Section two reviews the potential challenges associated with flood resilience and highlights the mechanism of resilience bonds and similar financial instruments. Section three illustrates the mechanism of flood resilience bond and its application to Towyn. Section four methodology used to elaborates the specification to assess the value of flood resilience bond. Section five focuses on the analysis of data and presenting the results obtained from the case study. In section six, a sensitivity analysis is conducted to explore the influential factors that can affect the valuation model. Section seven considers the limitations of this research. Finally, in the last two sections, the paper discusses the policy implications of the research and presents the overall conclusion of the study.
2. Literature review
2.1. Challenges of flood resilience and infrastructure financing
In recent years, climate change has been identified as the primary cause of the increasing frequency of flooding events. According to Tabari (Citation2020), the direction of changes in flood occurrence is linked to changes in extreme precipitation, particularly in regions where precipitation is the primary cause of flooding. In the U.K., extreme flooding has increased with three persistent heavy rainfalls in the summer of 2007 led to the worst flood event in 60 years, killing 13 people, trapping almost 7000 people, and impacting about 55,000 properties (Blackburn, Methven, and Roberts Citation2008; Mendoza-Tinoco et al. Citation2017). The frequent inundation puts almost 5 million properties at risk, which costs £1.1 billion worth of losses annually in the region and the unforeseen inundation damages are expected to reach £27 billion by 2080 (Bennett and Hartwell-Naguib Citation2014). A flood is a low-probability event but usually causes high consequences when it occurs. Enhancing the resilience of critical infrastructure against flooding intensified by climate change is a crucial strategy for minimising damage and expediting the recovery process (Vamvakeridou-Lyroudia et al. Citation2020). The concept of ‘resilience’ first emerged in studies of the ecosystem, referring to its capacity to persist despite catastrophic events (Holling Citation1973). Later, Holling widened the definition of resilience in the field of engineering to describe the recovery speed of a system to its equivalent original status from unexpected events (Holling Citation1996). Batica and Gourbesville (Citation2016) assert that a resilient flood system and community should possess the capacity to live with catastrophic floods, sustain their core function continuously before or after such events, promptly recover to their original state and establish a database to prepare for future unknown events. In the market, the concept of ‘resilient infrastructure’ is gaining prominence among both public and private sectors across different societies. It aims to enhance the resilience of infrastructure-based systems to resist natural disasters rather than simply build and maintain infrastructures (Mizutori Citation2019). For example, hybrid flood barriers, which combine flood defence infrastructures with salt marshes, have proven effective in fortifying flood protection (Marijnissen et al. Citation2020; Siemes et al. Citation2020). However, despite the increasing emphasis on building resilience in flood-related discussions, the actual implementation of resilience measures has been limited, primarily due to concerns about funding (Proverbs and Lamond Citation2008). The construction, operation, and maintenance of flood defence infrastructure account for a significant portion of government expenditures in flood risk management strategies (Dawson, Hall, and Davis Citation2004).
The infrastructure projects have strained government budgets to their limits, given that public resources have competing demands and high opportunity costs for other societal projects. According to Bennett and Hartwell-Naguib (Citation2014), the maintenance costs of current flood defences will rise to over £1 billion annually by 2035. The funding shortfall has prompted the government to introduce a new flood defence funding system, aiming to involve additional entities and investments in the development of flood defence systems. Financing issues consistently take precedence in infrastructure investment discussions worldwide, as governments globally grapple with limited public resources. According to Larsen, Christianson, and Amerasinghe (Citation2019), bonds provide attractive opportunities for both private and public sectors to secure low-cost borrowing from capital market investors. They are recognised as highly effective financial instruments for raising funds for infrastructure projects, including the enhancement of flood barriers. Sufficient financial investment is a crucial prerequisite to ensure the successful completion of infrastructure initiatives. However, conventional insurance-linked securities like catastrophe bonds and longevity swaps primarily focus on post-catastrophe reimbursement and risk transfer to investors, without actively supporting proactive resilience development. To provide comprehensive protection for residents in flood-prone areas, a ‘double glazing’ strategy is needed, combining insurance services with the advancement of resilient infrastructure-based flood defences. In this context, the resilience bond offers significant potential advantages by addressing both post-disaster reimbursement and upfront investment funding for resilient projects.
2.2. Resilience bonds and similar financial instruments
According to Re:Focus (Citation2015), a resilience bond is a financial tool where investors provide initial funding through bond purchases to support resilience projects, such as building coastal barriers or enhancing building resistance to natural disasters. These projects encompass endeavours like constructing coastal barriers or upgrading buildings to enhance resistance against natural catastrophes. The payout mechanism of a resilience bond involves a predetermined set of resilience objectives that the issuer must achieve to be eligible for payment. If the issuer fail to meet these objectives, the payout may be decreased or withheld entirely. The payout itself can be in the form of a fixed or variable coupon, depending on the specific terms outlined in the bond agreement. Particularly, the resilience bond possesses a distinctive feature which generates rebates based on the overall risk reduction achieved by the resilient projects to the entire system. In other words, a designated third-party will predict and quantify the mitigated risks resulting from the implementation of resilient infrastructure projects, and then, the assessed economic values from the risks anticipated will be deducted from the premium and principal. The deducted amount will be transformed into a rebate, which will be reinvested into the resilient projects during the initial stages of construction. Applying the resilience bond offers a significant benefit, which the risk mitigation value is immediately reinvested into the continuous development of the project, thereby enhancing the overall effectiveness and resilience systems (Vajjhala Citation2015).
This mechanism in is created to incentivise the issuer to take steps to improve resilience to climate changes and natural catastrophes, while also attracting investors with the opportunity to invest in socially responsible projects that have the potential to generate positive social and environmental impacts (Vaijhala and Rhodes Citation2018). Conventional bonds typically have a common maturity ranging from 3 to 5 years, similar to resilience bond which is appropriate for aligning with the construction timeline of resilient projects. It is essential to consider that certain projects may require a longer construction period, for example, the construction of the Thames Barrier in London extended over 8 years, surpassing the standard timeframe. Hence, the resilience bond can be issued in multiple tranches to align with a flexible duration of the construction for the resilient projects. The risk mitigation efforts are constantly evaluated, allowing for the distribution of rebates that can be reinvested in the project, further supporting its advancement.
Figure 1. Resilience bond mechanism. Source: Re:Focus (Citation2015).
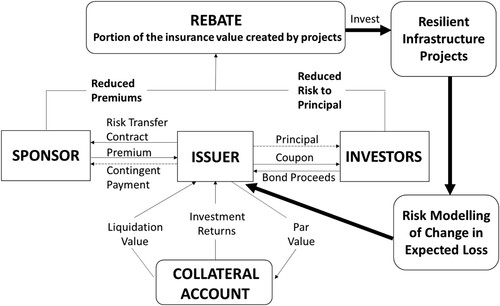
By considering of sustainable and resilient infrastructure investment, two notable financial instruments have served a similar purpose and presents different mechanisms and outcomes apart from resilience bonds. These similar financial instruments that available in the market are Environmental Impact Bonds (EIBs) and Green Bonds. As an innovative financial instrument, Environmental Impact Bonds are used to fund projects with specific environmental goals, it plays a role in addressing the environmental funding gap by providing upfront capital for environmental projects and repaying investors based on the project's success. According to Brand et al. (Citation2021), EIBs are similar to traditional bonds which involve borrowing principal from stakeholders or beneficiaries with a promise of repayment over time. Unlike traditional bonds that often repaid the bond holders by using general revenues from the issuer, EIBs specifically emphasis the financial return on the investment to the success of the environmental intervention. The returns are generated from the revenue and/or cost savings related to the success of these projects and particularly EIBs can provide upfront funding for large-scale environmental projects. However, Trotta (Citation2024) shows that the significant challenge of EIBs is the difficulty in verifying and measuring environmental outcomes, which is crucial to define the success of the issuance for the bonds. In addition, Green Bonds function as a financial instrument for raising capital for environmentally sustainable projects which are issued by governments, financial institutions, or corporations. The bond is focusing for financing or refinancing projects with environmental benefits, like renewable energy, energy efficiency, sustainable waste management, and pollution control. The issuer will define the bond in advance to specifying the amount, promising of repayment over the pre-defined maturity period and interest rate (coupon). These bonds are often certified by a third party to ensure their environmental credentials (Tuhkanen Citation2020; Sartzetakis Citation2020; Katori Citation2018). Issuing green bonds will require higher upfront costs and complexity that include costs related to the certification process, ongoing reporting requirements, and potential audits to ensure the funds are used for the intended green projects. Currently, the demand for Green Bond is higher in the market than the supply. As Larsen, Christianson, and Amerasinghe (Citation2019) and Clapp and Pillay (Citation2017) state Green Bonds attract investors who are interested in environmental sustainability and investors, however, some projects funded by green bonds may not deliver the expected environmental benefits as real ‘green’ projects since this can happen if the criteria for green projects are not strict or well-enforced.
Compared to the context of the investment in resilient infrastructure projects that mitigate risks associated with flood disasters, resilience bonds are narrowly focused on disaster risk reduction, making them highly targeted for resilient infrastructure investment. Environmental impact bonds are more suited for projects with direct environmental benefits. Green bonds have the broadest scope to fund a wide range of sustainable projects, including those contributing to resilient infrastructure. However, green bonds do not have a performance-linked return like resilience bonds and EIBs which will be the key as an incentivises for the successful completion of projects. Resilience bonds and EIBs may appeal more to impact-focused investors due to their direct linkage between investment and specific outcomes. Green bonds attract investors looking to support broader environmental sustainability. Besides, resilience bonds and EIBs might have a higher risk profile due to their reliance on project success. Green bonds generally carry risks similar to traditional bonds which depend on the issuer's creditworthiness. All three bonds play essential roles in financing sustainable and resilient infrastructure, they differ in their focus, mechanism, and appeal to investors. Resilience bonds are tailored for disaster risk reduction and independent from the financial market, EIBs for projects with measurable environmental impacts, and green bonds for a wide array of sustainable initiatives. The choice among these bonds depends on the specific goals, risk appetite, and impact focus of the investors and issuer. By funding flood-resilient infrastructure projects that directly enhance resilience, resilience bonds provide a mechanism for mitigating flood risks associated with climate change and other natural events. This makes resilience bonds particularly valuable for offer a proactive approach to disaster management and risk reduction in flooding catastrophic events.
3. Mechanism of flood resilience bonds: a case study of Towyn
Towyn, a charming seaside resort situated in North Wales has gained prominence for its idyllic location. However, it is also known for a catastrophic event that occurred in 1990. According to Brockway (Citation2020), Towyn suffered a devastating flood due to low atmospheric pressure, high-level tides and strong onshore winds in 1990. The 1990 Towyn flood has been classed as a rare event with a probability of occurring once every 500 years. If we looking back on this extreme flood event in 1990, it had lasting influence to Towyn and also to people who lived near coastal. The flood barriers in Towyn were breached by an extreme storm that coincided with a high tide, resulting in a surge of water reaching approximately 1.5 metres in height. Helicopters and lifeboats were swiftly deployed to rescue and evacuate people as the floodwaters swept past the railway, inundating caravans and houses. This unprecedented event set multiple records, with 4.5-meter high waves and a simultaneous 1.3-meter storm surge occurring during high tide. The flood wreaked havoc, causing significant damage, breaching the railway embankment, flooding the streets, and impacting around 2800 homes and a staggering 5000 individuals had to be evacuated (Bates et al. Citation2005). The devastating consequences of the Towyn flood, coupled with more recent instances of flooding, have spurred coastal residents into action, advocating for enhanced protection and resilience measures for their town, homes, and livelihoods.
The present paper proposes the application of a resilience bond, using the extreme catastrophic event of Towyn flood 1990 as a case study, to model investment in resilient flood barriers project in Towyn. The mechanism creates a positive cycle, facilitating the ongoing improvement of flood infrastructures. The Towyn flood resilience bond mechanism incorporates three primary functions: insurance services, rebates, and investment. The insurance function operates similarly to traditional catastrophe bonds, enabling entities in Towyn to access insurance services from the market (Polacek Citation2018; Chen et al. Citation2013). For example, residents can pay a premium and receive repayment contracts for flood events in return. To distribute unforeseen risks, a Special Purpose Vehicle (SPV) will issue the flood resilience bond on behalf of (re)insurance companies, bridging the gap between the insurance market and the capital market. The Towyn local authorities could gain advantages from the issuance of resilience bonds, as investor purchases of these bonds enhance the repayment capacity of issuers and enabling risk diversification. The key players involved in Towyn flood resilience bonds are similar to those involved in traditional bond issuances, with a particular focus on resilience and sustainability. These players work together to raise capital, assess risk and manage resilient flood barriers projects to ensure that they are successful in meeting their resilience targets. If the resilience projects fail to meet the specified outcomes, the issuer may be required to repay a portion of the bond principal to investors or provide compensation in some other form. On the contrary, if the projects achieve their resilience objectives, investors receive interest payments or a return of their principal, along with any additional financial incentives specified in the bond's terms. According to Vaijhala and Rhodes (Citation2018), leveraging non-public investments to enhance flood defence projects, the financial burden on governments by supporting infrastructure against natural disasters and climate-related events can be released. Entities that may interested in investing in the Flood Resilience Bond are those who could potentially be impacted by unforeseen flooding events in Towyn. This includes residents, communities, local authorities, and different infrastructure sectors that directly affected by such events. Recognising the potential economic losses that these parties may face in the event of a flood defense system failure, the bond offers a strong incentive to invest in initiatives that enhance flood resilience. By investing in the Flood Resilience Bond, these entities can actively contribute to the development of robust flood barriers for Towyn and protect themselves against unexpected economic losses resulting from flooding events.
As a newly introduced bond, resilience bonds face several challenges alongside their potential to enhance flood barriers in Towyn. A primary challenge is accurately quantifying the economic value of risk reduction achieved by resilient flood barrier projects. This quantification is complex, compelling comprehensive data collection, sophisticated modelling, and in-depth analysis. Another significant challenge lies in generating investor interest. Resilience bonds appeal to a specific group of investors, such as institutional investors, pension funds, insurance companies, and local infrastructure sectors, who are prepared to assume the risks associated with similar flooding catastrophes. These limitations will influence the liquidity of the bond and increase the potential borrowing costs for issuers. Additionally, the triggering and payout mechanism for the valuation of resilience bonds relies on a case study with the data generated from a specific resilient project. Therefore, this paper is focusing on the design of a valuation model and payout mechanism, using Towyn flood event as the case study to simulate a flood barrier projects. It proposes a valuation model for a flood resilience bond, incorporating a range of parameters derived from flood events. This approach aims to address the complexities involved in deploying resilience bonds for flood resilient projects, balancing the challenges and opportunities for sustainable infrastructure development.
4. Methodology
Estimating the expected price of resilience bonds involves a series of steps. Initially, a stochastic model is employed to analyse time-series data, assess the instantaneous interest rate, and simulate random catastrophic events. Subsequently, a compound Poisson process is utilised to represent the economic losses resulting from flood events. For instance, the economic losses caused by breaching specific flood barriers at particular surge heights are simulated and calculated. Furthermore, it is assumed that the enhancement of flood barrier project can effectively mitigate the anticipated losses in Towyn. As an alternative financial instrument, this rational valuation model serves as a useful tool for decision makers, guiding the fair market price for the bonds, and potentially increasing the attractiveness to investors by providing a clearer understanding of the risk and return.
4.1. Frequency and severity of catastrophic flood and economic losses in Towyn
Dynamic loss is changed by either surge level or different flood barriers breaches. Modelling the dynamic losses and subtracting the amount of losses mitigated from resilient infrastructure projects are the most significant components of the flood resilience bond, which is also an unique function to be distinguished from other financial instruments. As in Burnecki, Janczura, and Weron (Citation2011), the dynamic loss process is divided into two parts in the typical insurance risk model: frequency of catastrophe events and severity of the occurrence of the event. The two main components are defined as the counting process of events, [], where
and total claim size
where
. Therefore, the cumulative catastrophic flood losses at period
is modelled by
(1)
(1) where
is the aggregate loss of
,
if
;
follow the Poisson process that indicates the occurrence of catastrophic events at the period
. In particular,
in which
is the intensity of the process. For the severity of flood events,
represents the random variable of the cumulative post-flooding economic losses distribution, which is the combination of each independent events
with the random frequency of n defined by
. In addition, the catastrophic event economic losses are nonnegative, independent and identically distributed values (
), with no event occurring at the same time. Let distribution function F(x) represents the distribution of
, where,
. In order to define the distributions of
, the author conducted a simulation to generate economic losses data which extended the research from (Dawson, Peppe, and Wang Citation2011). The simulation created three-level (4, 5, and 6 m) surge events to test the flood barriers in Towyn and computes the aftermath economic losses for each independent value of
. The simulated data is vital for evaluating the distribution of dynamic losses and the distribution to describe the data will be tested by Kolmogorov Smirnov, Anderson Darling, and Chi-Squared tests to perform the goodness of fit.
4.2. Simulation of flood barriers breach economic losses in Towyn
Resilience bonds are designed based on the concept to support resilient infrastructure projects that effectively mitigate risks and enhance the overall system's resilience (Re:Focus Citation2017). In the context of Towyn, this paper presents a simulation of a project aimed at enhancing the existing flood barriers. This simulation based on the historical catastrophic event Towyn flood 1990 serves as a real-world illustration of the devastating consequences of flooding and has generated a valuable data, encompassing damage records, response efforts, and insights into community resilience. These insights can be leveraged to develop predictive models and algorithms that account for worst-case scenarios, thereby enhancing the assessment of bond values. The goal of this resilient project is to mitigate risks and enhance the flood barriers against a range of surge levels, thereby creating a more robust and resilient flood defense system. The conceptualisation of this flood resilience bond in this scenario is instrumental in facilitating the execution and success of such an flood barriers enhancement project, which may significantly improving Towyn's ability to endure and recover from flooding incidents. Furthermore, the findings derived from the case study offer valuable input for shaping policies related to flood risk management, disaster preparedness and infrastructure investment.
illustrates the location of 5 specific flood barriers in Towyn that are chosen due to the coverage of a town area situated within a 3 to 5-metre elevation range. To determine the effectiveness of the flood barriers enhancement project, the analysis concentrates on post-breach economic losses from the flood barriers at points F,G,H,I and J. For instance, scenarios where any of these 5 barriers are at risk of failure in the simulation, the model generates three different surge levels (4, 5, and 6 m) to assess the barrier’s effectiveness and calculates the economic losses of breaches. This process creates a variety of combinations of breach scenarios under each surge level, which are then documented to form a comprehensive database. To calculate the breach probabilities of flood barriers, designated as . The approach is established in probabilistic analysis and employs Bayes’ Theorem for enhanced accuracy.
Figure 2. Flood barriers in Towyn, Wales. Source: adapted from Dawson, Peppe, and Wang (Citation2011).
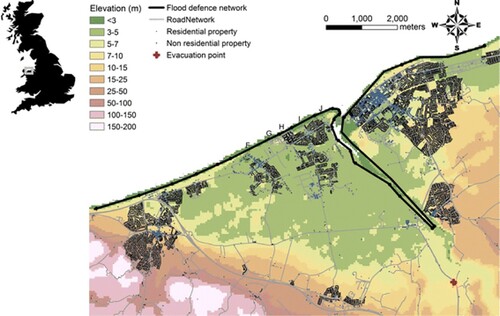
Initially, we define the breach probability of a flood barrier given a specific condition as follows:
(2)
(2) In this formulation,
represents the environmental conditions that affect the likelihood of a flood barrier failing, each
is a distinct scenario that could influence the barrier being evaluated.
indicates the probability of a breach
occurring under the condition
. Further, applying Bayes’ Theorem, we derive the conditional probability of the state
given a breach has occurred as
Assuming uniform probability across all conditions,
, the breach probability of the flood barriers can be calculated by
(3)
(3)
Lastly, to determine the probability of at least one barrier experiencing a breach, we utilise the complement rule:
(4)
(4) The calculation of
provides the overall likelihood of at least one barrier failing. This comprehensive probabilistic framework assesses the reliability and safety of flood barrier systems.
4.3. Flood resilience bond payoff mechanism
In the mechanism to pricing a bond, this paper align with the approach of Brigo and Mercurio (Citation2007) by simplifying bond pricing consideration to zero-coupon bonds. This simplification is particularly useful in the context of catastrophic events like floods, where rapid assessment and decision-making are crucial. Zero-coupon bonds, which are typically traded below their face value, offer a viable option for funding public resilience projects against such disasters. Predominantly issued by public authorities, these bonds provide an effective mechanism for governments and municipalities to finance flood resilience initiatives. The market for zero-coupon bonds is well-established and active, providing liquidity to investors who may choose to convert the bond before its maturity. This flexibility allows investors to capitalise on the bond's value without necessarily holding it until its maturity date. For the pricing of the bond at specific time, , we denote the price of a zero-coupon bond with a face value equal to 100 at the maturity date T as
. To value the price of resilience bond (
), the valuation framework of the flood resilience bond under the risk-neutralised pricing measure, Q, is given by
(5)
(5) Applying the Cox-Ingersoll-Ross (CIR) model to represent the dynamic interest rate, the expectation of risk-neutral measure is equal to the price of a pure-discount zero-coupon bond, which as
. The proposed resilient project aims to strengthen Towyn’s existing flood barriers, thereby reducing the likelihood of failure during extreme flood events. To quantify this risk reduction, an resilience impact parameter (ѯ) is introduced, representing the potential decrease in loss percentages achievable through the flood barriers enhancement project. This parameter is a critical component of the model, as it measures the effectiveness of the resilience interventions in mitigating flood risks. The payoff function and the value framework of the flood resilience bond are transferred in the equations
Then, we can obtain the final flood resilience bond pricing model that expressed as
(7)
(7) where
– is payoff amount of resilience bond at maturity;
– is the proportion of the face value repaid to investors;
– is face value of resilience bond;
– is aggregate losses; D – represents trigger value (threshold); ѯ – is the impact of the resilient project, where 0<ѯ<100%.
– is dynamic interest rate by using the CIR model;
is the distribution of the economic losses less than or equal to threshold, D.
This paper introduces a piecewise function for valuing the flood resilience bond, building on the research of Zimbidis, Frangos, and Pantelous (Citation2007) and Shao, Pantelous, and Papaioannou (Citation2015). This method is used to present the simulation of flood dynamic losses across three distinct surge levels. Consequently, the valuation framework for the flood resilience bond is formulated as a piecewise function, which effectively captures the varying conditions influenced by flood dynamics. The final valuation framework of flood resilience bond can be expressed as:
where – is value of the flood resilience bond at time t;
– is dynamic interest rate with an application of CIR model;
– is surge height, where 3 m < S < 7 m; D – represents trigger value; the trigger value is set within the range: low boundary as
and high boundary as
;
– is the proportion of the face value repaid to investors if bond triggered;
– is the face value of the resilience bond;
– is the probability of dynamic losses generated from different levels of surges; ѯ – is resilience impact from flood defense resilient project, where 0 < ѯ < 1.
5. Data and results
To assess the flood resilience bond for Towyn, this study implemented a simulation to estimate the post-flooding economic losses in Towyn. Extending the model from Dawson, Peppe, and Wang (Citation2011), the simulation examined three different surge levels – 4, 5, and 6 m – and their impact on five flood barriers (F, G, H, I, and J). The study took into account the individual responses of each barrier to various surge levels and how different combinations of breached barriers could lead to varying economic losses in Towyn. Analysis of the data set revealed that the probability distribution of the economic loss data exhibited a thinner tail compared to an exponential distribution. Consequently, the data were examined into appropriate distributions and evaluated using Kolmogorov–Smirnov, Anderson–Darling, and Chi-Squared tests to ensure accuracy and reliability. The results obtained as follow:
presents the results of various distribution fitness tests. The comprehensive outcomes of these tests suggest that the Generalised Extreme Value (GEV) distribution is more apt for characterising the simulated data. Consequently, the GEV distribution has been adopted to model the trend of economic losses resulting from the three different surge levels. This approach is supported by practical records, which provide variable factors for each of the surge levels.
Table 1. Distribution fitness tests.
identifies the probability of the occurrence of different level surges. Given that the five flood barriers (F, G, H, I, and J) are crucial for protecting the section of the town situated within a 3- to 5-meter elevation range, the breach probability for each barrier can be calculated by using EquationEquation (3)(3)
(3) to obtain:
Table 2. Probability of surge levels.
According to of the breach probability for each flood barrier, the probability of at least one flood barrier breach can be computed using formula (4), where
To define the distribution of post-flooding economic losses
, the data generated from the three different surge heights were tested in several distributions, including Weibull distribution, Normal distribution, Generalised Extreme Value distribution, and Lognormal distribution. These distributions were then subjected to Kolmogorov–Smirnov, Anderson–Darling, and Chi-Squared tests. The findings indicate that the GEV distribution most accurately represents the pattern of post-flooding economic loss data. According to Stevens, Clarke, and Nicholls (Citation2016), 785 national flooding events have been recorded in the U.K. in the past 100 years. The data reveals a notable increase in reported flooding incidents during the 20th and 21st centuries. Consequently, it is presumed that the frequency of these events
follows to a homogeneous Poisson process (HPP), with an intensity (λ) of 7.85. This implies that the surge events are considered to follow a Poisson process with independent occurrence probabilities. Moving forward, this paper adopts the model from Nowak and Romaniuk (Citation2013) to determine the parameters for the dynamic interest rate. These parameters are then integrated with the Generalised Extreme Value (GEV) distributions that corresponding to the three different surge levels and the intensity of HPP, to compute the pricing of the resilience bonds.
Table 3. Probability of breach for each flood barrier.
presents the parameters utilised in the final valuation framework (8) to calculate the price resilience bond. Considering a face value of 100 for the resilience bond, the calculation is performed under the scenario where the enhancement of the flood barriers project contributes 50% resilience to the overall system. Then, the value obtained for the flood resilience bonds are as follow:
Table 4. Parameters of flood resilience bond pricing model.
demonstrates the pricing of a flood catastrophe bond in a scenario where no investment is made in resilience projects, effectively representing a 0% resilience. This scenario is then contrasted with the pricing of a flood resilience bond, which reflects a 50% increase in resilience due to an effective flood barrier enhancement project. The comparative shows distinct pricing differences between the two types of bonds. Additionally, the data in and offers a detailed prices for both the flood catastrophe bonds and the resilience bonds over a five-year period, taking into account a variety of trigger thresholds. Similar results for catastrophe bond can be found in (Ma, Ma, and Xiao Citation2017) and (Burnecki, Kukla, and Taylor Citation2011).
Table 5. Price of a flood catastrophe bond.
Table 6. Price of flood resilience bond with 50% resilience.
Observing the price progression over a five-year period across various thresholds, it becomes apparent that the prices of these bonds tend to converge towards their face value as they near maturity. At the highest threshold of 28 million, the price of the flood resilience bond in the fifth year is 76.18, whereas the catastrophe bond is priced at 74.19 under the same conditions. This indicates a yield of 5.48% for the resilience bond and 6.15% for the catastrophe bond. These yields reflect the risk-return profiles of both bond types, with the resilience bond offering a slightly lower yield, suggesting a perceived lower risk compared to the catastrophe bond. Furthermore, if the insurance contract sets the threshold at 14 million, the yields exhibit a notable difference at the end of the five-year period. The resilience bond's yield stands at 6.06%, while the catastrophe bond significantly jumps to 15.63%. This significant contrast in yields under the 14 million threshold highlights the threshold's crucial role in influencing bond pricing. It underscores how the set threshold impacts the risk assessment and expected returns of these bonds. The threshold acts as a critical factor in the pricing model, affecting the bond's price variability. It essentially determines the bond's sensitivity to loss events, influencing investor perception and, consequently, the bond’s market value. This analysis underscores the importance of carefully setting thresholds in bond contracts, as they can significantly impact the attractiveness and performance of resilience and catastrophe bonds in the market.
6. Sensitivity analysis
The sensitivity analysis in this paper aims to guide decision-makers about the market pricing of the resilience bond. It examines the influence of various input variables on the bond's pricing model. Initially, the analysis acknowledges that resilient infrastructure projects positively impact the overall flood defense system. Consequently, different levels of resilience effectiveness from the flood barrier enhancement projects are incorporated to calculate the bond's price. Next, the analysis uses economic loss data from the simulation to adjust the parameters of distributions, which are indicative of future loss trends. It's important to note that selecting varying parameters can alter the bond's pricing. To address this, the study explores a range of distribution models to accurately describe the potential severity of losses from catastrophic floods and assess their impact on the bond's pricing. In addition, the study examines the influence of different dynamic interest rate models and their parameters. This is crucial in forecasting the bond's future interest rates and pricing under diverse dynamic interest rate scenarios. The role of a resilient infrastructure project is to enhance the infrastructure system's capacity to withstand and recover from disturbances. The effectiveness of resilience can vary based on the barriers enhancement project's performance, assumptions of 10% and 90% resilience from the project are used to compute the prices of the flood resilience bonds, thereby providing a comprehensive view of potential outcomes under different scenarios of project resilience.
and present the pricing of the Towyn flood resilience bond, considering a 10% resilience contribution from the flood barrier enhancement project. The data illustrate how the bond's price evolves over a five-year period under different threshold conditions. The trend in indicates that the bond's value increases over time, particularly as the threshold for triggering payouts increases. The higher thresholds correspond to more severe flood scenarios, thus reflecting a greater risk and a higher potential payout, which is factored into the bond's pricing. The 10% resilience factor, denoting a moderate level of risk mitigation, influences these prices, suggesting that even a modest improvement in resilience can impact the bond's market value.
Table 7. Price of flood resilience bond (10%) under different thresholds.
In the following simulations, we hypothesise that the flood barrier enhancement project can achieve an ambitious 90% level of resilience for the flood defense system. Although reaching such a high level of resilience is typically considered unfeasible in practical scenarios, this extreme assumption is used for the purpose of calculating and analysing bond prices. By adopting this high-end value, we aim to explore the range of bond price movements under varying degrees of resilience. This approach allows for a comparison with the earlier scenario, where a more moderate 10% resilience was assumed. Such a contrast between the two scenarios (10% and 90% resilience) provides valuable insights into how different levels of resilience can significantly influence the pricing dynamics of flood resilience bonds.
The data presented in and illustrate the pricing of flood resilience bonds under a high resilience scenario of 90%. This level of resilience serves as a hypothetical extreme to analyse the impact on bond pricing. The findings indicate a clear trend that the resilience level of the flood enhancement project increases, the price of the flood resilience bond increases. In this scenario, the effectiveness of the flood barrier enhancement project has a direct influence on the risks and potential losses to the entire system. A 90% resilience level significantly lowers the likelihood of the bond being triggered by a flood event, thereby reducing the risk for investors. As a result, investors are willing to pay a higher price for the bond, understanding that the improved resilience of the project translates to lower risks. However, this increased price has a flipside for investors, it means they will receive lower coupon payments upon bond maturity. Since a higher resilience level diminishes the probability of bond trigger events, the returns on investment for bondholders are correspondingly reduced. This inverse relationship between the level of resilience and investor returns is a crucial consideration for both issuers and investors in flood resilience bonds. This analysis underscores the importance of balancing project efficacy with investment attractiveness when designing resilience bonds.
Table 8. Price of flood resilience bond (90%) under different thresholds.
In the following sensitivity analysis, we aim to evaluate the impact of different interest rate scenarios on bond valuation. By applying both the Vasicek and CIR models, we can assess the resilience bond's price sensitivity under various interest rate conditions. This dual-model approach allows for a more robust and nuanced understanding of the factors that influence the pricing of resilience bonds, particularly in the context of dynamic financial markets. we utilise the 12-month LIBOR historical rate spanning from 2000 to 2011 to calibrate the parameters needed for generating stochastic interest rates. As detailed in , we can better understand the sensitivity of resilience bond prices to changes in the financial environment. This aspect of the analysis is crucial for gauging the potential risks and returns associated with investing in resilience bonds under varying economic conditions.
Table 9. Parameters of flood resilience bond under Vasicek/CIR models.
In the next phase of our analysis, we compute the price of the flood resilience bond using both interest rate model. This approach allows us to explore how fluctuations in interest rates, as predicted by these models, can impact the valuation of the bond.
and illustrate how applying different dynamic interest rate models influences the pricing of the Towyn flood resilience bond, specifically with a 50% resilience effect. The analysis reveals different pricing outcomes based on the chosen interest rate model. The CIR model, recognised for its ability to avoid negative interest rates, corrects a notable limitation of the Vasicek model. By comparing with both interest rate models, it evident that the Vasicek model generally estimates a higher bond price compared to the CIR model, particularly at earlier times and lower thresholds. This is because the Vasicek model does not restrict interest rates from turning negative, which can lead to a lower discount rate and a higher present value of future cash flows. As time progresses or the threshold increases, the differences in bond prices between the two models diminish. The comparison of these models reveals how sensitive bond prices are to the underlying interest rate assumptions. The choice of the interest rate model can significantly influence the bond's assessed risk and return profile. For instance, the Vasicek model might be more suitable in a stable or low-interest environment, while the CIR model could be preferable in situations where the assurance of positive rates is critical. Decision-makers must consider these differences when evaluating the resilience bond's value and when conducting risk assessments under various economic conditions.
7. Limitations
While providing valuable insights into the potential of resilience bonds for financing flood-resilient infrastructure, this study is subject to several limitations that should be considered, which might affect the generalisability and applicability of the findings to broader contexts or different scenarios. The primary limitation of the study is it focused on a single historical event – the Towyn flood of 1990. Case studies are valuable for in-depth analysis and can provide detailed insights into complex mechanisms, their findings are inherently specific to the geographical and temporal contexts in which they are set. This specificity can limit the applicability of the conclusions drawn to other regions or different types of flood events. Future research should look to validate these findings across various geographical locations and types of flood events to enhance the generalisability of the conclusions. Besides, the effectiveness of any model or simulation is naturally limited by the quality and extent of the data it uses. The model's accuracy in this research is contingent on Towyn’s historical flood data, economic impact simulation of flood damage and historical financial market interest rate data. This study makes efforts to use the best available data, despite the unique and complex nature of data related to catastrophic events like floods, which are inherently sporadic and varied in impact.
The concept of resilience bonds is relatively novel in the realm of financial instruments. Therefore, market readiness and investor familiarity with such bonds could limit initial uptake. The actual market adoption could vary significantly depending on investor risk appetite, awareness of resilience benefits, and the regulatory environment. The implementation and success of resilience bonds are also subject to the prevailing policy and regulatory environment, which can vary widely between jurisdictions. Changes in government policies, regulatory hurdles, and shifts in political priorities can all affect the viability and attractiveness of resilience bonds as a tool for financing flood resilience. The paper's scope may not fully account for these external variables, which could be extended in the future research to enhance the understanding and deployment of this financial instrument.
8. Policy implications
The United Nations’ Sustainable Development Goals (SDGs), particularly Goal 9, serve as a strategic framework for creating a better and more sustainable future worldwide. This goal emphasises the importance of resilient infrastructure, sustainable industrialisation and fostering innovation. In line with the 2030 Agenda for Sustainable Development, there is a global focus on enhancing infrastructure, especially in areas like public transportation, goods delivery networks and making transportation more affordable, efficient and convenient (United Nations Citation2023). However, sustainable infrastructure development faces significant challenges due to the insurance gap, particularly in the context of catastrophic events. Our study highlights the underinsurance of infrastructure projects, especially during the construction phase, due to the low probability of catastrophic events. However, other risks such as political, contractual and financial risks can have significant impacts (Munich-RE Citation2023). We explored the application of resilience bonds in developing flood resilience infrastructure, aiming to enhance the existing flood barriers and create more sustainable solutions. This approach not only contributes to infrastructure development but also potentially generates additional employment opportunities. Despite the low probability of catastrophic flooding, the potential impact remains high, posing a threat to existing infrastructure systems. We argue that governments should take responsibility for rebuilding and maintaining infrastructure after such catastrophes because government resources are limited and carry a high opportunity cost when considering different societal investment categories. Our research suggests the introduction of innovative insurance-linked financial instruments, like resilience bonds, which offer a more cost-effective capital solution for insurers and more affordable insurance options for policyholders. Beyond financial benefits, these bonds provide non-monetary incentives to investors, such as recognition for supporting sustainable initiatives, enhancing reputation, or contributing to vital social and environmental goals. Given the crucial role of infrastructure in the lives of billions, leveraging private finance to support infrastructure investment can open new avenues for building resilience. This approach aligns with the broader SDG objectives, integrating sustainability into the core of infrastructure development and financing.
9. Conclusion
This paper primarily emphasised to establish the mechanism and a valuation model for resilience bonds, which serve the purpose of hedging the flood risks and support the resilient flood barrier enhancement projects through its unique functions. The research findings yielded several noteworthy conclusions, which contribute significantly to the field. The design of the flood resilience bond presents a promising opportunity to attract a broader investor base from the capital market. Its structure has the potential to bridge the gap between the capital market and insurance market, creating avenues for effective public-private partnerships in future investments for resilient infrastructure projects. Through our simulations, we have observed that the breaching of different flood barriers can result in unforeseen losses for the town. This highlights the urgent need for flood barriers enhancement projects to reinforce the resilience of the existing defense system and safeguard local coastal residents. By modelling the expected losses incurred from breaches and incorporating the benefits of resilience enhancement projects, we provide vital information to coastal decision-makers. This information assists them in understanding the opportunity cost associated with long-term financial gains achieved through proactive preparedness. Such insights empower decision-makers to make informed choices and prioritise investments that enhance the community's resilience in the face of future flood events.
In our pricing model for a five-year flood resilience bond for Towyn, we compared catastrophe bonds to resilience bond prices under the highest threshold of approximately 28 million, and have calculated that a 50% resilience from a resilient project will generate a 0.67% reduction in the returns by switching from CAT bond to resilience bond. We verify that risks associated with a resilience bond are lower than the catastrophe bond and the resilience bond offers additional returns for investment. However, since the threshold is considered as one of the significant influential factors to the price and yield for both bonds, the yield of resilience bond is 6.06% and the yield of catastrophe bond is 15.63% under the threshold of about 14 million. In this situation, a 50% resilience effect from the resilient project will generate a 6.75% difference in the returns as the benefit of switching from catastrophe bond investment to resilience bond investment. From the sensitivity analysis, we found that threshold, dynamic interest rate, and performance of resilient projects are the main influential factors upon prices of the flood resilience bond. A higher resilience level provided from the resilient project will increase the price of the bond, which leads to lower returns for bond investors, due to the fact that the risk of losing the principal has been reduced. In addition, from the test of different interest rate models, the flood resilience bond shows the characteristic that the price will rise along with the increasing of trading time, because less occurrences of trigger events during longer trading period will lower the probability of economic losses and reduce the risks. Thus, resilience bonds emerge as a critical tool, supporting the objectives of financial viability, risk management, and sustainable development, marking a significant progress towards a more resilient and sustainable future.
Disclosure statement
No potential conflict of interest was reported by the author(s).
References
- Bates, P.D., R.J. Dawson, J.W. Hall, M.S. Horritt, R.J. Nicholls, J. Wicks, and M.A.A.M. Hassan. 2005. Simplified two-dimensional numerical modelling of coastal flooding and example applications. Coastal Engineering 52: 793–810.
- Batica, J., and P. Gourbesville. 2016. Resilience in flood risk management – a new communication tool. Procedia Engineering 154: 811–7.
- Bennett, O., and S. Hartwell-Naguib. 2014. Flood defence spending in England. House of Commons Library Standard Note SN/SC/ 5755.
- Blackburn, M., J. Methven, and N. Roberts. 2008. Large-scale context for the UK floods in summer 2007. Weather 63: 280–8.
- Brand, M., K.Q. Seipp, P. Saksa, N. Ulibarri, A. Bomblies, L. Mandle, M. Allaire, O. Wing, J. Tobin-DE La Puente, and E. Parker. 2021. Environmental impact bonds: A common framework and looking ahead. Environmental Research: Infrastructure and Sustainability 1: 023001.
- Brigo, D., and F. Mercurio. 2007. Interest rate models-theory and practice: With smile, inflation and credit. Berlin: Springer Science & Business Media.
- Brockway, D. 2020. Flooding: Looking back on the 1990 Towyn devastation. BBC News. https://www.bbc.co.uk/news/uk-wales-51630850.
- Brody, S.D., W.E. Highfield, M. Wilson, M.K. Lindell, and R. Blessing. 2017. Understanding the motivations of coastal residents to voluntarily purchase federal flood insurance. Journal of Risk Research 20: 760–75.
- Burnecki, K., J. Janczura, and R. Weron. 2011. Building loss models. In Statistical tools for finance and insurance, eds. P. Cizek, W. Härdle, and R. Weron, 293–328. Berlin: Springer.
- Burnecki, K., G. Kukla, and D. Taylor. 2011. Pricing of catastrophe bonds. In Statistical tools for finance and insurance, eds. P. Cizek, W. Härdle, and R. Weron, 371–91. Berlin: Springer.
- Chen, J., G. Liu, L. Yang, Q. Shao, and H. Wang. 2013. Pricing and simulation for extreme flood catastrophe bonds. Water Resources Management 27: 3713–25.
- Clapp, C., and K. Pillay. 2017. Green bonds and climate finance. In Climate finance: Theory and practice, Series on the economics of climate change climate finance, 79–105. World Scientific.
- Dawson, R., J. Hall, and J. Davis. 2004. Performance-based management of flood defence systems. Proceedings of the Institution of Civil Engineers-Water Management 157: 35–44.
- Dawson, R.J., R. Peppe, and M. Wang. 2011. An agent-based model for risk-based flood incident management. Natural Hazards 59: 167–89.
- Holling, C.S. 1973. Resilience and stability of ecological systems. Annual Review of Ecology and Systematics 4: 1–23.
- Holling, C.S. 1996. Engineering resilience versus ecological resilience. Engineering Within Ecological Constraints 31: 32.
- Inderst, G. 2016. Infrastructure investment, private finance, and institutional investors: Asia from a global perspective.
- IPCC. 2019. Sea level rise and implications for low-lying islands, coasts and communities. Special Report on the Ocean and Cryosphere in a Changing Climate. [Chapter 4]. https://www.ipcc.ch/srocc/chapter/chapter-4/.
- Katori, T. 2018. The financial potential of green bonds: Comparing the three issuing schemes. Available at SSRN 3158890.
- Kemp, L., C. Xu, J. Depledge, K.L. Ebi, G. Gibbins, T.A. Kohler, J. Rockström, M. Scheffer, H.J. Schellnhuber, and W. Steffen. 2022. Climate endgame: Exploring catastrophic climate change scenarios. Proceedings of the National Academy of Sciences 119: e2108146119.
- Kendon, M., M. Mccarthy, S. Jevrejeva, A. Matthews, J. Williams, T. Sparks, and F. West. 2023. State of the UK climate 2022. International Journal of Climatology 43: 1–83.
- Larsen, G., G. Christianson, and N. Amerasinghe. 2019. So far, green bonds fail to raise much money for resilience. The climate resilience principles aim to change that. World Resources Institute. https://www.wri.org/blog/2019/10/so-far-green-bonds-fail-raise-much-money-resilience-climate-resilience-principles-aim.
- Ma, Z., C. Ma, and S. Xiao. 2017. Pricing zero-coupon catastrophe bonds using EVT with doubly stochastic Poisson arrivals. Discrete Dynamics in Nature and Society 2017: 3279647.
- Marijnissen, R., M. Kok, C. Kroeze, and J. Van Loon-Steensma. 2020. The sensitivity of a Dike-Marsh System to sea-level rise – a model-based exploration. Journal of Marine Science and Engineering 8: 42.
- Mendoza-Tinoco, D., D. Guan, Z. Zeng, Y. Xia, and A. Serrano. 2017. Flood footprint of the 2007 floods in the UK: The case of the Yorkshire and The Humber region. Journal of Cleaner Production 168: 655–67.
- Mizutori, M. 2019. Resilient infrastructure and disaster risk reduction. United Nations Office for Disaster Risk Reduction (UNDRR) and Centre for Research on the Epidemiology of Disasters (CRED) Report.
- MUNICH-RE. 2023. Natural disaster risks – rising trend in losses. https://www.munichre.com/en/risks/natural-disasters-losses-are-trending-upwards.html.
- Nowak, P., and M. Romaniuk. 2013. Pricing and simulations of catastrophe bonds. Insurance: Mathematics and Economics 52: 18–28.
- ONS (Office for National Statistics). 2023. Investment in flood defences, UK: May 2023. ONS Website.
- Polacek, A. 2018. Catastrophe bonds: A primer and retrospective. Chicago Fed Letter 405: 1–7.
- Proverbs, D., and J. Lamond. 2008. The barriers to resilient reinstatement of flood damaged homes. Paper presented at 4th International i-Rec Conference, April, Christchurch, New Zealand.
- Re:Focus. 2015. Leveraging catastrophe bonds: As a mechanism for resilient infrastructure project finance. RE bound insurance report.
- Re:Focus. 2017. A guide for public-sector resilience bond sponsorship. RE bound insuring for resilience.
- Sartzetakis, E.S. 2020. Green bonds as an instrument to finance low carbon transition. Economic Change and Restructuring 54: 755–79.
- Schelfaut, K., B. Pannemans, I. Van Der Craats, J. Krywkow, J. Mysiak, and J. Cools. 2011. Bringing flood resilience into practice: The FREEMAN project. Environmental Science & Policy 14: 825–33.
- Shao, J., A. Pantelous, and A.D. Papaioannou. 2015. Catastrophe risk bonds with applications to earthquakes. European Actuarial Journal 5: 113–38.
- Siemes, R.W., B.W. Borsje, R.J. Daggenvoorde, and S.J. Hulscher. 2020. Artificial structures steer morphological development of salt marshes: A model study. Journal of Marine Science and Engineering 8: 326.
- Stevens, A.J., D. Clarke, and R.J. Nicholls. 2016. Trends in reported flooding in the UK: 1884–2013. Hydrological Sciences Journal 61: 50–63.
- Tabari, H. 2020. Climate change impact on flood and extreme precipitation increases with water availability. Scientific Reports 10: 13768.
- Tellman, B., J.A. Sullivan, C. Kuhn, A.J. Kettner, C.S. Doyle, G.R. Brakenridge, T.A. Erickson, and D.A. Slayback. 2021. Satellite imaging reveals increased proportion of population exposed to floods. Nature 596: 80–86.
- Trotta, A. 2024. Environmental impact bonds: Review, challenges, and perspectives. Current Opinion in Environmental Sustainability 66: 101396.
- Tuhkanen, H. 2020. Green bonds: A mechanism for bridging the adaptation gap? Stockholm Environment Institute.
- United Nations. 2023. Sustainable transport. Department of Economic and Social Affairs – Sustainable Development.
- Vaijhala, S., and J. Rhodes. 2018. Resilience bonds: A business-model for resilient infrastructure. Field Actions Science Reports. The Journal of Field Actions 18: 58–63.
- Vajjhala, S. 2015. Financing infrastructure through resilience bonds. https://www.brookings.edu/blog/the-avenue/2015/12/16/financing-infrastructure-through-resilience-bonds/.
- Vamvakeridou-Lyroudia, L., A. Chen, M. Khoury, M. Gibson, A. Kostaridis, D. Stewart, M. Wood, S. Djordjevic, and D. Savic. 2020. Assessing and visualising hazard impacts to enhance the resilience of critical infrastructures to urban flooding. Science of the Total Environment 707: 136078.
- Wdowinski, S., R. Bray, B.P. Kirtman, and Z. Wu. 2016. Increasing flooding hazard in coastal communities due to rising sea level: Case study of Miami Beach, Florida. Ocean & Coastal Management 126: 1–8.
- Woodruff, J.D., J.L. Irish, and S.J. Camargo. 2013. Coastal flooding by tropical cyclones and sea-level rise. Nature 504: 44–52.
- Zimbidis, A.A., N.E. Frangos, and A.A. Pantelous. 2007. Modeling earthquake risk via extreme value theory and pricing the respective catastrophe bonds. ASTIN Bulletin: The Journal of the IAA 37: 163–83.