ABSTRACT
Aquatic fungi are a largely unexplored group of organisms with a still unknown diversity of ecological niches. Groundwater biomes comprise vast but poorly explored habitats. In this study, we sampled the Icelandic groundwater, a unique system that has been separated and isolated into distinct basins for millions of years due to volcanic activity. The aim was to explore the fungal diversity of the basins and its connection with the surface waters. We screened the DNA-based fungal diversity of 11 Icelandic groundwater springs and their emerging surface waters by ITS metabarcoding, revealing that the species turnover was indeed distinct for each groundwater basin. Furthermore, the groundwater taxa, which include many psychrophilic yeast-like and ascomycete fungi, seem to serve as a constant inoculum for the surface water. Nevertheless, the groundwater springs had a lower sequence proportion of early diverging, primary aquatic fungal lineages (10%) and completely unknown lineages (13%) than the surface waters (41% and 22%, respectively), likely explained by the lower diversity of suitable hosts for these presumed parasitic lineages. Our results highlight the importance of overlooked organism groups in the resolution of overarching research questions in ecology, nutrient circulation, and global change biology at large.
Introduction
Estimated at 2.2–3.8 million extant species, fungi form a large and diverse kingdom of heterotrophic eukaryotes (Hawksworth and Lücking Citation2017). While fungi are traditionally perceived as a primarily terrestrial group of organisms, some are known to colonize aquatic habitats such as rivers and streams where they, for instance, degrade plant debris (e.g., Graça et al. Citation2016, Tsui et al. Citation2016) or cycle nutrients as plankton parasites (Kagami et al. Citation2014). Recent studies revealed that the fungal diversity goes far beyond the amount usually attributed to aquatic systems (Grossart et al. Citation2019). As late as 2011, a new fungal phylum, the Rozellomycota (Cryptomycota), was described from freshwater bodies (Lara et al. Citation2010, Jones et al. Citation2011), and subsequent studies have added other large undescribed fungal lineages to the mycological palette (Comeau et al. Citation2016, Grossart et al. Citation2016, Tedersoo et al. Citation2017). Many of these groups are found at or close to the root of the fungal kingdom, providing an opportunity to glean important information on the evolution of fungi and their colonization of land. The ecology and functional guilds of these new groups of aquatic fungi are poorly described, and mycological studies in freshwaters are still comparably scarce, leaving freshwater habitats as largely uncharted territories in mycology (Grossart et al. Citation2019).
A first step toward a comprehensive knowledge body on aquatic fungal communities is DNA-based exploration of a diverse selection of aquatic systems around the globe. Such studies are slowly beginning to emerge (e.g., Le Calvez et al. Citation2009, Wurzbacher et al. Citation2016, Bochdansky et al. Citation2017). In this study, we focus on one such largely unexplored habitat: groundwater springs. This unseen though essential part of the freshwater biome comprises roughly one-third of the continental freshwaters (Gleick Citation1996). Groundwater is important as drinking water, and aquifers connect and replenish the surface waters. This connection poses a potential route of dispersal for groundwater microbes to surface waters and vice versa. Much like bacteria, fungal microbes in groundwater have been investigated with respect to drinking water quality, but these studies were mainly based on culture-dependent methods and focused largely on pathogenic fungi in technically processed tap water (e.g., Pereira et al. Citation2010, Babič et al. Citation2016). Rare exceptions have identified fungi as an important component in polluted (Brad et al. Citation2008) and natural aquifers (Livermore and Mattes Citation2013, Sohlberg et al. Citation2015, Nawaz et al. Citation2016, Citation2018). These culture-independent, DNA-based studies indicated the presence of groundwater-specific fungal communities whose composition did not match that of other terrestrial or aquatic habitats. We thus hypothesize that groundwater fungal communities differ both locally and globally.
We examined the communities in 11 freshwater springs (the groundwater–surface water boundary) along the volcanic zone of Iceland, a hitherto unstudied geographical region and aquifer type with respect to freshwater fungi. Located at the junction of the Arctic and the Atlantic oceans, Iceland is geographically isolated and presents a geologically young habitat as the country was covered by glaciers during the last glacial maximum about 20 000 years ago (Geirsdóttir et al. Citation2009). Iceland is located at a tectonic boundary along the Mid-Atlantic Ridge and reaches above sea level because of high volcanic activity. A volcanic zone stretches from southwest to northeast Iceland; the northwest zone is characterized by porous lava fields (mainly basalt) and fissures. The area is rich in surface freshwater habitats, and groundwater springs are maintained both by large glaciers and precipitation (Sveinbjörnsdóttir and Johnsen Citation1992). This zone differs from the other areas of Iceland in the east and northwest, which present older bedrock shaped by glaciers and characterised by runoff rivers. The discovery and taxonomic description of the endemic groundwater amphipods Crangonyx islandicus (Svavarsson and Kristjansson Citation2006) and Crymostygious thingvallensis (Kristjánsson and Svavarsson Citation2004) in lava springs in the volcanic zone provide evidence of a nearly unexplored groundwater ecosystem, which may have been isolated since the origin of the island around 25 million years ago (cf. Kornobis et al. Citation2010). Genetic analysis of the amphipod C. islandicus suggests that the groundwater systems from southern and northern Iceland have been isolated over periods of 0.5–4.7 million years (Kornobis et al. Citation2010). Because of geological activities, Iceland’s aquifers are influenced by geothermal heating, resulting in a natural temperature gradient from cold springs to the emergence of hot springs of up to 100 °C or higher.
The current biota of the groundwater entering the springs is largely unknown. Aside from studies on the groundwater amphipods, studies on ciliates (Gudmundsdóttir et al. Citation2018) and eDNA of bacteria and ciliates (R. Gudmundsdóttir and others, unpubl.) have revealed several additional species in this isolated habitat. Furthermore, the influence of surface or epigean organisms to this groundwater system may also vary because organisms and extracellular DNA may leach into the groundwater from the soil and the surrounding water. This process may be especially true for limnocrene (pool-forming) springs where the water may penetrate into the ecotone between the groundwater and the surface water. In rheocrene (stream-forming) springs, this admixture may be of less concern because of the currents.
The aim of this study was to assess the taxonomic composition of the fungal community in freshwater springs on Iceland. We analysed whether differences in fungal community composition exist between (a) groundwater discharging into the springs and the surface water, (b) the limnocrene and the rheocrene springs, (c) the different areas of the volcanic zone, and (d) environmental factors, such as pH, temperature, and elevation.
Materials and methods
Sampling
We sampled 11 springs with distinct origins during summer 2015 (). The springs were located in 4 different areas within Iceland—denoted southwest (SW), southeast (SE), north (N), and northeast (NE)— characterized by distinct mtDNA lineages of the groundwater amphipod C. islandicus (Kornobis et al. Citation2010). Six of the sampling sites belong to the limnocrene spring type and 5 to the rheocrene. The springs vary in elevation from 6 to 430 m a.s.l. At each sampling location, the water temperature, oxygen content, and pH were measured with a multi-probe device (Hydrolab DS5, Hach Hydromet, Loveland, CO, USA; ).
Figure 1. Sampling sites of groundwater springs in Iceland. The volcanic zone is marked in dark grey; glaciers are illustrated in light grey. The sampling sites are displayed as letters and are abbreviated according to .
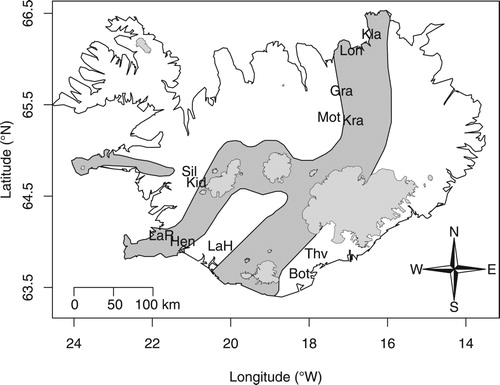
Table 1. Sampling sites and environmental parameters of the study sites in Iceland.
For DNA retrieval, 5 L of water was sampled from 2 locations in each spring. One was obtained by inserting a hose with an attached manual pump into the spring source and pumping the upwelling groundwater into sampling containers, or, when sources were too narrow to insert the hose, by collecting the upwelling water directly at the source opening. The second sample was collected by scooping up water from the free water column above the stream/pond bottom 2–3 m downstream of the source. To avoid cross-contamination, sampling containers were cleaned with HCl between sites and rinsed several times with spring water at each sampling site before the actual water sample was collected. The hose and pump were likewise thoroughly rinsed with clean water before and after each use. The collected water was vacuum-filtered through a DNA filter (Millipore Sterivex-GV Sterile Vented Filter Unit, 0.22 µm; Merck, Darmstadt, Germany) within 24 h after sampling. Filters were stored at −20 °C until further processing.
DNA extraction and PCR
Fungal diversity was evaluated by metabarcoding, targeting the 5.8S-ITS2 region (Schoch et al. Citation2012). Sample recovery and DNA extraction followed the protocol of Neufeld et al. (Citation2007), using the phenol:chloroform extraction method. DNA was diluted to a concentration of 0.3 ng/µL and amplified with fusion primers targeting fungi (ITS3-Mix2: Tedersoo et al. Citation2015; ITS4-cwmix1, ITS4-cwmix2: Wurzbacher et al. Citation2017) containing a 5’ adapter for the index polymerase chain reaction (PCR) (described later). PCR was carried out in 25 µL volumes and contained 5 µL DNA, 5 µL buffer, 2 µL MgCl2, 0.5 µL BSA (20 mg/mL), 0.5 µL ITS3-Mix2 (10 μM), 0.25 µL ITS4-cwmix1 (10 µM), 0.25 µL ITS4-cwmix2 (10 μM), 0.5 µL dNTPs (10 mM), and 0.25 µL Phusion Taq Polymerase (Thermo Fisher, Waltham, MA, USA). Amplification was performed using the following program: initial denaturation at 98 °C for 30 s, then 35 cycles of 10 s at 98 °C, 30 s at 55 °C, and 30 s at 72 °C, followed by final extension for 5 min at 72 °C, and then held at 4 °C. PCR amplifications were confirmed by 1% agarose gel electrophoresis. PCR failed repeatedly for 2 samples, and thus they were omitted from the DNA sequencing step.
DNA sequencing
Library preparation for sequencing followed the recommendation of “16S Metagenomic Sequencing Library Preparation” (Part#15044223Rev.B; Illumina, San Diego, CA, USA). Briefly, the initial 20 PCR products were barcoded by a second 8-cycle index PCR, introducing forward and reverse Illumina barcodes. The resulting PCR products were then pooled in an equimolar way (after estimating the DNA concentrations on a Bioanalyzer DNA7500 chip, Hong Kong), diluted, denatured, and sequenced on a MiSeq benchtop sequencer (Illumina) with the v3 chemistry that resulted in a forward and reverse read of ∼300 nt each.
Sequence data processing
Sequencing data were processed as outlined in Heeger et al. (Citation2019). Briefly, the forward and reverse reads were sorted (Flexbar 2.5), trimmed (Trimmomatic 0.36, with settings: SLIDINGWINDOW:8:20 TRAILING:20, MINLEN:200, AVGQUAL:30; Bolger et al. Citation2014), and merged (Pear 0.9.8, default with a merged read length range of 150–565 nt; Zhang et al. Citation2014). The ITS2 region was extracted (ITSx 1.1.2, with the activated option to save all regions: save_regions; Bengtsson-Palme et al. Citation2013), clustered (Swarm 2.1.8, default settings; Mahé et al. Citation2015), and classified using a lowest common ancestor (LCA) classification following Heeger et al. (Citation2019). We conservatively removed all resulting classified operational taxonomic units (OTUs) with <5 reads. Sequence data were deposited in the European Nucleotide Archive (ENA; www.ebi.ac.uk/ena) under the project accession number PRJEB34808.
Classification of sequences at the fungal root
One problem that occurs when analysing ITS sequences of aquatic fungi is a massive taxonomic under-representation in reference databases (Frenken et al. Citation2017, Khomich et al. Citation2018). Because of the high variability of the ITS1 and ITS2, this drawback often results in no close hits against any sequence in the ITS reference database UNITE (Kõljalg et al. Citation2013). To avoid this problem in our dataset, for which we expect a high percentage of early diverging fungal lineages, we used a complementary LCA classification of the 5.8S part of our sequences, as outlined in Heeger et al. (Citation2019). After this, only OTUs classified as fungi were retained. In addition, we considered a contrasting classification of 5.8S and ITS2 elements (discussed later) as putative artefacts and removed these from the OTU table.
Ecological annotation of the most prevalent OTUs
To assign the most probable ecology to the dominant fungal sequences, we manually examined a representative sequence from each of the fungal OTUs using BLAST (Altschul et al. Citation1997) in GenBank (Clark et al. Citation2016) and UNITE. In this process, we followed the sequence analysis guidelines by Nilsson et al. (Citation2012) and Hyde et al. (Citation2013), while also referring to the BLAST results provided by the 5.8S part of the sequence, to inspect and annotate the OTUs, whenever applicable, as yeast/yeast-like, aquatic, or as a potential parasite.
Statistical analyses
The sequence abundance across samples was on average 7962 fungal sequences. To reduce potential effects caused by too widely varying read abundances, we removed all samples with <500 fungal sequences or <2000 total reads (3 samples, ; Supplement S1) and randomly resampled all samples with >10 k reads (3 samples) to 8 k reads (based on an average of 100 subsamplings using rrarefy of the R package vegan 2.5-3; Oksanen et al. Citation2017). Only alpha diversity indices (Shannon and Shannon’s evenness) were calculated based on random subsamplings to 586 reads. Also, the sample LaH was excluded because of a potential sampling bottleneck that resulted in only 4 fungal OTUs. The final OTU matrix of 16 samples went into the multivariate statistical analysis. The fungal community composition of the samples was compared by computing a nonmetric multidimensional scaling (NMDS) based on ranked orders of a Bray-Curtis dissimilarity matrix and is thus less sensitive to variation in distances than ordinary multidimensional scale or principal coordinate analyses. Samples in the NMDS plots were identified by location, area, and spring and sample type. Differences among sites were tested statistically using the PERMANOVA-like adonis function in the vegan R-package (Oksanen et al. Citation2017) considering sample types as nested within springs using the strata option. Variation in alpha diversity was analysed by ANOVA. All analyses and calculations were conducted in R 3.3.2 (R core team). Results were visualized using the R package ampvis2 2.4 (https://madsalbertsen.github.io/ampvis2/).
Results
Fungal community composition
We obtained 835 fungal OTUs. The recovery of fungal sequences differed between source and surface water samples with a median proportion of 0.52 and 0.14 fungal reads, respectively. The Shannon diversity varied among areas and was on average higher for the source (3.18 [standard error: SE 0.20]) than the surface samples (2.94 [0.18]; ). In addition, a significant interaction was observed between area and spring type (). The fungal community composition, tested with adonis, differed significantly between the sample types, spring type, and area (, ). The effect of the environmental variables (temperature, elevation, pH, and oxygen concentrations) was not significant in our study.
Figure 2. Ordination of fungal OTU composition in freshwater samples from the volcanic zone in Iceland. LaH excluded. The 2 panels present the grouping by the 2 sample types (a) sample type (source: filled circles; surface: open circles) and (b) spring type (limnocrene: filled circles; rheocrene: open circles). Information for the spring labels in (a) are given in . SW (southwest), SE (southeast), N (north), and NE (northeast). The stress of the multidimensional scaling was 0.19.
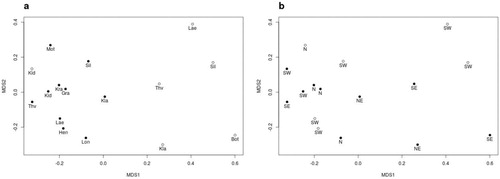
Table 2. Sequencing summary and variation at the sampling sites in Iceland – statistics summarized after rarefaction.
Table 3. Analysis of variance using Shannon diversity index. LaH sample excluded.
Table 4. Analysis of variation of species compositions among samples. LaH sample excluded. Significance was estimated based on 1000 permutations, tested with adonis in the vegan R-package.
Taxonomy of fungal DNA sequences
We classified 72% of the OTUs to phylum level, 46% to class level, 32% to family level, 32% to genus level, and 23% to species level (see final detailed composition of fungal sequences in Supplement S1). Overall, both the surface water and the source water fungal community differed in their phylum distribution and dominance. Notably the relative read abundances of typical freshwater phyla (Chytridiomycota and Rozellomycota) were higher in the surface waters (41% vs. 13%, including Zygomycota; ). The same pattern could be found for the unknown lineages (22% and 10%, respectively). This distribution was consistent when summarizing the presence–absence of taxa, confirming a dominance of diverse freshwater lineages (50%) in the surface water over the source water (12%). Both water sources shared dominant OTUs, but differences in the distribution of shared OTUs existed between surface waters (62%) and source samples (22%, ). In particular, the most dominant 49 OTUs (100%) of the surface waters were also shared by the source samples while 35% of the 49 dominant source water OTUs were unique to source water samples, indicating a directed transition of source water taxa into the surface waters. Most prevalent taxa in the Icelandic source and connected surface water samples from the 4 tested geographic areas were affiliated with aquatic lineages, yeasts, or unknown fungi (). Residual taxa may belong to plant or arthropod parasites (e.g., Lecanicillium, Stagonospora, Leptosphaeriaceae, and Microdochium).
Figure 3. Heatmap of the most abundant fungi grouped by water source and geography. Left: OTU grouping by phylum between surface waters and groundwater samples, summarizing the respective relative read abundances (read %) and adjunct the relative taxa abundance (taxa %, based on presence/absence of OTU). Right: list of the most dominant OTUs on species level grouped by water type and geographic area (based on relative read abundance). Blue text demarks aquatic taxa, orange unknown fungi, and filled circles mark yeasts/yeast-like fungi. (Color version can be viewed online.)
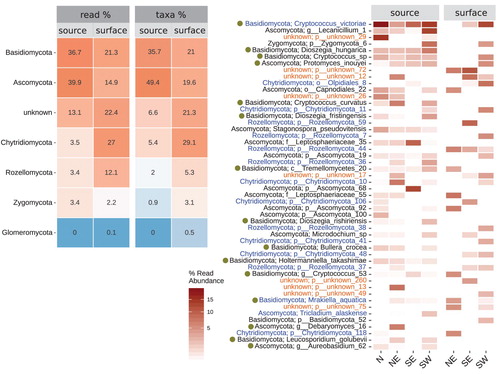
Figure 4. Venn diagram of the sum of shared species across all samples between source and surface water samples shows the shared and unique fungal taxa for each water sample type (source and surface water samples). The diagram is based on the rarefied OTU table. The taxa from the original OTU table are shown in parentheses.
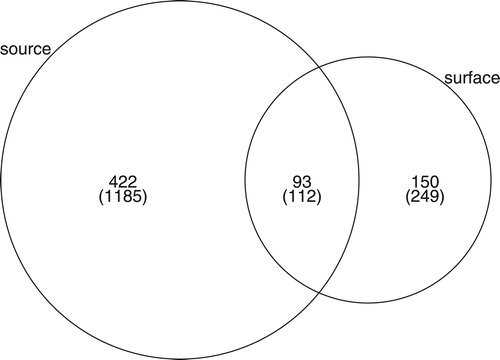
Discussion
The geographic isolation and the volcanic separation of the Icelandic groundwater systems renders Iceland a unique site for research on parallel evolving groundwater biota, such as the amphipods (Kornobis et al. Citation2010, Gudmundsdóttir et al. Citation2018). Although the knowledge on spatial groundwater microbiota evolution is fairly limited (Griebler and Lueders Citation2009), the Icelandic groundwater microbiota likely underwent a divergence similar to that of the amphipods, assuming that dispersal among watershed basins is limited. To obtain a first characterization of the fungal mycobiota of Iceland’s water sources, we performed a pioneering study through sampling the different hydrologically separated areas of Iceland. Although sample size was limited, our study indicates possible distinct geographical fungal communities in each area. While the type of groundwater spring seems to exert additional influence on the fungal community (limnocrene vs. rheocrene), environmental factors showed no effect in our study, which might relate to our narrow environmental variation. In our study, the variation of measured environmental factors was mainly attributable to elevation and pH, whereas oxygen concentration and water temperature were (with single exceptions) fairly constant, as expected for groundwater springs. While the emerging groundwater of the 11 springs had a larger number of OTUs and differed significantly from the surface waters in their fungal community, they shared many of the dominant groundwater OTUs, indicating that groundwater may serve as a constant fungal inoculum for the Icelandic surface waters. In our study, we gained a first indication of distinct groundwater communities in Iceland, offering promising research perspectives on spatially evolving groundwater microbial communities (Griebler and Lueders Citation2009).
One major aim of this study was to expand our knowledge of aquatic fungi by targeting fungal communities from hitherto unsampled inland waters of Iceland. We were explicitly interested in the fungal taxa found in freshwater springs in the groundwater basins of the volcanic zone along the Mid-Atlantic Ridge. Aerobic freshwater springs have rarely been sampled for fungi, and only limited knowledge exists on the fungal communities of these particular groundwater–surface water boundaries (to our knowledge only examined by Pereira et al. Citation2010). In this study, we showed that the emerging groundwater from the springs was dominated by Dikarya fungi, in accordance with previous findings from groundwater studies (Sohlberg et al. Citation2015, Babič et al. Citation2016, Nawaz et al. Citation2016, Citation2018, Perkins et al. Citation2019), followed by lower percentages of early diverging lineages, which matched the reported value for Rozellomycota from an aquifer in the United States (Livermore and Mattes Citation2013). A noteworthy similarity between our results and previous studies is the high proportion of yeasts and yeast-like fungi, which have been frequently isolated and identified in groundwater, with Cryptococcus as one of the dominant genera (Ekendahl et al. Citation2003, Sohlberg et al. Citation2015, Babič et al. Citation2016, Nawaz et al. Citation2016). Interestingly, our most dominant OTU was classified as Cryptococcus victoriae (average of 13%; recently combined to Vishniacozyma victoriae), a species previously described as a psychrophilic yeast from glacial polar biomes (de Garcia et al. Citation2012). In fact, some of the most abundant OTUs in our list were described as psychrophilic (Dioszegia species, Phenoliferia psychrophila, Thelebolus microsporus, Aureobasidium subglaciale, and Tricladium alaskense; Supplement S1). This adaptation to a cold climate might be rather specific to the Icelandic groundwater springs and could possibly be explained by the large glacier masses that cover the island and serve as freshwater reservoir for groundwater recharge (Sveinbjörnsdóttir and Johnsen Citation1992).
Regarding the transition of groundwater to surface water, we noted that (a) the dominant spring water OTUs persisted in the adjacent surface water and (b) there was a major turnover toward typical aquatic parasites of the early diverging lineages Rozellomycota and Chytridiomycota. This observation is comparable to other studies of surface waters (e.g., Monchy et al. Citation2011, Wurzbacher et al. Citation2016) and is possibly explained by a greater richness of suitable hosts (photoautotrophs and subsequent taxa from derived trophic levels) than in the groundwater. Also noteworthy is an increase in the relative abundance of unknown taxa from 13% in the groundwater to 22% in the surface waters. The inability to classify these further may be explained by a lack of reference data, in particular for the early diverging lineages (Frenken et al. Citation2017, Heeger et al. Citation2018). These unknown taxa also appear in other surface water and groundwater studies (Sohlberg et al. Citation2015, Nawaz et al. Citation2016) and were recently identified as an actively transcribing fungal component of the groundwater mycobiome (Nawaz et al. Citation2018).
In conclusion, the fungi we recovered from the Icelandic inland waters and freshwater springs largely derive from aquatic and yeast lineages we know little about. They belong in parts of the fungal tree where the phylogenetic resolution is poor, which also means we are left pondering the ecology and function of many of these fungi in freshwater springs and groundwater. How do they obtain their energy? What organisms do they interact with? These questions and others are important as we gradually expand our knowledge on these fungi. These fungi are also important from the perspective of ecosystem services, landscape ecology, and global change biology. The Icelandic freshwater systems comprise a set of small and remote inland waters that give rise to substantial, pressing, and global scientific questions.
Supplemental Material
Download Zip (369.8 KB)Acknowledgements
We thank Viggó Þ. Marteinsson for providing laboratory space and equipment at Matís (Reykjavík) and Stephen Knobloch for invaluable help during sample recovery, PCRs, and sequencing. SVdW was supported by a IGB Postdoc Fellowship.
Disclosure statement
No potential conflict of interest was reported by the authors.
Additional information
Funding
References
- Altschul SF, Madden TL, Schäffer AA, Zhang J, Zhang Z, Miller W, Lipman DJ. 1997. Gapped BLAST and PSI-BLAST: a new generation of protein database search programs. Nucleic Acids Res. 25:3389–3402. doi: 10.1093/nar/25.17.3389
- Babič MN, Zalar P, Ženko B, Džeroski S, Gunde-Cimerman N. 2016. Yeasts and yeast-like fungi in tap water and groundwater, and their transmission to household appliances. Fungal Ecol. 20:30–39. doi: 10.1016/j.funeco.2015.10.001
- Bengtsson-Palme J, Ryberg M, Hartmann M, Branco S, Wang Z, Godhe A, De Wit P, Sánchez-García M, Ebersberger I, de Sousa F, et al. 2013. Improved software detection and extraction of ITS1 and ITS2 from ribosomal ITS sequences of fungi and other eukaryotes for analysis of environmental sequencing data. Methods Ecol Evol. 4:914–919.
- Bochdansky AB, Clouse MA, Herndl GJ. 2017. Eukaryotic microbes, principally fungi and labyrinthulomycetes, dominate biomass on bathypelagic marine snow. ISME J. 11:362–373. doi: 10.1038/ismej.2016.113
- Bolger AM, Lohse M, Usadel B. 2014. Trimmomatic: a flexible trimmer for Illumina sequence data. Bioinformatics. 30:2114–2120. doi: 10.1093/bioinformatics/btu170
- Brad T, Braster M, van Breukelen BM, van Straalen NM, Röling WF. 2008. Eukaryotic diversity in an anaerobic aquifer polluted with landfill leachate. Appl Environ Microbiol. 74:3959–3968. doi: 10.1128/AEM.02820-07
- Clark K, Karsch-Mizrachi I, Lipman DJ, Ostell J, Sayers EW. 2016. Genbank. Nucleic Acids Res. 44:D67–D72. doi: 10.1093/nar/gkv1276
- Comeau AM, Vincent WF, Bernier L, Lovejoy C. 2016. Novel chytrid lineages dominate fungal sequences in diverse marine and freshwater habitats. Sci Rep. 6:30120. doi: 10.1038/srep30120
- de Garcia V, Zalar P, Brizzio S, Gunde-Cimerman N, van Broock M. 2012. Cryptococcus species (Tremellales) from glacial biomes in the Southern (Patagonia) and Northern (Svalbard) hemispheres. FEMS Microbiol Ecol. 82:523–539. doi: 10.1111/j.1574-6941.2012.01465.x
- Ekendahl S, O’Neill AH, Thomsson E, Pedersen K. 2003. Characterisation of yeasts isolated from deep igneous rock aquifers of the Fennoscandian Shield. Microb Ecol. 46:416–428. doi: 10.1007/s00248-003-2008-5
- Frenken T, Alacid E, Berger SA, Bourne EC, Gerphagnon M, Grossart HP, Gsell AS, Ibelings BW, Kagami M, Küpper FC, et al. 2017. Integrating chytrid fungal parasites into plankton ecology: research gaps and needs. Environ Microbiol. 19:3802–3822. doi: 10.1111/1462-2920.13827
- Gleick PH. 1996. Water resources. In: Schneider SH, editor. Encyclopedia of climate and weather. Oxford (UK): Oxford University Press; p. 817–823.
- Geirsdóttir Á, Miller GH, Axford Y, Ólafsdóttir S. 2009. Holocene and latest Pleistocene climate and glacier fluctuations in Iceland. Quat Sci Rev. 28:2107–2118. doi: 10.1016/j.quascirev.2009.03.013
- Graça MA, Hyde K, Chauvet E. 2016. Aquatic hyphomycetes and litter decomposition in tropical–subtropical low order streams. Fungal Ecol. 19:182–189. doi: 10.1016/j.funeco.2015.08.001
- Griebler C, Lueders T. 2009. Microbial biodiversity in groundwater ecosystems. Freshw Biol. 54:649–677. doi: 10.1111/j.1365-2427.2008.02013.x
- Grossart HP, Van den Wyngaert S, Kagami M, Wurzbacher C, Cunliffe M, Rojas-Jimenez K. 2019. Fungi in aquatic ecosystems. Nat Rev Microbiol. 17:339–354. doi: 10.1038/s41579-019-0175-8
- Grossart HP, Wurzbacher C, James TY, Kagami M. 2016. Discovery of dark matter fungi in aquatic ecosystems demands a reappraisal of the phylogeny and ecology of zoosporic fungi. Fungal Ecol. 19:28–38. doi: 10.1016/j.funeco.2015.06.004
- Gudmundsdóttir R, Kornobis E, Kristjánsson BK, Pálsson S. 2018. Genetic analysis of ciliates living on the groundwater amphipod Crangonyx islandicus (Amphipoda: Crangonyctidae). Acta Zool. 99:188–198. doi: 10.1111/azo.12204
- Hawksworth D, Lücking R. 2017. Fungal diversity revisited: 2.2 to 3.8 million species. In: Heitman J, Howlett B, Crous P, Stukenbrock E, James T, Gow N, editors. The fungal kingdom. Washington (DC): ASM Press; p. 79–95.
- Heeger F, Bourne EC, Baschien C, Yurkov A, Bunk B, Spröer C, Overmann J, Mazzoni CJ, Monaghan MT. 2018. Long-read DNA metabarcoding of ribosomal RNA in the analysis of fungi from aquatic environments. Mol Ecol Resour. 18:1500–1514. doi: 10.1111/1755-0998.12937
- Heeger F, Wurzbacher C, Bourne EC, Mazzoni CJ, Monaghan MT. 2019. Combining the 5.8S and ITS2 to improve classification of fungi. Methods Ecol Evol. 10:1702–1711. doi: 10.1111/2041-210X.13266
- Hyde KD, Udayanga D, Manamgoda DS, Tedersoo L, Larsson E, Abarenkov K, Bertrand YJK, Oxelman B, Hartmann M, Kauserud H, et al. 2013. Incorporating molecular data in fungal systematics: a guide for aspiring researchers. Curr Res Environ Appl Mycol J Fungal Biol. 3:1–32. doi: 10.5943/cream/3/1/1
- Jones MDM, Forn I, Gadelha C, Egan MJ, Bass D, Massana R, Richards TA. 2011. Discovery of novel intermediate forms redefines the fungal tree of life. Nature. 474:200–203. doi: 10.1038/nature09984
- Kagami M, Miki T, Takimoto G. 2014. Mycoloop: chytrids in aquatic food webs. Front Microbiol. 5:166. doi: 10.3389/fmicb.2014.00166
- Khomich M, Cox F, Andrew CJ, Andersen T, Kauserud H, Davey ML. 2018. Coming up short: identifying substrate and geographic biases in fungal sequence databases. Fungal Ecol. 36:75–80. doi: 10.1016/j.funeco.2018.08.002
- Kõljalg U, Nilsson RH, Abarenkov K, Tedersoo L, Taylor AF, Bahram M, Bates ST, Bengtsson-Palme J, Callaghan TM, Douglas B, et al. 2013. Towards a unified paradigm for sequence-based identification of fungi. Mol Ecol. 22:5271–5277. doi: 10.1111/mec.12481
- Kornobis E, Pálsson S, Kristjansson BK, Svavarsson J. 2010. Molecular evidence of the survival of subterranean amphipods (Arthropoda) during Ice Age underneath glaciers in Iceland. Mol Ecol. 19:2516–2530.
- Kristjánsson BK, Svavarsson J. 2004. Crymostygidae, a new family of subterranean freshwater gammaridean amphipods (Crustacea) recorded from subarctic Europe. J Nat Hist. 38:1881–1894. doi: 10.1080/00222930310001597295
- Lara E, Moreira D, López-García P. 2010. The environmental clade LKM11 and Rozella form the deepest branching clade of fungi. Protist. 161:116–121. doi: 10.1016/j.protis.2009.06.005
- Le Calvez T, Burgaud G, Mahé S, Barbier G, Vandenkoornhuyse P. 2009. Fungal diversity in deep-sea hydrothermal ecosystems. Appl Environ Microbiol. 75:6415–6421. doi: 10.1128/AEM.00653-09
- Livermore JA, Mattes TE. 2013. Phylogenetic detection of novel Cryptomycota in an Iowa (United States) aquifer and from previously collected marine and freshwater targeted high-throughput sequencing sets. Environ Microbiol. 15:2333–2341. doi: 10.1111/1462-2920.12106
- Mahé F, Rognes T, Quince C, De Vargas C, Dunthorn M. 2015. Swarm v2: highly-scalable and high-resolution amplicon clustering. PeerJ. 3:e1420. doi: 10.7717/peerj.1420
- Monchy S, Sanciu G, Jobard M, Rasconi S, Gerphagnon M, Chabé M, Cian A, Meloni D, Niquil N, Christaki U, et al. 2011. Exploring and quantifying fungal diversity in freshwater lake ecosystems using rDNA cloning/sequencing and SSU tag pyrosequencing. Environ Microbiol. 13:1433–1453. doi: 10.1111/j.1462-2920.2011.02444.x
- Nawaz A, Purahong W, Lehmann R, Herrmann M, Küsel K, Totsche KU, Buscot F, Wubet T. 2016. Superimposed pristine limestone aquifers with marked hydrochemical differences exhibit distinct fungal communities. Front Microbiol. 7:666. doi: 10.3389/fmicb.2016.00666
- Nawaz A, Purahong W, Lehmann R, Herrmann M, Totsche KU, Küsel K, Wubet T, Buscot F. 2018. First insights into the living groundwater mycobiome of the terrestrial biogeosphere. Water Res. 145:50–61. doi: 10.1016/j.watres.2018.07.067
- Neufeld JD, Schafer H, Cox MJ, Boden R, McDonald IR, Murrell JC. 2007. Stable-isotope probing implicates Methylophaga spp. and novel Gammaproteobacteria in marine methanol and methylamine metabolism. ISME J. 1:480–491. doi: 10.1038/ismej.2007.65
- Nilsson RH, Tedersoo L, Abarenkov K, Ryberg M, Kristiansson E, Hartmann M, Schoch CL, Nylander JA, Bergsten J, Porter TM, et al. 2012. Five simple guidelines for establishing basic authenticity and reliability of newly generated fungal ITS sequences. MycoKeys. 4:37–63. doi: 10.3897/mycokeys.4.3606
- Oksanen J, Blanchet FG, Friendly M, Kindt R, Legendre P, McGlinn D, Minchin PR, O’Hara RB, Simpson GL. 2017. Solymos P. vegan: community ecology package. R Package Version. 2:4–4.
- Pereira VJ, Fernandes D, Carvalho G, Benoliel MJ, San Romão MV, Crespo MB. 2010. Assessment of the presence and dynamics of fungi in drinking water sources using cultural and molecular methods. Water Res. 44:4850–4859. doi: 10.1016/j.watres.2010.07.018
- Perkins A, Ganzert L, Rojas-Jimenez K, Fonvielle J, Hose G, Grossart HP. 2019. Highly diverse fungal communities in carbon-rich aquifers of two contrasting lakes in Northeast Germany. Fungal Ecol. 41:116–125. doi: 10.1016/j.funeco.2019.04.004
- Schoch CL, Seifert KA, Huhndorf S, Robert V, Spouge JL, Levesque CA, Chen W, Fungal Barcoding Consortium. 2012. Nuclear ribosomal internal transcribed spacer (ITS) region as a universal DNA barcode marker for fungi. Proc Natl Acad Sci USA. 109:6241–6246. doi: 10.1073/pnas.1117018109
- Sohlberg E, Bomberg M, Miettinen H, Nyyssönen M, Salavirta H, Vikman M, Itävaara M. 2015. Revealing the unexplored fungal communities in deep groundwater of crystalline bedrock fracture zones in Olkiluoto, Finland. Front Microbiol. 6:573. doi: 10.3389/fmicb.2015.00573
- Svavarsson J, Kristjansson BK. 2006. Crangonyx islandicus sp. nov., a subterranean freshwater amphipod (Crustacea, Amphipoda, Crangonyctidae) from springs in lava fields in Iceland. Zootaxa. 1365:1–7. doi: 10.11646/zootaxa.1365.1.1
- Sveinbjörnsdóttir ÁE, Johnsen SJ. 1992. Stable isotope study of the Thingvallavatn area. Groundwater origin, age and evaporation models. Oikos. 64:136–150. doi: 10.2307/3545049
- Tedersoo L, Anslan S, Bahram M, Põlme S, Riit T, Liiv I, Kõljalg U, Kisand V, Nilsson RH, Hildebrand F, et al. 2015. Shotgun metagenomes and multiple primer pair-barcode combinations of amplicons reveal biases in metabarcoding analyses of fungi. MycoKeys. 10:1–43. doi: 10.3897/mycokeys.10.4852
- Tedersoo L, Bahram M, Puusepp R, Nilsson RH, James TY. 2017. Novel soil-inhabiting clades fill gaps in the fungal tree of life. Microbiome. 5:42. doi: 10.1186/s40168-017-0259-5
- Tsui CK, Baschien C, Goh TK. 2016. Biology and ecology of freshwater fungi. In: Li DW, editor. Biology of microfungi: fungal biology. Cham (Switzerland): Springer; p. 285–313.
- Wurzbacher C, Nilsson RH, Rautio M, Peura S. 2017. Poorly known microbial taxa dominate the microbiome of permafrost thaw ponds. ISME J. 11:1938–1941. doi: 10.1038/ismej.2017.54
- Wurzbacher C, Warthmann N, Bourne EC, Attermeyer K, Allgaier M, Powell JR, Detering H, Mbedi S, Grossart HP, Monaghan MT. 2016. High habitat-specificity in fungal communities in oligo-mesotrophic, temperate Lake Stechlin (North-East Germany). MycoKeys. 16:17–44. doi: 10.3897/mycokeys.16.9646
- Zhang J, Kobert K, Flouri T, Stamatakis A. 2014. PEAR: a fast and accurate Illumina Paired-End reAd mergeR. Bioinformatics. 30:614–620. doi: 10.1093/bioinformatics/btt593