ABSTRACT
Coordinating multiple tasks requires a high degree of cognitive control, and individuals with limited executive functions often show difficulties in everyday multitasking. We tested the hypothesis that demands on executive control can be alleviated by internally representing the temporal pattern of goals and deadlines as spatial relations. In two experiments, participants completed a multitasking session by monitoring deadlines of four clocks running at different rates, along with separate tasks of executive functioning and spatial ability. In Experiment 1, individual and gender-related differences in spatial ability (mental rotation) predicted multitasking performance, beyond the contributions of both the updating and inhibition components of executive functioning, and even when spatial cues were eliminated from the layout of the monitoring task. Experiment 2 extended these findings by showing that concurrent spatial load impaired task monitoring accuracy, and that these detrimental effects were accentuated when spatial abilities were compromized due to fluctuation in female sex hormones. These findings suggest that multiple task monitoring involves working memory-related functions, but that these cognitive control demands can be offloaded by relying on spatial relation processes.
Demands on handling multiple tasks within limited time frames have increased during the last decades, partly reflecting more recent developments of the digital society. How people schedule and coordinate multiple goals and deadlines in the context of everyday activities is a topic of considerable theoretical and practical interest (e.g. Burgess, Veitch, De Lacy Costello, & Shallice, Citation2000; Logie, Trawley, & Law, Citation2011; Meyer & Kieras, Citation1997; Navon & Gopher, Citation1979; Redick et al., Citation2016; Salvucci & Taatgen, Citation2008; Wickens, Citation2002).
A large body of the research referred to as “multitasking” is based on dual-task paradigms, where pinpointing different levels of interference such as cognitive bottlenecks (Pashler, Citation1994) and crosstalk between sensory input and active processing mechanisms is of essence (Janczyk, Renas, & Durst, Citation2017). Rather than focusing on cognitive bottlenecks between conflicting tasks, the primary focus of this study was to understand how we avoid these bottlenecks by scheduling and interleaving deadlines and other task constraints in order to achieve multiple goals end states (Janczyk et al., Citation2017; Logie et al., Citation2011). These simulations of everyday multitasking differ from typical dual-task paradigms both in terms of number of component tasks and overall duration of the multitasking scenario. Compared to monitoring and scheduling multiple ongoing activities, demands for temporal coordination are very low in most dual-task conditions in that they do not require coordination of complex patterns of temporal contingencies.
A key aspect is that most goal-directed tasks, including multiple-task performance, are of temporal nature, meaning that scheduling, monitoring and task interleaving take place on a future-oriented time scale. As a consequence, coordinating multiple goals and patterns of deadlines requires a high degree of cognitive control. Several lines of research have shown that individuals with limited cognitive control functions, due to, for example, delayed development, brain damage, pathology or aging, have major difficulties in handling and coordinating multiple tasks and intentions within limited time frames (Burgess et al., Citation2000; Craik & Bialystok, Citation2006; Mackinlay, Charman, & Karmiloff-Smith, Citation2006; Shallice & Burgess, Citation1991). On the other hand, highly trained experts in diverse fields can monitor and coordinate multiple tasks with seemingly limited effort (Chisholm, Dornfeld, Nelson, & Cordell, Citation2001; Loukopoulos, Dismukes, & Barshi, Citation2009; Wickens, Citation2008), supporting the idea that these control demands of complex task coordination can be offloaded by relying on overlearned action scripts, schemas, and related knowledge structures.
However, not only experts, but all cognitive agents can alter the information processing requirements of a task in order to reduce its control demands (see Risko & Gilbert, Citation2016, for an overview). In many everyday situations, these executive control demands are handled and alleviated by overlearned knowledge structures domain-specific skills (i.e. implicit memory and semantic memory, Burgess et al., Citation2000; Fuster, Citation2001; Norman & Shallice, Citation1986; Salvucci, Citation2005). Experts on the other hand, such as air-traffic controllers, are better multitaskers than non-experts due to their domain-specific scripts and knowledge structures that allow them to offload cognitive control demands (Nunes & Kramer, Citation2009). Similarly, older adults with reduced cognitive resources rely more on schematic knowledge when compared to younger adults with better executive control functions, validating the idea that experience leads to reliance on learned tactics as a way of reducing cognitive demands (Peters, Hess, Västfjäll, & Auman, Citation2007).
Cognitive science studies have shown that humans can change the task affordances by performing “epistemic” (Kirsh & Maglio, Citation1994) or physical actions (Goldinger, Papesh, Barnhart, Hansen, & Hout, Citation2016; Wilson & Golonka, Citation2013) and by relying both on internal and external representations of the task (Zhang & Norman, Citation1994). Extending this view, we propose a complementary mechanism for alleviating control demands in multitasking performance.
Our proposal was inspired by the notion of embodied cognition (e.g. Clark, Citation2010; Hutchins Citation1995, 2010; Risko, Medimorec, Chisholm, & Kingstone, Citation2014; Wilson, Citation2002). In this view, cognition includes actions and strategies for offloading cognitive demands, and its central goal is to understand how we use our body and the world in the course of cognitive activities (see also Risko & Gilbert, Citation2016, for a review). However, cognitive offloading does not only involve embodied and situated actions (such as head tilts to align task representations, e.g. Risko et al., Citation2014), but also internal processes of transformation and normalisation. We reasoned that coordinating temporal patterns of multiple ongoing activities is cognitively demanding but that these control demands might be reduced by representing patterns of temporal deadlines to spatial relations. Consistent with this line of reasoning, research on several domains of cognitive sciences, including linquistics and neurosciences, suggest that we use space to represent temporal relations (see e.g. Bonato, Zorzi, & Umiltà, Citation2012; Casasanto & Boroditsky, Citation2008; Dehaene & Brannon, Citation2011; Gijssels, Bottini, Rueschemeyer, & Casasanto, Citation2013; Núñez & Cooperrider, Citation2013; Stocker, Citation2014; Walsh, Citation2003).
As a direct support for this spatial offloading hypothesis, we have reported several studies (with Swedish and Italian participants) showing that spatial ability and executive functioning are independent predictors of multitasking performance, and that spatial ability predicts multitasking over and beyond executive functioning (Mäntylä, Citation2013; Mäntylä, Coni, Kubik, Todorov, & Del Missier, Citation2017; Todorov, Del Missier, & Mäntylä, Citation2014; Todorov, Del Missier, Konke, & Mäntylä, Citation2015).
For example, Mäntylä (Citation2013) reported a study in which individuals with varying spatial skills completed a multitasking session and separate tasks of executive functioning (working memory updating) and spatial ability (mental rotation). Specifically, participants completed a time-based multitasking session with four identical component tasks, requiring a high degree of time coordination among the tasks. In this counter task, participants monitored four digital “clocks” (or counters) and were instructed to respond whenever one of the counters showed a specific deadline. Individual differences in executive functioning (working memory updating) and spatial ability (mental rotation) were shown to be independent pre- dictors of multiple-task monitoring but only spatial ability was related to sex differences in multitasking. Furthermore, and consistent with the offloading hypothesis, multitasking performance reflected sex hormone-related fluctuations in spatial ability. Contrary to popular beliefs (Fisher, Citation1999; Pease & Pease, Citation2003, in which females are expected to outperform males, (see also Stoet, O’Connor, Conner, & Laws, Citation2013; Szameitat, Lepsien, Von Cramon, Sterr, & Schubert, Citation2006), Mäntylä (Citation2013) found the opposite pattern. Males showed better performance than females, and sex differences were fully mediated by spatial ability. Furthermore, hormonal variation moderated these effects in that gender differences in spatial ability and multitasking (but not in executive functioning) were observed between males and females in the luteal phase (during which the level of estradiol is elevated), but not for females in the menstrual phase of the cycle.
The aim of the current study was to extend the generality of the spatial offloading hypothesis by contrasting it with two alternative accounts (Experiment 1) and by testing its central prediction through an experimental manipulation (Experiment 2). A reasonable alternative account for the incremental effects of spatial ability on multitasking is that these effects reflect task-specific characteristics, rather than more general cognitive functions. Specifically, in many everyday situations of multitasking, the component tasks are spatially defined with distinct positions (e.g. on the kitchen sink when preparing a dinner or on a computer screen when handling different programmes or sources of media). Similarly, in our counter task, the digital clocks are presented on different positions of the computer screen. As spatial information is a distinct characteristic of the component tasks (in both everyday multitasking and experimental simulations), one might argue that that the observed role of spatial ability in our earlier work was induced by the spatial nature of the tasks per se.
This alternative hypothesis is supported by the findings of Morgan et al. (Citation2013) who related measures of working memory and mental rotation to varying difficulty of multitasking in a pilot simulation task. Consistent with the spatial offloading hypothesis, both working memory and spatial ability conributed to multitasking performance, and the role of spatial ability was accentuated (and that of working memory was reduced) when task difficulty increased. However, Morgan et al. (Citation2013) attributed these effects to task-specific effects in that certain aspects of the pilot simulation environment might draw on spatial abilities, thereby supporting the alternative hypothesis formulated here.
To explicitly test this alternative account, participants of Experiment 1 completed either the original version of the counter task (i.e. physically separated component tasks, here referred to as the spatial-counter task) or a modified version, in which we eliminated its spatial characteristics by modifying the graphic layout of the counters. The spatial and nonspatial versions were virtually identical, except that in the latter task, the four counters were overlaid on top of each other and appeared on the same position of the screen. To the extent that the spatial nature of the counter task was relevant, individual differences in spatial ability should contribute to multitasking performance only in the spatial version of the task. Alternatively, to the extent that reliance on internal “space in time” transformational processes is a more general offloading strategy of complex task coordination, individuals with effective spatial abilities should perform better than less skilled individuals in both tasks.
Another aim of Experiment 1 was to test the hypothesis that spatial ability contributes to multiple-task performance beyond executive functioning because only its updating component was considered in our previous studies (see also Todorov et al., Citation2015). Considering the central role of updating in cognitive control, including its close association with both working memory and fluid intelligence (e g., Shipstead, Harrison, & Engle, Citation2016), the updating component of executive functioning should be the best predictor of individual differences in multitasking. Consistent with this reasoning, Mäntylä and colleagues obtained support for the incremental role of spatial ability in multitasking by using tasks that are considered to tap the updating component of executive functioning. Yet, inconsistent with the offloading hypothesis, these effects might reflect the fact that only its updating component was considered in these studies. A related concern is that as many tests of executive functioning are associated with measurement problems, it has been suggested that its assessment should be based on multiple measures (cf. Miyake et al., Citation2000).
To address these concerns, participants of Experiment 1 completed a multitasking session along with two separate measures of response inhibition (stop-signal and Stroop tasks) and working-memory updating (letter-memory and matrix-monitoring tasks). As the two components are considered as independent, but correlated, dimensions of cognitive control (Miyake et al., Citation2000), or tapping a more general vs. a more specific dimension of control (Miyake & Friedman, Citation2012), we hypothesised that both measures would contribute to individual differences in multitasking performance. Indeed, inhibition processes may be generally relevant to goal-directed performance, supporting selective actions and to eliminate irrelevant or no more relevant information in sequential processing (e.g. Carretti, Cornoldi, DeBeni, & Roman, Citation2005). In contrast, updating processes are expected to be crucial in time-based performance, such as in event monitoring, when temporal deadlines need to be met (Mäntylä, Carelli, & Forman, Citation2007). Furthermore, following the spatial offloading hypothesis, we expected that individual differences in spatial ability would incrementally contribute to multitasking performance even when both the updating and inhibition components were considered.
In Experiment 2, we examined the implications of the spatial offloading hypothesis experimentally, testing its predictions by manipulating concurrent task demands on spatial processing. We reasoned that to the extent that spatial processing contributes to coordination of multiple deadlines, then dynamically reconfiguring the spatial layout of component tasks (cf. changing the position of tools on a work bench) should have detrimental effects on multitasking performance and these detrimental effects should be accentuated in individuals with less compromised spatial skills.
Experiment 1
Method
Participants
A total of 93 undergraduates (60 women) between 18 and 35 years of age (Mage = 24.2 years) participated in the study in return for partial course credit. Based on our earlier work, an a priori power analysis for the spatial vs. nonspatial contrast provided an estimate of N = 70 for a .80 power (two tailed). To better meet the standards for the application of regression analysis (e.g. Tabachnick & Fidell, Citation2001), we increased the sample size of the spatial-counter group by including an additional sample of 23 participants.
Tasks and procedure
Multitasking was assessed with the counter task. In this time-based monitoring task, four digital clocks, or counters, were occluded by coloured rectangles on the computer screen (see also Mäntylä, Citation2013). Participants could monitor each counter by pressing a specific key, whereupon the corresponding counter appeared for 2 s. To prevent the four tasks from being handled as a unitary task, the counters ran at different rates. Participants pressed spacebar whenever one of the counters displayed a target reading defined by a simple rule. Participants were instructed to press spacebar when the last digit of the Green Counter (running at 5.7s/item) was 7, when the last two digits of the Blue Counter (running at 3.9s/item) were a multiple of 11, when the last two digits of the Red Counter (running at 2.3s/item) were a multiple of 20, and when the last two digits of the Yellow Counter (running at 1.9s/item) were a multiple of 25. The response rules and the task rates were calibrated so that the number of deadlines was approximately the same (12–14 targets/counter). Participants could check the reading of each counter whenever they wanted by pressing a same-colored key on the keyboard (see also ). In the spatial version of the counter task, the four component tasks appeared on separate positions of the computer screen, occluded by coloured rectangles (a)). In the nonspatial version (b), a grey rectangle appeared in the middle of the screen, indicating the position of the counters, which were defined by coloured frames.
Figure 1. A schematic illustration of the counter task, with (a) coloured occluders and response keys of the spatial version (Experiment 1) and the no-load condition (Experiment 2), b shows the grey overlay of the four counters in the nonspatial version of Experiment 1. In the load-conditions of Experiment 2 (c), the counters switched positions every 4 min (medium load) or 3 min (high load), while the gray response keys were consistently mapped.
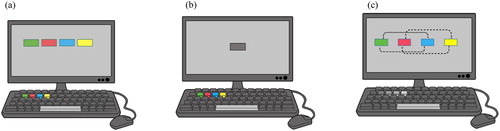
Executive functioning was assessed with the letter-memory and matrix-monitoring tasks, which are considered to tap the updating component, and response inhibition was measured with the stop-signal and Stroop tasks. In each trial of the letter–memory task (Miyake et al., Citation2000; Morris & Jones, Citation1990), letters are sequentially presented on the screen, with the length of the series varying randomly. At the end of the sequence, participants are asked to report the last three letters. The final score is the proportion of final letters correctly recalled across 48 trials.
In the matrix-monitoring task (Salthouse, Atkinson, & Berish, Citation2003), two 4 × 4 matrices appeared on the computer screen, separated by a line. A black dot then appeared in one of the cells in both matrices for 2.5 s. After a 500-ms ISI, two sequences of three arrows appeared for 1.2 s (separated by a 250-ms ISI) to indicate the movement of the dot in either the upper or the lower matrix. Finally, one matrix reappeared either above or below the line, with the dot in one of the cells. Participants decided whether the position of the dot was the same as or different from the final position indicated by the arrows. The main task comprised 12 trials, and the number of correct responses was the dependent measure.
In the Stroop task (Stroop, Citation1935), a set of three words is presented on the screen, with the central word being in colour. We used a computerised version of this task, requiring manual responses on 96 trials (see Del Missier, Mäntylä, & Bruin, Citation2012; Mäntylä, Del Missier, & Nilsson, Citation2009). In half the trials, the colour is congruent with the word (e.g. the word “red” is printed in red), whereas in the other half, it is incongruent (e.g. the word “red” is printed in blue). The two lateral words to the left and right on the screen are colour names that are always printed in black. Participants are instructed to press a right-side key to indicate that the colour of the central word corresponds to the colour name on the right side of the screen, whereas they have to press a left-side key to indicate that the colour of the central word corresponds to the colour name on the left side. The dependent measures were number of correct responses and the difference in response time (RT) between congruent and incongruent trials.
In each trial of the stop-signal task (Logan, Citation1994; Salthouse et al., Citation2003), a stimulus letter (“X” or “O”) appears briefly on the computer screen. Participants are instructed to press a specific key, except for when they hear a “beep” (or a stop signal) after the stimulus presentation. The task comprised 48 trials, of which 12 trials were associated with a stop signal (presented between 200 to 400 ms after each stimulus letter). Stop signal RT and response accuracy were the dependent measures (see also Mäntylä et al., Citation2007; Miyake et al., Citation2000).
Spatial ability was assessed with the Vandenberg and Kuse Mental Rotations Test (MRT; Peters et al., Citation1995; Vandenberg & Kuse, Citation1978). After receiving written instructions and completing four practice problems, participants were given 3 min to complete Set A, followed by a short break after which they completed Set C. The dependent measure of this task was number of correct responses. Responses were scored such that participants were given a point only if both stimulus figures that matched the target figure were identified correctly (max = 24 for both sets).
During the test session, which took about one hour, each individually tested participant completed first a background questionnaire, followed by the cognitive tasks of Stroop, MRT, letter memory, counter, stop-signal, and matrix-monitoring. As typical for individual-differences studies (cf. Wilhelm, Hildebrandt, & Oberauer, Citation2013), the task order was the same for all participants. Except for the MRT, all the tasks were computerised, and the stimuli were presented on a 20” display. Each task included separate instructions and a practice phase during which the experimenter confirmed that instructions were properly understood. Informed consent was obtained before participation, and the study was completed according to the ethical guidelines established by the Declaration of Helsinki.
Results
Multitasking performance was based on a combined score of the four counter tasks (see also Todorov et al., Citation2015), with response accuracy and monitoring frequency as dependent measures. A response was considered correct if the spacebar was pressed within 1 digit of the target (e.g. the digits of 19, 20, and 21 would be considered correct responses if the target was 20).
The executive functioning data were submitted to principal components analyses (with varimax rotation), separately for the RT and accuracy data. These analyses showed virtually identical two-component solutions, with the two inhibition tasks constituting Factor 1, and the updating tasks Factor 2. These two component explained 71% (accuracy) and 63% (RT) of the total variance. In the following analyses, each participant's factor scores were used as measures of executive functioning, and we report the accuracy data as they explained a somewhat larger proportion of the total variance than the RT data.
As shown in , the spatial and nonspatial versions of the counter task showed highly similar results, measured in terms of counter task accuracy and monitoring frequency. The two groups of participants were also similar in terms of spatial ability and executive functioning, suggesting that the difference in spatial arrangement of the counters did not have any reliable effects. Consequently, the spatial and nonspatial data were combined in the following analyses.
Table 1. Task performance across group and gender in Experiment 1, with standard deviations in parenthesis.
Consistent with our earlier work, the counter-task data showed a significant gender difference, with males outperforming females in response accuracy, F(1, 91) = 4.06, MSe = .01, p < .05. Furthermore, this effect was not related to gender difference in monitoring frequency in that males and females monitored the readings of the four counters at the same rate (F = 1.11, p < .30). As shown in , males were somewhat more accurate in MRT than females, but this difference was not significant (F = 2.86, p < .10). However, when MRT was included as a covariate, the gender difference in response accuracy was eliminated, suggesting that spatial ability mediated this effect. As expected, no gender differences were observed in executive functioning (Fs < 1).
In the second step of analysis, we examined the relation between multitasking performance and the measures of updating, inhibition and MRT, respectively. The correlation data, summarised in , suggest that multitasking performance, measured in terms of response accuracy, was related to individual differences in both spatial ability and executive functioning. Furthermore, both measures of executive functioning correlated with counter-task accuracy, although the updating index showed a somewhat stronger association than the inhibition index. These data also indicate that counter-task accuracy and frequency were associated, but that neither executive functioning nor spatial ability correlated with monitoring frequency.
Table 2. pearson correlations for multitasking, spatial ability, and components of executive functioning in experiment 1, separately for counter-task accuracy (upper diagonal) and counter-task monitoring frequency (lower diagonal).
Finally, we conducted a hierarchical regression analysis, entering the updating and inhibition indices first, followed by MRT. Results showed that both updating (β = 0.33, p < .01, and inhibition (β = 0.20, p < .05 were significant predictors, explaining 16% of the accuracy variance. More importantly, after controlling for the two compound measures of executive functioning, MRT remained a reliable predictor of counter-task accuracy (β = 0.39, p < .01), explaining an additional 15% of variance, R = .56, F change (3,87) = 12.93, SE = .08, p < .01.
To take explicitly into account the potential influence of the relation between updating and inhibition components of executive functioning, we also estimated (via maximum likelihood) a path-analysis model in which updating, inhibition, and spatial ability were independent predictors of multitasking performance, while considering the positive correlation between updating and inhibition in the model. The results showed that all three predictors contributed significantly to multitasking performance (R2 = .31), with spatial ability (standardised coefficient = .38, p < .001) contributing somewhat more than updating (standardised coefficient = .32, p < .001) and inhibition (standardised coefficient = .19, p < .05), while updating and inhibition were significantly related (standardised coefficient = .21, p < .05). Overall, the model had an excellent level of fit, Chi-square = .515, df = 2, p = .773, Chi-square/df = 2 .773, CFI = 1.000, RMSEA = 0.000, NCP = 0.000, AIC = 24.515.
Experiment 2
The results of Experiment 1 provided correlational evidence for the hypothesis that succesful multitasking involves executive functioning, and spatial abilities. In Experiment 2, we aimed to extend these findings by experimentally testing the hypothesis that concurrent spatial processing should have detrimental effects on multitasking. To this end, we induced varying levels of spatial load by dynamically reconfiguring the spatiotemporal pattern of the deadlines of the four component tasks. Specifically, we repeatedly re-arranged the task layout by switching the positions of the four counters on the screen. Participants initially learned and followed a fixed monitoring sequence, based on the spatiotemporal arrangement of the four clocks and their deadlines (e.g. first A then B, followed by C and D, after which the same pattern of deadlines is repeated during the task session). In the no-load condition, participants completed the whole session by following the same task order (e.g. A-B-C-D). In two spatial load conditions, this initial layout was changed after a given time (e.g. C-B-A-D), and participants needed to reconfigure the spatiotemporal order of deadlines. In the medium load condition the spatial arrangement of the four component tasks changed every four minutes, and in the high load condition, it changed every three minutes.
Following the spatial offloading hypothesis, we expected better performance in the no-load condition than in the spatial-load conditions. More important, we expected that participants with better spatial skills would show better multitasking performance than less skilled participants, and that these effects would be accentuated when spatial load was increased. Furthermore, and related to our earlier work (Mäntylä, Citation2013; Todorov et al., Citation2015; see also Halpern, Citation2012; Hampson & Morley, Citation2013; Hampson, Levy-Cooperman, & Korman, Citation2014; Miller & Halpern, Citation2014), we expected that these effects would interact with sex-hormone fluctuations in that females in the luteal phase of the menstrual cycle (during which the level of circulating estradiol is heightened and mental-rotation skills reduced) would have been more sensitive to the load manipulation than males and females in the menstrual phase of the cycle (during which gender differences in mental rotation are typically eliminated).
Method
Participants
A total of 185 university undergraduates (83 males) between 19 and 47 years of age (Mage = 25.6, SD = 5.5) participated in the study in return for partial course credit or a movie voucher. About half of the female participants completed the test session while being in the menstrual phase, and the remaining females were in the luteal phase of their menstrual cycle. Estimates of menstrual phase were based on self-reports, and both groups were defined as 2–3 days before or after the target phase (see also Mäntylä, Citation2013; Todorov et al., Citation2015). Individuals, who were under hormonal treatment, used hormonal contraceptives, or were pregnant were not included in the study. Excessive computer gamers (>1.5 h/day) were also excluded from the study.
Tasks and procedure
In the no-load condition, participants completed the same (spatial) version of the counter task as in Experiment 1. The load conditions were virtually identical to that of the no-load condition, except that the positions of the counters on the computer screen changed every 4 min (medium load) or every 3 min (high load) during a 20-min test interval. Furthermore, the responses keys were coloured in the no-low condition but appeared in different grey shades in the load conditions (see ). To avoid response-mapping confusion, the response keys always mapped the positions of the counters appearing on the screen with the first (#1) key from left referring to the first counter from left on the screen etc.
Each participant was tested individually during a 30-min session. After informed consent was obtained, participants first completed a brief questionnaire about demographic background and computer gaming habits. Female participants also responded to a few questions about menstrual cycle and use of hormonal substances. Then participants received written instructions for the counter task, followed by practice trials for 60 s. After the experimenter had confirmed that participants had understod the instructions, they completed the multitasking session for 20 min.
To confirm that differences in female participants’ menstrual phase actually reflected differences in spatial ability, a subset of participants in each group (N = 122) completed the MRT at the final stage of the test session. These data showed consistent group differences in MRT, F(2, 119) = 18.89, MSe = 0.023, p < .01, in that males (M = .47, SD = .17) outperformed the menstrual (M = .36, SD = .14) and luteal (M = .27, SD = .14) groups, including a significant difference between the two female groups (all ps < .05).
Results
As in Experiment 1, multitasking performance was based on a combined score of the four counter tasks, and responses were considered as correct if they were within 1 digit of the target. As the menstrual phase of one female participant could not be determined due to an unclear self-report, her data were excluded from the analysis. Furthermore, three participants showed exceptionally low rates of counter-task accuracy (<3 SD), and also their data were excluded from the final data set (N = 184).
A 3 (Task type) × 3 (Group) ANOVA showed significant main effects of task type, F(2, 184) = 9.94, MSe = 0.163, p < .01. Post hoc analyses confirmed that performance in the no-load condition (M = .82, SD = .12) was more accurate than in the medium-load (M = .78, SD = .17, p < .05) and high-load (M = .72, SD = .15, p < .01) conditions (with a nonsignificant difference between these two). Also the main effect of group was significant, F(2, 184) = 29.22, MSe = 0.478, p < .01, with reliable contrasts among the means of males (M = .86, SD = .10), females in the menstrual (M = .78, SD = .11) and luteal (M = .68, SD = .18) groups (all ps < .01).
Furthermore, as shown in , these group differences interacted with spatial load, F(4, 174) = 3.22, MSe = 0.053, p < .01, and post-hoc analyses indicated that females in the luteal phase (with less effective spatial skills) were more sensitive to the spatial-load manipulation than the other two groups, with a significant decline between the no load and medium load conditions (p < .01). The menstrual group and males were not affected by the medium load (vs. no-load) manipulation, but the accuracy of both groups declined significantly in the high load condition (ps < .01), in which males (with most effective spatial skills) outperformed both the luteal group (p < .01) and menstrual group (p < .05).
General discussion
The aim of this study was to test the hypothesis that multiple task performance is determined by individual differences in executive functions, but that these control demands can be alleviated by representing complex temporal relations in terms of spatial relations. In Experiment 1, we examined this hypothesis by relating multitasking performance, as measured by the counter task, to two central components of executive functioning, each measured with multiple tasks and to a measure of spatial ability. Another central aim of Experiment 1 was to test the validity of the spatial offloading hypothesis of multitasking while reducing effects of task-specific spatial cues embedded into the multitasking session.
Supporting the hypothesis, the results of Experiment 1 showed that individual differences in executive functioning and spatial ability were independent predictors of multiple-task performance. First, we demonstrated that both the inhibition and updating components of executive functioning were independent predictors of counter-task accuracy, suggesting that multiple task performance reflects both common and more specific mechanism of executive functioning.
Following the more recent view, inhibition reflects a central aspect of a common cognitive-control dimension, namely, the ability to suppress no longer relevant information, while updating is a more specific executive component (Miyake & Friedman, Citation2012). From this perspective, our findings suggest that effective multitasking and handling of multiple deadlines requires both the ability to keep attention focused on the target stimuli at the right time, suppressing irrelevant information, and the ability to keep track of the passage of time and events in order to update the tasks representations and meet the appropriate deadlines.
A second main finding of Experiment 1 was that spatial ability incrementally contributed to multitasking performance, even when the contribution of both components of executive functioning was considered. This result provides additional support for the spatial offloading hypothesis, and suggests that spatial ability contributes to multiple-task performance beyond executive functioning even when both the inhibition and updating components are considered (see also Todorov et al., Citation2015).
Another central finding was that these effects were observed even when the spatial characteristics of the counter task were eliminated. The spatial and nonspatial versions showed virtually identical overall levels of performance and highly similar patterns of correlations with the cognitive reference measures. These results suggest that “space in time” transformational processes reflect a more general offloading strategy of complex-task coordination, rather than being mediated by the spatial nature of the tasks per se (cf. Morgan et al., Citation2013).
Related to these findings, Todorov et al. (Citation2015) found that the effect of spatial ability on multitasking performance was not counter-task specific in that they observed a similar pattern of results in a highly realistic work-place simulation of multitasking. Although the task environment is rather complex and realistic, performance in this Simultaneous Capacity/Multitasking simulation (SIMKAP, Bratfisch & Hagman, Citation2003) correlated significantly with both counter-task accuracy and spatial ability, including sex hormone-related effects.
Although these findings, along with related work in other domains of cognitive sciences, support the hypothesis that spatial relation processing contribute to temporal coordinationg of multiple tasks, the exact nature of these transformational processes is not clear. Spatial ability as measured by the MRT, predicts individua and gender-related differences multitasking performance, beyond the independent contribution of executive functioning. These transformational processes may reflect specific spatial relation processes (see Todorov et al., Citation2015 for selective effects coordinate vs. categorical spatial processing in multitasking), although they also might indicate even more abstract neurocognitive mechanisms of magnitude processing (e.g. Walsh, Citation2003).
The results of Experiment 1 showed that spatial ability predicted counter-task accuracy over and beyond multiple measures of executive functioning, while task-specific spatial information was minimised. An implication of these findings is that multitasking performance should be compromised when the possibility of relying on spatial processing is reduced or eliminated. In other words, to the extent that special relations are used to alleviate cognitive control of temporal relations, multiple-task performance should be harmed when access to spatial relation processing is constrained. In Experiment 2, we tested this hypothesis by dynamically rearranging the spatial layout of task components, and found that these reconfigurations of the task layout had detrimental effects on multitasking performance, especially when the spatial load was high. Furthermore, these effects were accentuated in females in the luteal phase of the menstrual cycle.
These findings provide causal evidence for the spatial offloading hypothesis, suggesting that reliance on spatial relation processes provides a functional strategy for alleviating cognitive control demands in complex task conditions. Even if one might argue that that our load manipulation of changing task configurations actually increased demands on more general (working-memory related) cognitive functions, rather than spatial processing, and that these findings do not provide direct support for the spatial offloading hypothesis. This alternative account is reasonable but weakened by the fact that the multitasking performance of the three groups reflected expected group differences in spatial ability. Specifically, compared to males and females in the menstrual phase, females in the luteal phase of the menstrual cycle showed reduced ability in mental rotation (and multitasking, Mäntylä, Citation2013; Todorov et al., Citation2015), with minimal differences in executive functioning. These findings were expected and consistent with earlier work (e.g., Halpern, Citation2012; Hampson et al., Citation2014; Hampson & Morley, Citation2013; Maki, Rich, & Shayna Rosenbaum, Citation2002; Miller & Halpern, Citation2014). Thus, the three groups showed consistent effects of spatial load on their multitasking performance, while our own and others earlier studies reliably suggest that sex hormone-related effects on executive functioning are minimal.
It should be noted that, in Experiment 2, we tested the offloading hypothesis by manipulating task-specific (intrinsic) spatial configurations (cf. changing the arrangement of objects on a workspace), rather than inducing task-independent (extrinsic) spatial distraction (cf. handling objects on a workspace while completing additional spatial tasks). We recently reported a study (Mäntylä et al., Citation2017), in which participants completed a similar multitasking session, while making concurrent spatial judgments. We expected and found concurrent task costs, suggesting that possibilities for spatial offloading were reduced when the coordination of temporal deadlines was completed under concurrent spatial task. Furthermore, these effects were selective with greater concurrent-task costs on coordinate (metric) than categorical (nonmetric) spatial relation processing (see also Kosslyn, Citation1987; Laeng, Chabris, & Kosslyn, Citation2003; Todorov et al., Citation2015). Taken together, these intrinsic and extrinsic manipulations of spatial load provide consistent causal evidence for the notion that, not only executive functioning, but also spatial relation processing contributes to multiple-task performance.
Although the findings of this study are consistent with our spatial offloading hypothesis of multitasking, certain limitations should be acknowledged. First, a central assumption of our framework, which we have emphasised in earlier work (Mäntylä et al., Citation2017; Mäntylä & Todorov, Citation2013; Todorov et al., Citation2015), is that expertise and executive control functioning are the primary sources for individual differences in multitasking. Experts are better than nonexperts in handling multiple tasks (e.g. air traffic control, Loukopoulos et al., Citation2009; preparing a breakfast in one's own kitchen), and individuals with better executive functions are typically more effective multitaskers than individuals with less competent control functions (Redick et al., Citation2016; Shallice & Burgess, Citation1991). However, in many novel or complex situations, overlearned scripts and schematic knowledge structures are not relevant (or even interfering) for handling executively demanding task coordination (cf. preparing a breakfast in someone else's kitchen). In these conditions, recoding temporal patterns of deadlines to spatial relations may reduce executive control demands of multiple-task coordination.
Another boundary condition of this hypothesis is that multitasking is assumed to reflect individual differences in spatial ability only when demands on temporal coordination are high. Thus, the role of spatial ability should be reduced or even eliminated under flexible deadlines and time windows. Similarly, the contribution of spatial skills should be less prominent in dual-task performance, in which demands on temporal coordination are minimised. This hypothesis is also supported by studies, in which “multitasking” requires the coordination of two component tasks (cf. driving while talking to the phone, see also Mäntylä & Todorov, Citation2013; Strayer, Medeiros-Ward, & Watson, Citation2013).
To conclude, our findings are consistent with the proposal that complex goal-directed tasks, such as multitasking or memory for multiple intentions, require high degrees of cognitive control, but that successful performance in these tasks may also reflect the influence of other higher cognitive functions. In this spatial offloading hypothesis of multitasking, we suggest that executive control demands involved in temporal coordination of complex patterns of deadlines can be alleviated by transforming temporal relations into spatial relations, and that individuals with effective spatial abilities are better multitaskers than individuals with more limited spatial skills. An interesting avenue for future work would be to investigate the relation among cognitive control, working memory, spatial processing and related references measures of higher cognition, while systematically manipulating central characteristics of multitasking under theoretically driven boundary conditions.
Acknowledgement
Portions of this research were presented at the International Meeting of the Psychonomic Society 2016, Granada, Spain.
Disclosure statement
No potential conflict of interest was reported by the authors.
Additional information
Funding
References
- Bonato, M., Zorzi, M., & Umiltà, C. (2012). When time is space: Evidence for a mental time line. Neuroscience and Biobehavioral Reviews, 36, 2257–2273. doi: 10.1016/j.neubiorev.2012.08.007
- Bratfisch, O., & Hagman, E. (2003). Simultankapazität/Multi-Tasking (SIMKAP) Version 24.00: Handanweisung [Simultaneous capacity/multi-tasking (SIMKAP) release 24.00: Manual]. Mödling: Schuhfried.
- Burgess, P. W., Veitch, E., De Lacy Costello, A., & Shallice, T. (2000). The cognitive and neuroanatomical correlates of multitasking. Neuropsychologia, 38, 848–863. doi: 10.1016/S0028-3932(99)00134-7
- Carretti, B., Cornoldi, C., DeBeni, R., & Roman, M. (2005). Updating in working memory: A comparison of poor and good comprehenders. Journal of Experimental Child Psychology, 91, 45–66. doi: 10.1016/j.jecp.2005.01.005
- Casasanto, D., & Boroditsky, L. (2008). Time in the mind: Using space to think about time. Cognition, 106, 579–593. doi: 10.1016/j.cognition.2007.03.004
- Chisholm, C. D., Dornfeld, A. M., Nelson, D. R., & Cordell, W. H. (2001). Work interrupted: A comparison of workplace interruptions in emergency departments and primary care offices. Annals of Emergency Medicine, 38, 146–151. doi: 10.1067/mem.2001.115440
- Clark, A. (2010). Supersizing the mind. New York: Oxford University Press.
- Craik, F. I. M., & Bialystok, E. (2006). Planning and task management in older adults: Cooking breakfast. Memory & Cognition, 34, 1236–1249. doi: 10.3758/BF03193268
- Dehaene, S., & Brannon, E. M. (2011). Space, time and number in the brain: Searching for the foundations of mathematical thought. London: Elsevier.
- Del Missier, F., Mäntylä, T., & Bruin, W. B. (2012). Decision-making competence, executive functioning, and general cognitive abilities. Journal of Behavioral Decision Making, 25, 331–351. doi: 10.1002/bdm.731
- Fisher, H. (1999). The first sex: The natural talents of women and how they are changing the world. New York, NY: Random House.
- Fuster, J. M. (2001). The prefrontal cortex - an update: Time is of the essence. Neuron, 30, 319–333. doi: 10.1016/S0896-6273(01)00285-9
- Gijssels, T., Bottini, R., Rueschemeyer, S.-A., & Casasanto, D. (2013). Space and time in the parietal cortex: fMRI evidence for a neural asymmetry. Proceedings of the 35th Annual Meeting of the Cognitive Science Society (CogSci 2013), 4, 495–500.
- Goldinger, S. D., Papesh, M. H., Barnhart, A. S., Hansen, W. A., & Hout, M. C. (2016). The poverty of embodied cognition. Psychonomic Bulletin & Review, 23, 959–978. doi: 10.3758/s13423-015-0860-1
- Halpern, D. F. (2012). Sex differences in cognitive abilities (4th ed.). New York, NY: Psychology Press.
- Hampson, E., Levy-Cooperman, N., & Korman, J. M. (2014). Estradiol and mental rotation: Relation to dimensionality, difficulty, or angular disparity? Hormones and Behavior, 65, 238–248. doi: 10.1016/j.yhbeh.2013.12.016
- Hampson, E., & Morley, E. E. (2013). Estradiol concentrations and working memory performance in women of reproductive age. Psychoneuroendocrinology, 38, 2897–2904. doi: 10.1016/j.psyneuen.2013.07.020
- Hutchins, E. (1995). Cognition in the Wild. Cambridge: MIT Press.
- Janczyk, M., Renas, S., & Durst, M. (2017). Identifying the locus of compatibility-based backward crosstalk: Evidence from an extended PRP paradigm. Journal of Experimental Psychology : Human Perception and Performance, 49(0), 1–49.
- Kirsh, D., & Maglio, P. (1994). On distinguishing epistemic from pragmatic action. Cognitive Science, 18, 513–549. doi: 10.1207/s15516709cog1804_1
- Kosslyn, S. M. (1987). Seeing and imagining in the cerebral hemispheres: Computational approach. Psychological Review, 94, 148–175. doi: 10.1037/0033-295X.94.2.148
- Laeng, B., & Chabris, C. F. (2003). Asymmetries in encoding spatial relations. In K. Hugdahl & R. J. Davidson (Eds.), The asymmetrical brain (pp. 303–309). Cambridge, MA: MIT Press.
- Logan, G. D. (1994). On the ability to inhibit thought and action: A users’ guide to the stop signal paradigm. In D. Dagenbach, & T. H. Carr (Eds.), Inhibitory processes in attention, memory, and language (pp. 189–239). San Diego, CA: Academic Press.
- Logie, R. H., Trawley, S., & Law, A. S. (2011). Multitasking: Multiple, domain-specific cognitive functions in a virtual environment. Memory & Cognition, 39, 1561–1574. doi: 10.3758/s13421-011-0120-1
- Loukopoulos, L. D., Dismukes, R. K., & Barshi, I. (2009). The multitasking myth: Handling complexity in real-world operations. Farnham: Ashgate.
- Mackinlay, R., Charman, T., & Karmiloff-Smith, A. (2006). High functioning children with autism spectrum disorder: A novel test of multitasking. Brain and Cognition, 61(1), 14–24. doi: 10.1016/j.bandc.2005.12.006
- Maki, P. M., Rich, J. B., & Shayna Rosenbaum, R. (2002). Implicit memory varies across the menstrual cycle: Estrogen effects in young women. Neuropsychologia, 40, 518–529. doi: 10.1016/S0028-3932(01)00126-9
- Mäntylä, T. (2013). Gender differences in multitasking reflect spatial ability. Psychological Science, 24(5), 514–520. doi: 10.1177/0956797612459660
- Mäntylä, T., Carelli, M. G., & Forman, H. (2007). Time monitoring and executive functioning in children and adults. Journal of Experimental Child Psychology, 96, 1–19. doi: 10.1016/j.jecp.2006.08.003
- Mäntylä, T., Coni, V., Kubik, V., Todorov, I., & Del Missier, F. (2017). Time takes space: Selective effects of multitasking on concurrent spatial processing. Cognitive Processing, 18, 229–235. doi: 10.1007/s10339-017-0799-4
- Mäntylä, T., Del Missier, F., & Nilsson, L. G. (2009). Age differences in multiple outcome measures of time-based prospective memory. Aging, Neuropsychology, and Cognition, 16, 708–720. doi: 10.1080/13825580902912721
- Mäntylä, T., & Todorov, I. (2013). Questioning anecdotal beliefs and scientific findings. Psychological Science, 24, 811–812. doi: 10.1177/0956797612475319
- Meyer, D. E., & Kieras, D. E. (1997). A computational theory of executive cognitive processes and multiple-task performance: Part 1. Basic mechanisms. Psychological Review, 104, 3–65. doi: 10.1037/0033-295X.104.1.3
- Miller, D. I., & Halpern, D. F. (2014). The new science of cognitive sex differences. Trends in Cognitive Sciences, 18, 37–45. doi: 10.1016/j.tics.2013.10.011
- Miyake, A., & Friedman, N. P. (2012). The nature and organization of individual differences in executive functions four general conclusions. Current Directions in Psychological Science, 21, 8–14. doi: 10.1177/0963721411429458
- Miyake, A., Friedman, N. P., Emerson, M. J., Witzki, A. H., Howerter, A., & Wager, T. D. (2000). The unity and diversity of executive functions and their contributions to complex “frontal lobe” tasks: A latent variable analysis. Cognitive Psychology, 41, 49–100. doi: 10.1006/cogp.1999.0734
- Morgan, B., D’Mello, S., Abbott, R., Radvansky, G., Haass, M., & Tamplin, A. (2013). Individual differences in multitasking ability and adaptability. Human Factors: The Journal of the Human Factors and Ergonomics Society, 55, 776–788. doi: 10.1177/0018720812470842
- Morris, N., & Jones, D. M. (1990). Memory updating in working memory: The role of the central executive. British Journal of Psychology, 81, 111–121. doi: 10.1111/j.2044-8295.1990.tb02349.x
- Navon, D., & Gopher, D. (1979). On the economy of the human-processing system. Psychological Review, 86, 214–255. doi: 10.1037/0033-295X.86.3.214
- Norman, D. A., & Shallice, T. (1986). Attention to action: Willed and automatic control of behaviour (Revised reprint of Norman and Shallice (1980)). In R. J. Davidson, G. E. Schwartz, & D. Shapiro (Eds.), Consciousness and self-regulation: Advances in research and theory (pp. 1–18). Boston, MA: Springer.
- Nunes, A., & Kramer, A. F. (2009). Experience-based mitigation of age-related performance declines: Evidence from air traffic control. Journal of Experimental Psychology: Applied, 15, 12–24.
- Núñez, R., & Cooperrider, K. (2013). The tangle of space and time in human cognition. Trends in Cognitive Sciences, 17(5), 220–229. doi: 10.1016/j.tics.2013.03.008
- Pashler, H. (1994). Dual-task interference in simple tasks: Data and theory. Psychological Bulletin, 116(2), 220–244. doi: 10.1037/0033-2909.116.2.220
- Pease, A., & Pease, B. (2003). Why men can only do one thing at a time and women never stop talking. Bhopal: Orion.
- Peters, E., Hess, T. M., Västfjäll, D., & Auman, C. (2007). Adult age differences in dual information processes: Implications for the role of affective and deliberative processes in older adults’ decision making. Perspectives on Psychological Science, 2, 1–23. doi: 10.1111/j.1745-6916.2007.00025.x
- Peters, M., Laeng, B., Latham, K., Jackson, M., Zaiyouna, R., & Richardson, C. (1995). A redrawn Vandenberg and Kuse mental rotations test-different versions and factors that affect performance. Brain and Cognition, 28, 39–58. doi: 10.1006/brcg.1995.1032
- Redick, T. S., Shipstead, Z., Meier, M. E., Montroy, J. J., Hicks, K. L., Unsworth, N., & Engle, R. W. (2016). Cognitive predictors of a common multitasking ability: Contributions from working memory, attention control, and fluid intelligence. Journal of Experimental Psychology: General, 142, 359–379. doi: 10.1037/a0029082
- Risko, E. F., & Gilbert, S. J. (2016). Cognitive offloading. Trends in Cognitive Sciences, 20, 676–688. doi: 10.1016/j.tics.2016.07.002
- Risko, E. F., Medimorec, S., Chisholm, J., & Kingstone, A. (2014). Rotating with rotated text: A natural behavior approach to investigating cognitive offloading. Cognitive Science, 38(3), 537–564. doi: 10.1111/cogs.12087
- Salthouse, T. A., Atkinson, T. M., & Berish, D. E. (2003). Executive functioning as a potential mediator of age-related cognitive decline in normal adults. Journal of Experimental Psychology: General, 132, 566–594. doi: 10.1037/0096-3445.132.4.566
- Salvucci, D. D. (2005). A multitasking general executive for compound continuous tasks. Cognitive Science, 29, 457–492. doi: 10.1207/s15516709cog0000_19
- Salvucci, D. D., & Taatgen, N. A. (2008). Threaded cognition: An integrated theory of concurrent multitasking. Psychological Review, 115, 101–130. doi: 10.1037/0033-295X.115.1.101
- Shallice, T., & Burgess, P. (1991). Deficits in strategy application following frontal lobe damage in man. Brain, 114, 727–741. doi: 10.1093/brain/114.2.727
- Shipstead, Z., Harrison, T. L., & Engle, R. W. (2016). Working memory capacity and fluid intelligence. Perspectives on Psychological Science, 11, 771–799. doi: 10.1177/1745691616650647
- Stocker, K. (2014). The theory of cognitive spacetime. Metaphor and Symbol, 29(2), 71–93. doi: 10.1080/10926488.2014.889991
- Stoet, G., O’Connor, D. B., Conner, M., & Laws, K. R. (2013). Are women better than men at multi-tasking? BMC Psychology, 1, 18–28. doi: 10.1186/2050-7283-1-18
- Strayer, D. L., Medeiros-Ward, N., & Watson, J. M. (2013). Gender invariance in multitasking: A comment on Mäntylä (2013). Psychological Science, 24, 809–810. doi: 10.1177/0956797612465199
- Stroop, J. R. (1935). Stroop color word test. Journal of Experimental Physiology, 18, 643–662.
- Szameitat, A. J., Lepsien, J., Von Cramon, D. Y., Sterr, A., & Schubert, T. (2006). Task-order coordination in dual-task performance and the lateral prefrontal cortex: An event-related fMRI study. Psychological Research, 70(6), 541–552. doi: 10.1007/s00426-005-0015-5
- Tabachnick, B. G., & Fidell, L. S. (2001). Cleaning up your act: Screening data prior to analysis. Using Multivariate Statistics, 5, 61–116.
- Todorov, I., Del Missier, F., Konke, L. A., & Mäntylä, T. (2015). Deadlines in space: Selective effects of coordinate spatial processing in multitasking. Memory & Cognition, 43, 1216–1228. doi: 10.3758/s13421-015-0529-z
- Todorov, I., Del Missier, F., & Mäntylä, T. (2014). Age-related differences in multiple task monitoring. PloS ONE, 9(9), e107619. doi: 10.1371/journal.pone.0107619
- Vandenberg, S. G., & Kuse, A. R. (1978). Mental rotations, a group test of three-dimensional spatial visualization. Perceptual and Motor Skills, 47, 599–604. doi: 10.2466/pms.1978.47.2.599
- Walsh, V. (2003). A theory of magnitude: Common cortical metrics of time, space and quantity. Trends in Cognitive Sciences, 7(11), 483–488. doi: 10.1016/j.tics.2003.09.002
- Wickens, C. D. (2002). Multiple resources and performance prediction. Theoretical Issues in Ergonomics Science, 3, 159–177. doi: 10.1080/14639220210123806
- Wickens, C. D. (2008). Multiple resources and mental workload. Human Factors: The Journal of the Human Factors and Ergonomics Society, 50, 449–455. doi: 10.1518/001872008X288394
- Wilhelm, O., Hildebrandt, A., & Oberauer, K. (2013). What is working memory capacity, and how can we measure it? Frontiers in Psychology, 4(433), 1–22.
- Wilson, M. (2002). Six views of embodied cognition. Psychonomic Bulletin and Review, 9, 625–636. doi: 10.3758/BF03196322
- Wilson, A. D., & Golonka, S. (2013). Embodied cognition is not what you think it is. Frontiers in Psychology, 4, 263–275. doi: 10.3389/fpsyg.2013.00058
- Zhang, J., & Norman, D. A. (1994). Representations in distributed cognitive tasks. Cognitive Science, 18(1), 87–122. doi: 10.1207/s15516709cog1801_3