ABSTRACT
Individuals show large individual differences in arithmetic abilities, especially in arithmetic facts. However, it is still unclear if individual differences in these abilities can be explained through individual differences in sensitivity to interference during retrieval from the associative network, and whether this sensitivity to interference is domain-general. To this end, we compared 18 adults with low arithmetic abilities with 18 intelligence matched individuals with high arithmetic abilities in two arithmetic and one lexico-semantic task. While individuals with low arithmetic abilities performed worse (longer response time and lower accuracy) on related lures, where interference occurs, individuals with high arithmetic abilities performed equally well on related and unrelated lures. This pattern of results emerged in the arithmetic tasks as well as in the lexico-semantic task. This finding indicates that sensitivity to interference is a domain-general construct hindering the selective retrieval of information from long-term memory in individuals with low arithmetic abilities.
1. Introduction
Arithmetic abilities are essential to operate confidently and independently in everyday life, education, and work (Gross et al., Citation2009). In the past decades, several studies have revealed large individual differences in these abilities (Dowker, Citation2019). This holds particularly true for solving arithmetic problems by directly retrieving the solution from an arithmetic fact network in long-term memory (fact retrieval; e.g. in single-digit multiplication problems; De Smedt, Citation2016). Difficulties in retrieving arithmetic facts have been explained through interference effects when accessing this associative network (Verguts & Fias, Citation2005). However, it is still not clear whether individual differences in these interference effects can account for individual differences in arithmetic abilities and whether they are domain-specific or domain-general.
In general, there is wide consensus that arithmetic facts are stored in an associative network (Ashcraft, Citation1992; Verguts & Fias, Citation2005), where problems and solutions can be represented as connected nodes. Given this network structure, a confrontation with a problem does not only activate the correct solution but also other associated nodes (e.g. incorrect solutions). Similar arithmetic problems are connected more closely, which leads to a coactivation of their respective solutions. This especially applies to arithmetic facts that share one or more operands (Campbell, Citation1997). For instance, the problem 6 × 8, would not only activate its correct answer 48, but also 40, 56, 42, and 54, which are the correct solutions to the neighbouring problems 5 × 8, 7 × 8, 6 × 7, and 6 × 9. This type of interference during arithmetic fact retrieval typically emerges in the operand-related-lure (OR) task. In this task, single-digit multiplications are presented together with a solution that must be evaluated for correctness. Incorrect solutions are either operand-related lures that belong to the same multiplication table (e.g. 6 × 8 = 40 [5 × 8]) or operand-unrelated lures that are numerically close to the correct solution but do not belong to a related multiplication table (e.g. 6 × 8 = 44). Further, arithmetic facts can also be related by sharing both operands but differing in their operation. The problem 3 × 4, for instance, not only activates its correct answer 12, but also 7, which is the correct solutions for 3 + 4 (Winkelman & Schmidt, Citation1974; Zbrodoff & Logan, Citation1986). In other words, in multiplication tasks the respective addition problem is activated and vice versa. This type of interference has been examined in the associative confusion task (AC). Single-digit multiplications are presented with different types of lures: Associative lures are related to the correct multiplication solution, because they are the correct solution for the respective addition (e.g. 3 × 4 = 7 [3 + 4]), whereas non-associative lures are numerically close to the correct solution but otherwise unrelated to it (e.g. 3 × 4 = 11).
In adults, studies consistently show that related as well as associative lures are solved less accurately and more slowly than unrelated as well as non-associative lures (Domahs et al., Citation2007; Megías et al., Citation2015; Stazyk et al., Citation1982; Zbrodoff & Logan, Citation1986, Citation1990). This interference effect has also been demonstrated using other conceptually similar paradigms (e.g. negative priming: Megías et al., Citation2015; retrieval induced forgetting: Campbell & Thompson, Citation2012; Galfano et al., Citation2011). Overall, this evidence indicates that arithmetic facts are stored in an associative network and that incorrect solutions interfere with retrieving the correct solution. Results from this work also indicate that executive functions might be involved in overcoming incorrect competitive solutions (Campbell & Thompson, Citation2012; Galfano et al., Citation2011) and that domain-general inhibitory mechanisms play a central role in this process (Anderson et al., Citation2016; Megías et al., Citation2015). In this account, individual differences in arithmetic abilities emerge as factor of differences in inhibition abilities (e.g. Szucs et al., Citation2013).
However, individual differences in arithmetic might also relate to differences in the sensitivity to interference. De Visscher and Noël (Citation2014b) postulated that interference effects in the arithmetic fact network already emerge during the acquisition of arithmetic facts, and that feature overlap between arithmetic facts cause interference during learning. By operationalising this overlap into an interference parameter, De Visscher and Noël (Citation2014a) showed that problems with higher interference are solved more slowly and less accurately. Further, research demonstrated that differences in arithmetic abilities are related to individual variations in the sensitivity to interference both on the behavioural (De Visscher et al., Citation2016) and neural level (De Visscher et al., Citation2018). Additional behavioural work showed that this relationship is not limited to arithmetic but also transfers to non-arithmetic tasks, suggesting a domain-general rather than a domain-specific influence (De Visscher et al., Citation2015; De Visscher & Noël, Citation2014b).
Since previous studies on individual differences in sensitivity to interference have focused on newly learned material, it is unclear if individual differences in the sensitivity to interference during the retrieval of information from long-term memory have a similar relationship with arithmetic abilities. In addition, it remains an open question whether such a relationship is domain-specific (i.e. restricted to the retrieval of arithmetic facts) or domain-general (i.e. can also be found when retrieving non-numerical knowledge). The aim of the present study is to provide first answers to both questions.
In this study, we administered the two previously described arithmetic tasks (AC, OR) to measure sensitivity to interference during the retrieval of arithmetic facts from semantic long-term memory. To examine whether the ability-interference relationship is domain-specific or domain-general, we employed a well-established lexico-semantic interference task, the picture-word (PW) task. In this task, pictures are presented with a word and participants must evaluate whether those two have the same meaning. Again, incorrect solutions are divided into two types of solutions. Related lures belong to the same semantic category as the picture (e.g. picture: dog, word: cat). Unrelated lures are from a different semantic category than the picture (e.g. picture: flower, word: hat). Like the AC and OR task, related lures have been found to be solved more slowly and with a lower accuracy than unrelated lures (e.g. Costa et al., Citation2005).
The main research question in the present study is if individuals with low arithmetic abilities (low AA) differ from individuals with high arithmetic abilities (high AA) in their sensitivity to interference during the retrieval of information from long-term memory across different domains. Based on previous studies, we expect individuals with low AA to solve related lures more slowly and/or less accurately than unrelated lures, indicating a higher sensitivity to interference. In contrast, individuals with high AA are expected to solve related and unrelated lures equally fast and accurate. If sensitivity to interference is a domain-general construct, this pattern should not only be evident in the two arithmetic tasks but also in the lexico-semantic task.
2. Method
2.1. Participants
Participants for this study were recruited from a large preexisting sample of German-speaking adults (N = 425), who had been prescreened on a number of relevant psychometric measures.Footnote1 From these, we used demographic data, intelligence scores, mathematical competence, and arithmetic ability (see below) for the current study. Here, we compared two groups: individuals with high AA, who were recruited from the top 25% in arithmetic ability, and individuals with low AA, who were recruited from the bottom 25%.
The final sample consisted of 36 individuals (23 females) aged between 18 and 42 years (M = 23.81, SD = 5.84). The majority (84.4%) of participants were enrolled as students at the local university. The high AA (N = 18) and low AA (N = 18) groups did not differ in their mean age or sex distribution (low AA: 14 females; high AA: 9 females; Χ2 = 3.01, p = .083). While the original sampling was based on arithmetic ability, in the end, the two groups were not just defined by arithmetic differences but also by differences in broader mathematical competencies and by numerical intelligence (see ). However, apart from numerical intelligence, both groups did not differ in intelligence. All participants gave written informed consent prior to participation and were compensated monetarily for their time. This study was approved by the local ethics committee.
Table 1. Descriptive statistics (M, SD) for the low arithmetic abilities group (low AA) and the high arithmetic abilities group (high AA) as well as test statistics derived from independent samples t-tests.
2.2. Materials and stimuli
2.2.1. Arithmetic abilities
Arithmetic ability was measured using a paper-pencil arithmetic fluency test (Vogel et al., Citation2017) with the number of correctly solved problems as outcome measure. The task included 64 simple additions, consisting of two single-digit operands, 128 simple multiplications, consisting of two single-digit operands, and 128 simple subtractions, consisting of one operand smaller than 20 and one single-digit operand. Participants were given 90 s per type of operation to solve as many problems as possible. The task further included 60 complex additions, consisting of three double-digit operands, 60 complex multiplications, consisting of one double-digit and one single-digit operand, and 60 complex subtractions, consisting of two double-digit operands. Participants were given 120 s per type of operation to solve as many problems as possible.
2.2.2. Mathematical competence
Mathematical competence was measured with the Mathematics Test for Personnel Selection (Jasper & Wagener, Citation2013). The short form used measures performance in higher-order mathematics and covers the topics fractions, conversion of units, exponentiation, division with decimals, algebra, geometry, roots, and logarithm. Participants had a maximum of 15 min to answer 31 open-ended as well as multiple-choice questions. The mathematical competence score is the sum of all correct answers given.
2.2.3. Intelligence
Intelligence scores were measured with the Berlin Intelligence Structure Test (Jäger et al., Citation1997). The short version used includes five subtasks for each of the three domains of intelligence (numerical (N), verbal (V), and figural (F)) and covers the operational abilities processing speed (S), memory (M), reasoning (R), and creativity (C). The fifteen subtasks are: x-greater (N-S), part whole (V-S), crossing letters (F-S), number pairs (N-M), text with sense (V-M), orientation memory (F-M), number sequence (N-R), estimation (N-R), verbal analogies (V-R), fact opinion (V-R), figural analogies (F-R), charkow (F-R), divergent calculation (N-C), properties abilities (V-C), and layout (F-C). This test took approximately 45 min and for further analyses we summed up the raw scores for each of the three domains.
Scoring for the arithmetic abilities, mathematical competence, and intelligence test was done according to the manual by one trained individual using evaluation templates.
2.2.4. Associative confusion (AC)
In the AC task (Lemaire et al., Citation1991) participants had to verify single-digit multiplications as well as single-digit additions. Half of the trials were correct, the other half were incorrect. The incorrect solutions were constructed in two different ways, they were either related lures or unrelated lures. Related lures were solutions which were incorrect in the presented operation but correct in the other operation (e.g. 3 + 4 = 12[3×4]). Unrelated lures were constructed by either adding 1 or 2 to the related lure or by subtracting 1 or 2 to from the related lure. Before starting the main task, 10 practice items, on which participants received feedback, had to be solved. Overall, 208 trials (50% correct, 25% related, 25% unrelated) were presented, split into four runs, resulting in a task duration of approximately 25 min.
2.2.5. Operand related lure (OR)
The OR task (Domahs et al., Citation2007) is conceptually similar to the AC task, however, participants had to verify single-digit multiplications. Related lures were solutions from a multiplication problem with one related operand, like the neighbour problem (e.g. 2 × 7 = 16[2×8]); they were constructed by adding or subtracting 1 to one of the problem’s operands. Unrelated lures were solutions that did not belong to any multiplication table (e.g. 8 × 4 = 26), but which were matched for parity (Krueger, Citation1986) and decade (Domahs et al., Citation2007) with the related lures. The 50% of the incorrect solutions were evenly split into related and unrelated lures. Again, participants solved 10 practice trials followed by 208 multiplications organised into four runs, resulting in in a task duration of approximately 25 min.
2.2.6. Picture-Word (PW)
The PW task was constructed with the aim to be a non-numerical equivalent to the AC and the OR task (Heidekum et al., Citation2019). Participants had to judge whether a concept displayed on a picture matched the meaning of a subsequent word. Like the numerical paradigms described above, half of the items were correct, meaning the picture was presented with its correct word concept, and the other half were incorrect. Incorrect trials were either related lures, where the picture and the word were from the same semantic category (e.g. dog–cat) or unrelated lures, where the picture and the word were from different semantic categories (e.g. flower–hat). To familiarise participants with the material, all 28 pictures in combination with the correct word were shown for 2000 ms each. Again, participants did 10 practice trials followed by 224 items split into four runs. The PW paradigm had a task duration of approximately 30 min.
2.3. Experimental procedure
The data were collected as part of an EEG study and EEG signals were recorded during task performance. However, only behavioural results will be reported here. All paradigms were programmed with PsychoPy 2 and presented on a 21″ computer screen. Responses were given by using the number pad on the keyboard. Participants were tested individually. After receiving general instruction about the test session, participants gave informed consent. The order of the three tasks (AC, OR and PW) was randomised for each participant with short self-paced breaks between tasks. All trials in the three tasks followed the same sequence: After a fixation the operation/picture was presented followed by a solution/word. Trials were separated by a jittered inter-stimulus-interval (blank screen) with a mean duration of 1500 ms (see ). Participants were instructed to answer as accurately and as fast as possible. If the proposed solution was correct, participants should press the key “4” (stickered with a green dot) on the number pad. If the proposed solution was incorrect the key “6” (stickered with a red dot) had to be pressed. Overall, the test session took approximately two and a half hours due to the EEG measurements, pure processing time was 90 min.
2.4. Data analysis
This study realised a 2 × 2 design with the between-subject variable arithmetic abilities (low AA vs. high AA) and the within subject variable interference (related vs. unrelated). Trials where the presented answer was correct were not included in the analysis. To analyse if individuals with low AA were more sensitive to interference during memory retrieval compared to individuals with high AA, six mixed analyses of variances were computed. The dependent variables were accuracy and response time of each task. Trials with response times below 200 ms were excluded, as these are not considered valid (e.g. Berger & Kiefer, Citation2021; Niedeggen et al., Citation1999; Whelan, Citation2008). Response time reflects the trimmed mean (excluding the outer 10% of the distribution) of the response duration of all correctly answered trials. All calculations were done using IBM SPSS Statistics (Version 28; IBM Corp., Citation2021). A post hoc power analysis using G-power (Faul et al., Citation2007) showed that the achieved power for finding an interaction effect (two-tailed, p < .05) was .54 for AC response time, .99 for AC accuracy, 1.00 for OR response time, OR accuracy and PW response time, and .89 for PW accuracy.
Table 2. Test statistics (M, SD) for the 2 × 2 Mixed ANOVA with the between-subject variable arithmetic abilities (low AA vs. high AA) and the within subject variable interference (related vs. unrelated).
3. Results
3.1. Associative confusion (AC)
We found a significant main effect of arithmetic abilities both for response time and accuracy (see ). As expected, the high AA group solved additions and multiplications faster and with higher accuracy than the low AA group. While we found no significant main effect of interference for response time, showing that related and unrelated lures were solved equally fast (see b), the main effect of interference was significant for accuracy. In line with our expectations, related lures were answered less accurately than unrelated lures.
Figure 2. Means and 95% CI of (a) accuracy; (b) response times in milliseconds for the three tasks Associative Confusion (AC), Operand-Related Lure (OR), and Picture-Word (PW) separated for the low arithmetic abilities group (low AA) and the high arithmetic abilities group (high AA). * indicates a significant difference (p < .050) between related and unrelated lures in post-hoc comparisons with Bonferroni adjustment for multiple comparisons.
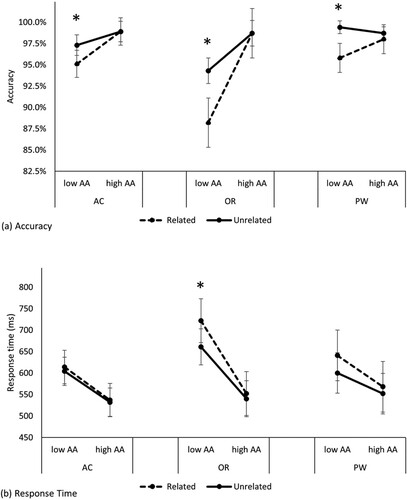
Contrary to our expectations, there was no significant interaction between arithmetic abilities and interference for response time. However, the interaction for accuracy was significant, so we conducted post-hoc pairwise tests with Bonferroni correction for multiple comparison. As expected, individuals with low AA solved related lures less accurately than unrelated lures (p = .003), whereas individuals with high AA solved related and unrelated lures equally well (p = .964; see a).
3.2. Operand related lure (OR)
Similar to the first arithmetic task, we found a significant main effect of arithmetic abilities both in response time and accuracy (see ). The high AA group solved the additions and multiplications faster and with a higher accuracy than the low AA group. We also found a significant main effect of interference for both response time and accuracy. In line with your expectations, related lures were generally answered less accurately and slower than unrelated lures.
Significant interaction effects were observed in the OR task with respect to response time and accuracy. Post-hoc pairwise comparison with Bonferroni correction for multiple comparison confirmed our hypothesis. Individuals with low AA solved related lures slower (p < .001) and less accurately (p < .001) compared to unrelated lures. Individuals with high AA neither differed in response time (p = .266) nor in accuracy (p = .996) between interference conditions (see a,b).
3.3. Picture-Word (PW)
As the PW task is a lexico-semantic task, and not an arithmetic task, we did not expect general between group differences, which was also the case. The main effect arithmetic abilities was neither significant for response time nor for accuracy (see ). The main effect interference was significant for both response time and accuracy. Overall, related lures were solved slower and less accurate than unrelated lures.
The interaction for response time was not significant, indicating that arithmetic abilities did not influence the interference effect. However, the interaction for accuracy was significant. Post-hoc pairwise comparison with Bonferroni correction for multiple comparison confirmed our hypothesis. Individuals with low AA solved related lures less accurate (p < .001) compared to unrelated lures. Individuals with high AA did not differ in accuracy (p = .461) depending on interference (see a).
4. Discussion
This study tested whether individual differences in interference effects during the retrieval of information from long-term memory across different domains are related to arithmetic abilities in adults. To this end, we compared individuals with low AA with individuals with high AA during two arithmetic and one lexico-semantic task inducing retrieval interference. As expected, individuals with low AA needed significantly more time and made more mistakes while working on related lures compared to unrelated lures. In contrast, individuals with high AA solved related and unrelated lures equally accurately. This pattern of results was similar for the two arithmetic tasks but also for the lexico-semantic task.
Our results are the first to show that individual differences in arithmetic abilities are related to sensitivity to interference during retrieval. This is in line with previous studies showing that individual differences in arithmetic abilities are related to sensitivity to interference during acquiring arithmetic knowledge (for a summary see Noël & De Visscher, Citation2018). Not only is the performance on arithmetic facts influenced by the interference, but individuals with poor arithmetic abilities were more sensitive to this kind of interference (De Visscher & Noël, Citation2014a; Dotan & Friedmann, Citation2019). However, the interference occurs during the learning of arithmetic facts and the reason for the interference is the mere overlap of features. This only reflects one way of how arithmetic facts and their solutions are related. In contrast, the two tasks in our study induced two different ways of how arithmetic facts are related, caused by the way how arithmetic facts are stored in an associative network, and therefore focused on interference during retrieval from long-term memory.
Interestingly, while people with low AA showed higher sensitivity to interference in terms of lower accuracy and longer response time in the OR task, this effect was limited to accuracy in the AC task. One assumption why we did not find an interaction in the response time of the AC task could be the small differences in accuracy to begin with. Individuals with low AA solved on average 95% of the trials in the related condition and 97.5% in the unrelated condition, which can be translated into 1–2 trials difference. Thus, this small difference may also transfer to response times, suggesting that we have not enough power to detect a potential subtle response time effect. This could also indicate that neighbouring multiplications are simply linked more strongly to the problem and its solution than problems with the same operands but a different operation sign. This could be related to the fact that incorrect solutions in the AC task were numerically more distant from the correct solution, compared to the incorrect and correct solutions in the OR task.
While we hypothesise that individuals with low AA might be more sensitive to interference than individuals with high AA, it might also be the case that the two groups differ in their ability to overcome the interference from strong competitors (i.e. related lures) due to differences in inhibitory control processes. Indeed, several studies suggest that overcoming interference involves executive functions (Campbell & Thompson, Citation2012; Galfano et al., Citation2011) and in particular inhibitory mechanisms (Anderson et al., Citation2016; Megías et al., Citation2015). Some research also suggests that poor inhibitions skills might explain low arithmetic abilities (e.g. Szucs et al., Citation2013), while other studies found inhibition to be not related to arithmetic fact retrieval (e.g. Bellon et al., Citation2016). For a recent and more complete review on the relationship between interference and arithmetic see Medrano and Prather (Citation2023).
We additionally wanted to find out if individual differences in arithmetic abilities are only related to inference during the retrieval of arithmetic knowledge or whether sensitivity to interference is rather a domain-general construct. To this end, we used the PW task as a lexico-semantic equivalent to the AC and OR task. Identical to the significant interaction in the two arithmetic tasks, the low AA group also showed a significant sensitivity to interference in the PW task, which the high AA group did not. As both groups were matched for verbal intelligence and no general performance differences were found in the lexico-semantic task, we concluded that this interaction effect is mainly caused by differences in arithmetic abilities. This is also corroborated by a post-hoc correlation analysis that indicated significant correlations between arithmetic abilities and the interference effect across all tasks. No such correlations were found for numerical intelligence and mathematical competence (those analyses can be found in the analysis script on OSF).
Despite the careful planning of the study, it suffers from certain limitations. First, even though our post hoc power analysis indicated appropriate power, our sample size (N = 36) is rather small, and this has to be considered when drawing conclusions from our findings. A high-powered replication study, where the sample size is determined by an a-priori power analysis, is therefore recommended. Second, while we put our focus on individual difference in arithmetic abilities, the two groups also differed in numerical intelligence and mathematical competence, thus raising the question what underlying factors are responsible for between group differences. While additional analyses (see above), showed that indeed arithmetic abilities are uniquely associated to individual differences in sensitivity to interference during retrieval, the other two factors cannot be completely disregarded. Especially because all three variables are related to each other (arithmetic abilities correlated at r = .63 with numerical intelligence and at r = .45 with mathematical competence in our sample) and because arithmetic abilities are considered as a precursor for more complex mathematical abilities (e.g. De Smedt, Citation2016). Therefore, future studies should more systematically explore the possible influence of numerical intelligence and mathematical competence.
Taken together, our findings are in line with previous work and extend our understanding to a significant amount. This study strengthens the theory that sensitivity to interference during retrieval from long-term memory may be one cause for individual differences in arithmetic abilities and especially in arithmetic fact knowledge. It extends previous research by showing that sensitivity to interference seems to be a domain-general construct that is not limited to the arithmetic domain and this sensitivity occurs during the retrieval of deeply anchored material, which is stored in an associative network in semantic long-term memory. Further research is needed to find out if and how individuals can reduce their sensitivity to interference, and if these improvements can be transferred to eventually improve arithmetic abilities.
Disclosure statement
No potential conflict of interest was reported by the author(s).
Data availability statement
The data as well as the analyses that support the findings of this study are openly available in OSF at http://doi.org/10.17605/OSF.IO/GYEKW.
Notes
1Other measures included in the prescreening were personality measures (e.g., Big Five, Math Anxiety) as well as computerized measures (e.g., Cardinality, Ordinality).
References
- Anderson, M. C., Bunce, J. G., & Barbas, H. (2016). Prefrontal–hippocampal pathways underlying inhibitory control over memory. Neurobiology of Learning and Memory, 134, 145–161. https://doi.org/10.1016/j.nlm.2015.11.008
- Ashcraft, M. H. (1992). Cognitive arithmetic: A review of data and theory. Cognition, 44(1-2), 75–106. https://doi.org/10.1016/0010-0277(92)90051-I
- Bellon, E., Fias, W., & De Smedt, B. (2016). Are individual differences in arithmetic fact retrieval in children related to inhibition? Frontiers in Psychology, 7(JUN), 1–11. https://doi.org/10.3389/fpsyg.2016.00825
- Berger, A., & Kiefer, M. (2021). Comparison of different response time outlier exclusion methods: A simulation study. Frontiers in Psychology, 12(June), 1–13. https://doi.org/10.3389/fpsyg.2021.675558
- Campbell, J. I. D. (1997). On the relation between skilled performance of simple division and multiplication. Journal of Experimental Psychology: Learning, Memory, and Cognition, 23(5), 1140–1159. https://doi.org/10.1037/0278-7393.23.5.1140
- Campbell, J. I. D., & Thompson, V. A. (2012). Retrieval-induced forgetting of arithmetic facts. Journal of Experimental Psychology: Learning, Memory, and Cognition, 38(1), 118–129. https://doi.org/10.1037/a0025056
- Costa, A., Alario, F.-X., & Caramazza, A. (2005). On the categorical nature of the semantic interference effect in the picture-word interference paradigm. Psychonomic Bulletin & Review, 12(1), 125–131. https://doi.org/10.3758/BF03196357
- De Smedt, B. (2016). Individual differences in arithmetic fact retrieval. In D. Berch, D. Geary, & K. Koepke (Eds.), Development of Mathematical Cognition (pp. 219–243). Elsevier. https://doi.org/10.1016/B978-0-12-801871-2.00009-5
- De Visscher, A., & Noël, M. (2014b). Arithmetic facts storage deficit: the hypersensitivity-to-interference in memory hypothesis. Developmental Science, 17(3), 434–442. https://doi.org/10.1111/desc.12135
- De Visscher, A., & Noël, M.-P. (2014a). The detrimental effect of interference in multiplication facts storing: Typical development and individual differences. Journal of Experimental Psychology: General, 143(6), 2380–2400. https://doi.org/10.1037/xge0000029
- De Visscher, A., Noël, M.-P., & De Smedt, B. (2016). The role of physical digit representation and numerical magnitude representation in children’s multiplication fact retrieval. Journal of Experimental Child Psychology, 152, 41–53. https://doi.org/10.1016/j.jecp.2016.06.014
- De Visscher, A., Szmalec, A., Van Der Linden, L., & Noël, M.-P. (2015). Serial-order learning impairment and hypersensitivity-to-interference in dyscalculia. Cognition, 144, 38–48. https://doi.org/10.1016/j.cognition.2015.07.007
- Domahs, F., Domahs, U., Schlesewsky, M., Ratinckx, E., Verguts, T., Willmes, K., & Nuerk, H.-C. (2007). Neighborhood consistency in mental arithmetic: Behavioral and ERP evidence. Behavioral and Brain Functions, 3(1), 66. https://doi.org/10.1186/1744-9081-3-66
- Dotan, D., & Friedmann, N. (2019). Reducing interference improves the memorization of multiplication facts in case of hypersensitivity to interference. Journal of Numerical Cognition, 5(3), 400–430. https://doi.org/10.5964/jnc.v5i3.203
- Dowker, A.. (2019). Individual Differences in Arithmetic (Vol. 7) (pp. 5–28). Routledge. https://doi.org/10.4324/9781315755526-2
- Faul, F., Erdfelder, E., Lang, A.-G., & Buchner, A. (2007). G*Power 3: A flexible statistical power analysis program for the social, behavioral, and biomedical sciences. Behavior Research Methods, 39(2), 175–191. https://doi.org/10.3758/BF03193146
- Galfano, G., Penolazzi, B., Fardo, F., D’Hooge, E., Angrilli, A., & Umiltà, C. (2011). Neurophysiological markers of retrieval-induced forgetting in multiplication fact retrieval. Psychophysiology, 48(12), 1681–1691. https://doi.org/10.1111/j.1469-8986.2011.01267.x
- Gross, J., Hudson, C., Price, D., & Trust, E. C. a. C. (2009). The long term cost of literacy difficulties. January.
- Heidekum, A. E., Grabner, R. H., De Smedt, B., De Visscher, A., & Vogel, S. E. (2019). Interference during the retrieval of arithmetic and lexico-semantic knowledge modulates similar brain regions: Evidence from functional magnetic resonance imaging (fMRI). Cortex, 120, 375–393. https://doi.org/10.1016/j.cortex.2019.06.007
- IBM Corp. Released. (2021). Ibm SPSS statistics for windows, version 28.0. IBM Corp.
- Jäger, A. O., Süß, H.-M., & Beauducel, A. (1997). Berliner Intelligenzstruktur-Test (BIS) [Berlin intelligence structure test]. Hoegrefe.
- Jasper, F., & Wagener, D. (2013). Mathematiktest für die personalauswahl (MPA) [Mathematics test for personnel selection]. Hogrefe.
- Krueger, L. E. (1986). Why 2×2=5 looks so wrong: On the odd-even rule in product verification. Memory & Cognition, 14(2), 141–149. https://doi.org/10.3758/BF03198374
- Lemaire, P., Fayol, M., & Abdi, H. (1991). Associative confusion effect in cognitive arithmetic: Evidence for partially autonomous processes. Cahiers de Psychologie Cognitive, 11, 587–604.
- Medrano, J., & Prather, R. W. (2023). Rethinking executive functions in mathematical cognition. Journal of Cognition and Development, 24(2), 280–295. https://doi.org/10.1080/15248372.2023.2172414
- Megías, P., Macizo, P., & Herrera, A. (2015). Simple arithmetic: evidence of an inhibitory mechanism to select arithmetic facts. Psychological Research, 79(5), 773–784. https://doi.org/10.1007/s00426-014-0603-3
- Niedeggen, M., Rösler, F., & Jost, K. (1999). Processing of incongruous mental calculation problems: Evidence for an arithmetic N400 effect. Psychophysiology, 36(3), 307–324. https://doi.org/10.1017/S0048577299980149
- Noël, M.-P., & De Visscher, A. (2018). Hypersensitivity-to-interference in memory as a possible cause of difficulty in arithmetic facts storing. In A. Henik & W. Fias (Eds.), Heterogeneity of Function in Numerical Cognition (pp. 387–408). Elsevier. https://doi.org/10.1016/B978-0-12-811529-9.00018-2
- Stazyk, E. H., Ashcraft, M. H., & Hamann, M. S. (1982). A network approach to mental multiplication. Journal of Experimental Psychology: Learning, Memory, and Cognition, 8(4), 320–335. https://doi.org/10.1037/0278-7393.8.4.320
- Szucs, D., Devine, A., Soltesz, F., Nobes, A., & Gabriel, F. (2013). Developmental dyscalculia is related to visuo-spatial memory and inhibition impairment. Cortex, 49(10), 2674–2688. https://doi.org/10.1016/j.cortex.2013.06.007
- Verguts, T., & Fias, W. (2005). Interacting neighbors: A connectionist model of retrieval in single-digit multiplication. Memory & Cognition, 33(1), 1–16. https://doi.org/10.3758/BF03195293
- Visscher, D., Vogel, A., Reishofer, S. E., Hassler, G., Koschutnig, E., De Smedt, K., Grabner, B., & H, R. (2018). Interference and problem size effect in multiplication fact solving: Individual differences in brain activations and arithmetic performance. NeuroImage, 172, 718–727. https://doi.org/10.1016/j.neuroimage.2018.01.060
- Vogel, S. E., Haigh, T., Sommerauer, G., Spindler, M., Brunner, C., Lyons, I. M., & Grabner, R. H. (2017). Processing the order of symbolic numbers: A reliable and unique predictor of arithmetic fluency. Journal of Numerical Cognition, 3(2), 288–308. https://doi.org/10.5964/jnc.v3i2.55
- Whelan, R. (2008). Effective analysis of reaction time data. The Psychological Record, 58(3), 475–482. https://doi.org/10.1007/BF03395630
- Winkelman, J. H., & Schmidt, J. (1974). Associative confusions in mental arithmetic. Journal of Experimental Psychology, 102(4), 734–736. https://doi.org/10.1037/h0036103
- Zbrodoff, N. J., & Logan, G. D. (1986). On the autonomy of mental processes: A case study of arithmetic. Journal of Experimental Psychology: General, 115(2), 118–130. https://doi.org/10.1037/0096-3445.115.2.118
- Zbrodoff, N. J., & Logan, G. D. (1990). On the relation between production and verification tasks in the psychology of simple arithmetic. Journal of Experimental Psychology: Learning, Memory, and Cognition, 16(1), 83–97. doi:10.1037/0278-7393.16.1.83