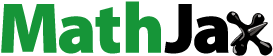
ABSTRACT
This study concerns a model of the fuel consumption and emissions from the bottom longline fishing fleet at Rio de Janeiro State, Brazil with data comprised vessel and engine characteristics, fuel consumption, landings and fishing trip duration of seventeen longliners operating in Rio de Janeiro between 2015 and 2016. The operational pattern was investigated for this fleet, based on an empirical approach to model the propulsion power requirements of the typical longliner fishing vessel. The current propulsion and power plants of the longliner fishing vessels under consideration are compared with alternative designs by estimating fuel consumption for the different models of propulsion systems. When the operational pattern evaluation is taken into account, it indicates the future potential for diesel-electric propulsion for longliner fishing vessels, as a result of a reduction in fuel consumption.
Nomenclature
CH4 | = | Methane |
CO2e | = | Carbon dioxide equivalents |
D | = | Propeller diameter |
Ev | = | Estimation error for each vessel |
FAO | = | Food and agriculture organisation |
FIPERJ | = | Fishing Institute Foundation of the State of Rio de Janeiro |
GHG | = | Greenhouse gas |
ICE | = | Internal combustion engine |
IPCC | = | Intergovernmental panel on climate change |
J | = | Advance coefficient |
Kq | = | Torque coefficient |
Kt | = | Thrust coefficient |
MAPA | = | Ministry of Agriculture, Livestock and Supply |
MAPE | = | Mean absolute percentage error |
MPA | = | Ministry of Fisheries and Aquaculture |
N2O | = | Nitrous oxide |
NPV | = | Net present value |
ηo | = | Open water efficiency |
ηrr | = | Relative rotative efficiency |
ηt | = | Transmission efficiency |
P | = | Engine power |
P/D | = | Pitch-diameter ratio |
Pd | = | Delivered power |
Pe | = | Effective power |
Pi | = | Installed power |
Pt | = | Thrust power |
Rt | = | Total ship resistance |
RPM | = | Engine rpm (revolutions per minute) |
SFC | = | Specific fuel consumption |
t | = | Operating time of a vessel |
Va | = | Speed of advance |
Yv | = | Total fuel consumption reported for each vessel |
1. Introduction
Energy use has been of great concern within fisheries, because this economic activity sector is highly dependent on fossil fuels for energy needs, especially in fish catching activities (Driscoll and Tyedmers Citation2010; Hua and Wu Citation2011; Muir Citation2015).
Globally, fuel costs represent from 30% to 50% of the total costs of fishing fleets and it can reach up to 20–30% of the total catch value, with higher consumption in small-scale fisheries, especially in developing countries (Van Beek and Van der Steenhoven Citation2005; Basurko et al. Citation2012; Parker and Tyedmers Citation2015; Gabiña et al. Citation2016).
As a consequence of the growing concern for fuel prices and environmental awareness, there has been a growing interest by ship owners to look into new technologies and solutions regarding propulsion and the power plant. (Schau et al. Citation2009; Fernández et al. Citation2010; Sala et al. Citation2010; Gulbrandsen Citation2012; Notti and Sala Citation2012; Basurko et al. Citation2013; Logason Citation2015).
Fuel consumption of a fishing vessel depends on its operating profile and fishing method (Thrane Citation2004; Karlsen Citation2012; Suuronen et al. Citation2012).
Longline fishing vessels have a characteristic operational profile, which combines periods of high speed (line setting and hauling) with periods of low power demand (waiting), in addition to the periods of sailing between fishing grounds at a higher speed. Therefore, there is great potential for reducing fuel consumption through the use of propulsion systems more suited to its mode of operation, such as electric or hybrid diesel propulsion (Fernández et al. Citation2010; Logason Citation2015).
The objective of the paper is to identify the level of energy consumption in longliner fishing fleet of Rio de Janeiro, Brazil, quantify the scale of energy use and GHG emissions from the different steps of a typical fishing trip and find the basis for the development of better strategies to reduce fuel consumption and GHG emission.
The GHG emissions from this fishery were determined using the ‘Tier 1’ method and also applied water-borne navigation emission factors which are included in mobile combustion emission factors. Both are provided by the Intergovernmental Panel on Climate Change (IPCC) (IPCC et al. Citation2006; Park et al. Citation2015).
2. Material and methods
2.1. Operational profile of longline fishing
A typical round trip for a bottom longliner consists of several operating situations for different engine loads. While travelling fast to and between the fishing grounds, the vessels travel at a very slow speed during laying and hauling in the lines.
The main important operations of a typical fishing trip were divided into two phases: access and capture.
The access phase to the fishing grounds includes departure, possible paths between fishing grounds and return to the harbour.
Thus, the access to the fishing grounds can be broken down into the following steps: Going to fishing ground; Shifts between fishing grounds (Navigating between different fishing grounds) and Return to the harbour.
The capture phase, which consists in line setting, waiting and hauling, may be repeated several times during a fishing trip and was defined as ‘launching fishing’.
• Setting fishing gear: release the lines with hooks and baits to capture fish;
• Waiting: period in which the ship waits before starting to haul the line;
• Hauling fishing gear: Consists in the retrieving of lines with hooked fish to be taken into the vessel.
2.2. Data collection – research field
This study considered a fleet of seventeen bottom longliner vessels, representing 100% of the Rio de Janeiro fleet or 76% of the total bottom longliner vessels that unload in the main ports of the State (FIPERJ Citation2013).
The data collection was done during field campaigns in cooperation with the Fishing Institute Foundation of the State of Rio de Janeiro (FIPERJ)Footnote1 in 2015 and 2016, where interviews and questionnaires to owners and fishermen operating vessels of the longline fleet were carried out. Table shows the main parameters relating to fishing trips obtained from these interviews.
Table 1. Summary of performance data for the bottom longliner fishing fleet.
The site choices, as well as the number of fishing areas, are specific decisions of each fishing trip since it depends on factors such as the availability of marine resources, climatic factors, etc. Thus, due to the large variation of this parameter and, consequently, the displacement time between the fishing areas, the fuel consumption at this stage was not considered in this analysis.
The age of each vessel is not available. However, a survey carried out by FIPERJ between 2010 and 2012, contains the most recent vessels age data. This study found that 12 of the 17 vessels of the bottom longline fleet of Rio de Janeiro have an average age of 33 years (FIPERJ Citation2013).
Another parameter required for estimating the fuel consumption in each stage of operation is the specific fuel consumption (SFC). The SFC is the amount of fuel required to produce a unit of power per time unit, usually expressed as (l/hp-h), and can be obtained by the performance charts (consumed power with its respective torque and rpm) of the engine, provided by manufacturers. Thus, it is possible to plot different results and trace a consumption curve for the engine under analysis. Despite variations among engines, the specific fuel consumption curve, in general, has its minimum value at the maximum torque point, with a load of around 80% of nominal power and 70–80% of rpm (Wilson Citation1999; Gulbrandsen Citation2012).
In the seventeen analysed vessels, four engines manufactures were identified: Mercedes, MWM, Cummins and Scania. Since the manufacturers provide little information about the specific curves of each type of engine identified during the interviews, due to their variety and different fabrication times, the SFC was estimated considering curves with powers close to the one obtained in the field research stage (Appendix 1). For calculations using the SFC of each curve, the maximum power and rpm were considered.
2.3. Methods for fuel consumption estimation
Three methods drawn from literature addressing specific issues of fishing fleets were used to estimate the fuel consumption by stages of a benchmark trip of fishing, and the results were compared to the data obtained from field research.
2.3.1. Method 1
The method 1 is based on ‘Fuel Savings for Small Fishing Vessels’ (Gulbrandsen Citation2012) and uses the following equation to estimate fuel consumption:
(1)
(1)
where C is the fuel consumption by vessels in a given stage or fishing trip, (l); P is the maximum engine power of the vessel (hp); rpm is the engine rpm (revolutions per minute) at a given stage;
is the Engine maximum rpm; SFC is the specific fuel consumption; represents the amount of fuel required to produce a unit of power per unit of time (l/hp-h); t is the operating time of a vessel at a given stage (h).
The ratio is defined as ‘propeller shaft power fraction’ and indicates the reduction in power consumed by the engine when its rpm is reduced.
2.3.2. Method 2
Method 2 is used by the Brazilian Ministry of Fisheries and Aquaculture (MPA) to estimate the fuel consumed according to the economic diesel subsidy programme, based on a general equation (Brasil Citation2011):
(2)
(2)
where C is the fuel consumption by vessels in a given stage or fishing trip, (l); P is the maximum engine power of the vessel, (hp); SFC is the engine specific fuel consumption (constant value defined as 0.0963 l/hp-h for all fishing gear); t is the operating time of a vessel at a given stage, (h).
2.3.3. Method 3
Method 3 was based on Tyedmers (Citation2001). Data regarding fuel consumption on a fishing trip were obtained from field research, and related to the fishing effort through a linear regression equation:
(3)
(3)
where Y is the total fuel consumption on a fishing trip obtained in the field research; x is the fishing effort, the product of main engine horsepower and total hours at sea by vessels, (hp.sea-hours); a is the slope of the fit line, the generic rate of fuel consumption, which represents the engine specific fuel consumption (SFC), (l/hp.sea-hours).
It is noteworthy that the best fit line was forced through the origin based on the simplifying assumption that there is no fuel consumption when the engine power or time is zero.
With the calculated a value, the fuel consumption in stages of a fishing trip was calculated with the same Equation (3) used in Method 2.
2.4. Estimation error measurements
After submitting the data sample to the proposed estimation methodologies, a comparative analysis was performed among each of the models using MAPE. The MAPE expresses the mean absolute error as a percentage of the actual value:
(4)
(4)
where Ev is the estimation error for each vessel. It is the difference between the reported fuel consumption and estimated consumption; Yv is the total fuel consumption reported for each vessel; N is the number of vessels in the sample.
2.5. Propulsion systems
2.5.1. Mechanical propulsion
Mechanical propulsion system implies the use of the prime over to drive the ship's propeller shaft, either directly or through a gearbox (Geertsma et al. Citation2017). This review focuses on diesel engines used by all fishing vessels in this study.
2.5.2. Diesel-electric propulsion and description of the proposed system
Diesel-electric propulsion is a system driven by an electrical motor providing power for the propeller. The power production for the electrical motor is produced from a diesel generator.
The proposed diesel-electric arrangement for the studied longliner vessel in this work (Figure ) only includes main components, where two gensets are chosen in order to have the flexibility in power generation.
2.6. Current research and development projects analysing electric and hybrid systems
Several projects aimed to identify the energy consumption profile of different types of fishing vessels and reducing fuel use with electric and hybrid configurations have been introduced in fishing a while ago in northern Europe and other regions.
These solutions have been introduced in fishing vessels, mainly longliners, stern trawlers and some tuna purse seiners. Among these pioneers, we may cite a pair of diesel-electric longliners built in Spain in the late eighties. These ships featured a power plant with a 550 kW electric motor and four generators.
Several Spanish longliners, such as two bottom longliners ‘Illa de Rua’ delivered in 1998 and ‘Anchousa’, delivered in 2008, have installed a type of system which consists on using a diesel engine as main propulsion and installing an auxiliary electric motor driving a power take in (PTI) on the reduction gear. The electrical machine operates only as an auxiliary electric motor (electrical power delivered by auxiliary diesel generators) (Fernández et al. Citation2010).
Marin Teknikk AS of Norway designed a diesel-electric bottom longliner which was delivered by Solstrand AS to Ervik Havfiske AS in 2011. The ship, called ‘Froyanes Senior’, is fitted with diesel-electric propulsion with two electric motors (2*800 kW) driving a propeller through a reduction gear. The power generation plant consists of three diesel generators. Frøyanes is the biggest diesel-electric propulsion longliner ever built, equipped with refrigerated fish cargo tanks and fish process factory.
An important analysis of hybrid Longline fishing vessel designs was the project carried out by Alexander Andersson and Kári Logason in 2015. It consists of investigating the feasibility of installing a hybrid propulsion power system into the longliner Jóhanna Gísladóttir GK in the Icelandic fleet. The current propulsion and power plant of this fishing vessel under consideration is compared with alternative designs including hybrid and diesel-electric propulsion in terms of efficiency and bunker consumption. The results show that a diesel-electric system should be saving 22% compared to the baseline system that represents traditional mechanical shaft line propulsion. The hybrid system will be saving about 37% compared to the baseline system and about 19% with regards to the diesel-electric system (Logason Citation2015).
Regarding other fishing fleets, an innovative solution is the one installed in the stern trawler Ms Teigenes. It consists of hybrid propulsion without electrical energy storage system. The total propulsion power of 5800 kW is delivered to a double input reduction gear by a 2000 kW electric motor and a 3800 kW diesel engine. In such a system design the vessel can utilise the power required for the specific operation in pure electric mode, or in diesel mechanical mode, or in a boost mode by engaging both systems. A hybrid system configuration is a fuel efficient and flexible system, with high redundancy. A novel characteristic is the fact that the main diesel engine is able to drive a generator through its forward power take off, giving the ship a higher flexibility.
Another hybrid diesel-electric solution was analysed in a pilot project (Notti et al. Citation2012), through a technical feasibility of a new propulsion system architecture, studied in 2008. In the propulsion system proposed, the overall power required by the vessel is subdivided into multiple power units, each one obtained by coupling a diesel engine with electric generator, while the propeller is coupled with an electric motor. Through an electronic management system, it is possible to maintain one or more power units at different operating points to guarantee the minimum overall fuel consumption. In this study, two power units have been considered. Many load tests have been done on a marine diesel engine, to evaluate its fuel consumption, torque and power delivered against the revolution speed and a fuel saving of up to 15% was achieved with a power unit equipped with a 257 kW and 3800 rpm diesel engine.
2.7. Engine and propeller selection methodology
The overall assessment of the propulsion system for a particular vessel requires knowledge of the required thrust at a speed (V), its conversion into required power, besides the propulsion system efficiency chain.
Table shows the definitions and relationships between the force and power components needed to estimate the total installed power of the propulsion system, based on (Molland et al. Citation2011):
Table 2. Definitions of the main parameters of ship propulsion.
The hull efficiency (ηh) is defined as the ratio between the effective power, and the thrust power, i.e.:
Where t is the thrust deduction factor related to the ship design and w is the wake fraction.
Propeller open water efficiency (ηo) is related to working in open water, i.e. the propeller works in a homogeneous wake field with no hull in front of it (MAN Citation2012a), and the relative rotative efficiency (ηrr) represents the ratio between propeller efficiency working behind the ship (ηp) and open water efficiency.
The design of the propeller and the calculation of the total installed power starts with the estimation of Rt. Then the propeller parameters are determined and, finally, the QPC and total installed power are calculated.
The total installed power will exceed the delivered power by the amount of power lost in the transmission system, and by a design power margin.
2.7.1. Total ship resistance estimation
The estimate of Rt was made based on the method proposed by J. Holtrop and G.G.J. Mennem (Holtrop and Mennen Citation1982).
Vessel with similar dimensions and characteristics to the vessels from the sample and that was within the range of values that allowed the application of the method was modelled.
This vessel was defined as a ‘typical vessel’ of the longline fleet. Its particularities were based on the main dimensions and characteristics to the vessels from the sample and that was within the range of values that allowed the application of the Holtrop and Mennem method (Table ).
Table 3. Main features of the vessel.
The service speed of six knots corresponds to the average speed of the fleet in the going and return steps, obtained in the field research.
The limitations of the Holtrop and Mennem method regarding the range of values of some parameters of the vessel are as follows (Table ):
Table 4. Limits of applicability for Holtrop and Mennen.
where Lwl is the load waterline length; B is the moulded breadth; T is the average draught; Fn is the Froude Number = (dimensionless number related to the calculation of total ship resistance); v is the ship speed; g is the acceleration due to gravity; thus, it was possible to obtain estimates of Rt, the coefficients w and t, and the efficiencies ηh e ηrr necessary to calculate the other efficiencies and power required in the design of the propeller and propulsion engine.
2.7.2. Calculation of propeller parameters by systematic series: diameter; required thrust coefficient and efficiency
The propeller diameter estimate can be based on a percentage of the draft. Through consultation with fishermen, ship owners and specialists from FIPERJ, it has been verified that this percentage varies from about 40% to 60%.
Thus, six values from 0.6 m to 0.85 m in diameter were tested to estimate the values of ηo and to select the propeller which, in addition to reaching the required thrust with the maximum possible efficiency, is within the established limits of cavitation.
A common methodology for carrying out the sizing of a propeller for a specific vessel is the study of the set of systematic series of the propeller (Lewis Citation1988). In general, this set for a given systematic series comprises propeller thrust coefficient (Kt), torque coefficient (Kq) and open water efficiency curves.
The propellers studied are from the systematic series of Wageningen B-series propellers (Bernitsas et al. Citation1981). Ten propeller diagrams with 3 and 4 blades for each of the six test diameters were chosen to select the propeller with the maximum efficiency: B3-45; B3-55; B3-65; B3-75; B3-80; B4-40; B4-55; B4-65; B4-70; B4-85.
The nomenclature of the propellers of this series is represented first by the letter B, symbolising the series. Next, the number and blade area ratio is defined, for example: Propeller B- 4.45 (Series B propeller, with four blades and blade area ratio (BAR) 0.45).
These curves were evaluated in conjunction with the resistance curve required by the vessel for which the propeller is being (Ktrequired), in order to find the propeller that provides the best operating point for the vessel in question. The Ktrequired was obtained from a dimensionless relationship, established between the Ktavailable (curves of the systematic series) and the propeller advance coefficient J:
(5)
(5)
(6)
(6)
(7)
(7)
Where
is the density of the fluid, D is the propeller diameter, Va is the advance speed (speed of advance of the propeller) and n is the rate of propeller revolution.
The operating point is obtained by crossing the available thrust curve with the required thrust curve and thus, the corresponding efficiency and torque coefficient are obtained.
For each of the ten chosen diagrams, there are ten points at which the curve Ktavailable crosses the curve Ktrequired. Therefore ten values for the operating J (one for each propeller pitch-diameter ratio P/D) and their respective efficiencies are found, where the point with greater efficiency is chosen. The optimum propeller selected is the most efficient among the ten diagrams analysed.
With the open water propeller efficiency obtained from the open water diagram and the efficiencies ηrr and ηh calculated accordingly (Holtrop and Mennen Citation1982), we obtain ηp, Delivered Power (Pd) and Quasi-propulsive coefficient (QPC) with the relationship presented in Table .
Therefore, ten propellers with different BARs were tested for each diameter in order to select the most efficient propeller. The values chosen for the BAR are within the range of values used in the Wageningen B-series, between 0.3 and 1.05. Then, it should be checked to see whether the selected propellers meet the criteria for cavitation established in this study.
2.7.3. Cavitation criterion
The cavitation criterion used was based on 28 points extracted from 20%, 10%, 5% and 2.5% cavitation curves of the Burril diagram, in which is an acceptable limit of cavitation of up to 5%.
If the operating point of the propeller is below the curve relative to the percentage of cavitation of 5%, it meets the criterion of cavitation.
2.7.4. Electric balance
The electric balance is an estimate of all the electrical demand on board and its calculation was based on the standard NBR 7567 NOV/1982 (ABNT Citation1982).
First, the vessel's electrical system was divided into eleven groups of electrical charges divided according to their type of functionality and their degree of importance within the vessel. The electric balance was done by the group, separately, subdividing into four normal situations the vessel may be operating.
2.7.5. Calculation of total installed power and application service margins
Once the propulsion system arrangement is defined, the total installed power is calculated considering the transmission efficiency and the required power margin for roughness, fouling and weather:
• Sea margin: compensate the state of the sea and the age of the hull. The value adopted was 30%.
• Engine margin: the motor must operate in a condition lighter than its maximum operation. The value adopted was 10%.
• Rotation Margin: Applied to compensate for aging of the propeller and its increased loading due to accumulated fouling on the hull and adverse weather conditions. The value adopted was 5%.
2.8. Economical evaluation
Although the application of diesel-electric propulsion systems has potential when considering energy savings, it requires an additional investment cost compared to conventional propulsion systems.
To be able to compare the current and alternative design regarding their feasibility with an economical point of view, an investment evaluation is performed. The investment evaluation should only be seen as tentative since several parameters are excluded, such as additional commissioning costs and maintenance requirements.
A lot of assumptions were needed to be made. In particular, the discount rate and the lifetime of the investment are essential for the outcome in combination with the average fuel price.
The total installed power is multiplied with a cost factor in US$/kW which expresses the relationship between the price of the diesel engine and its power.
For the diesel system, the values of the engine chosen for the estimation of fuel consumption, described in Section 3.6.1, will be used. For the diesel-electric system, the installed power of the two gensets is added as a total power installed and is multiplied with a cost factor in US$/kW in the same way as for the diesel system. The cost of the gensets is obtained by using the cost of similar gensets to that chosen for the estimation of fuel consumption in Section 3.6.2, obtained from consults with dealers. Further, additional costs are added to account for the power electronics, i.e. for frequency converters and electrical motors.
where CFPI is the cost factor installed power; PI is the Installed power.
where: CFgenset is the cost factor generator sets; Pgenset is the installed power generator sets; Cpe is the cost power electronics.
The additional cost stated for the diesel-electric propulsion compared to current design only consider generators set, electrical motor and a frequency converter.
The total investment cost for the respective systems is then evaluated with the Net Present Value (NPV), which is commonly used when performing investment assessments. The NPV is calculated accordingly to the general formula in Equation (8):
(8)
(8)
where I is the initial investment cost; a is the annual cost reduction due to fuel saving; r is the discount rate; N is the expected lifetime of the investment.
The annual fuel savings will be obtained by comparing the fuel consumption of the diesel-electric system with the conventional diesel system, and the initial investment cost is the difference between the alternative propulsion system investment cost compared to the investment cost of the conventional system. It can be seen as an additional cost when comparing the different design alternatives.
For the case under consideration, the discount rate is assumed to be 8% and, as well as fuel savings, is assumed to be fixed value throughout this period. The discount rate was established based on the methodology of the Weighted Average Cost of Capital used by the Energy Research Company (EPE) as one of its assumptions in the elaboration of their supply and demand projections for energy resources in the Ten Year Energy Expansion Plan 2026 (EPE Citation2017).
2.9. Emissions of GHG – IPCC ‘Tier 1’ method
This study estimated GHG emissions using Tier 1 of the three methods provided by the IPCC.
According to the IPCC, ‘Fishery’ is classified as water-borne navigation and mobile combustion, which requires the use of mobile rather than stationary emission
Factors (IPCC et al. Citation2006). The Water-Borne navigation calculation is based on the amount of fuel burned and on emission factors for CO2, CH4, and N2O (Equation (9)) (IPCC et al. Citation2006):
(9)
(9)
The CO2 emission factor used in this study was 3.17 kg/kg of fuel, based on the default carbon dioxide emission factor for diesel oil and an energy content of 43.3 MJ/kg fuel (IPCC et al. Citation1997, Citation2006). The emission factors for CH4 (0.00015 kg/l) and N2O (0.00109 kg/l) were based on the estimated emission factors for European non-road mobile sources and machinery; diesel engines operating in inland waterways, (IPCC et al. Citation1997).
3. Results and discussion
3.1. Comparison of methods of estimating fuel consumption for the longline fleet: mean absolute percentage error
In method 1 the estimated SFC value was estimated for each vessel of a fishing trip by steps (Appendix 2).
In Method 2 a fixed value of 0.0963 l/hp-h was considered for all fishing stages, while in method 3 the SFC is the slope of the best the fit line of the fuel consumption and fishing effort relationship. The slope of this line provides an estimate of the SFC of the 0.032 l/hp.sea-hours, as shown in Figure .
Table shows the results according to each one of the three methods for each step of a fishing trip, as well as the comparison of the average fleet consumption of a fishing trip with the consumption value obtained in field research. The methods used were subjected to a comparative analysis based on the MAPE assessment.
Table 5. Comparison of estimated fuel with consumption obtained in the field research, in litres.
By comparing the results Method 3 presented the best results due to the need for less imprecise inputs for the formulation of this method.
The average consumption of a fishing trip estimated by the method 1 was the closest to the consumption obtained by field research. However, it presented a MAPE slightly higher than the method 3.
As fuel consumption was not considered in the stages of navigating between fishing grounds, the lowest value calculated by Method 3 is closer to real data. If we calculate the average consumption of a fishing trip through Equation (Equation3(3)
(3) ), considering the average engine power of the vessels (223 hp) and the average number of sea days (20 days), the estimated value would be 3432 L. In this case, the consumption in the navigating between the fishing grounds would be included.
In the access to the fishing grounds steps, Method 1 obtained results close to those of Method 2. This indicates the equivalence between the product of the variable by the SFC, with the fixed value of 0.0963 l/hp-h adopted in Method 2.
The high value of consumption estimated by Method 2 in the capture stages (setting, waiting and hauling) shows that, in the case of bottom longline fishing, the SFC of 0.0963 l/hp-h used is overestimating the consumption in these stages. In this case, the calculated value of 0.032 l/hp.sea-hours in Method 3 seems to be the most appropriate.
In the case of fishing gears requiring higher levels of power and greater fuel consumption at the time of fishing, as is the case of trawling, the value adopted by Method 2 would be suitable.
The calculation methodology used by the Brazilian Government of a single SFC for all fisheries can lead to imprecise results since each fishing method has its own operational profile, which will influence the fuel consumption (Suuronen et al. Citation2012). The most appropriate would be to perform calculations based on the methodology of Method 3 for samples of different types of fisheries, in order to obtain a suitable SFC value for each one and, consequently, more precise fuel consumption estimates.
3.2. Propeller selection and calculation of total installed power
3.2.1. Effective power and optimum propeller selection
Considering a service speed of six knots, the obtained Rt value was 2.72 kN, resulting in a Pe = 8.4 kW. With the values of w and t, it is possible to calculate the propeller thrust and thrust power for the different values of propeller diameters tested.
The optimal propellers selected according to the procedures adopted in this study, as well as their main parameters, are presented in Table . These results were obtained considering the nominal propeller condition, that is, the optimum rotation condition found for the speed of service of the vessel.
Table 6. Optimum results for selected propellers.
The propulsive efficiency (ηd) is a function of the propeller diameter. With the increase of D, an increase in ηd is observed from 41% to 51%.
All ten propellers, with different P/D and blade area ratio values of each selected diameter (between 0.6 and 0.85 m) were tested in the cavitation criterion.
Considering a cavitation limit of 5% in the blades, only the optimum results of propellers with a diameter of 0.6 m and 0.65 m were rejected in the cavitation criterion. In this way, the diameter selected for the propeller was 0.75 m. This diameter value offers good efficiency (55%) and its size is adequate, representing 50% of the draft length, besides having an acceptable limit of cavitation.
With this, the selected propeller was the B4-40, with four blades and P/D ratio of 0.9, as it was the one with the highest efficiency between the P/D ratios evaluated.
The power supplied to the propeller by the drive shaft was calculated by the ratio:
3.3. Estimate of electrical demand
Table presents a summary of the electrical power consumption of each group, for each operating condition of the vessel:
Table 7. Electrical Balance of the consumer groups for each operating condition.
3.4. Estimate of the total installed power: conventional diesel system
After selecting the ideal propeller, the installed power estimate is made considering the transmission efficiency (ηt), the power required to supply the electric demand and the service margins. The considered transmission efficiency was 0.94, obtained by multiplying the efficiency of the transmission shaft by the efficiency of the gearbox, 0.99 and 0.95 respectively (El-Gohary and El-Sherif Citation2004; Molland et al. Citation2011; Notti et al. Citation2012; Logason Citation2015).
Therefore, the total engine power required at the vessel's service speed prior to the application of the service margins is:
Power Required at Service Speed: = 19 kW
Required Electrical Demand: 10.4 kW
Installed power = 19 kW + 10.4 kW = 29.4 kW
Adding the extra power margins to the installed power, the total installed power is 42 kW or 57 hp.
3.5. Estimate of the total installed power: diesel-electric system
The same vessel and propeller are considered, with the same geometric characteristics and operating parameters, differentiating only in its propulsion system equipment. With this, the powers Pe, Pt and Pd continue to be the same ones calculated for the conventional diesel system.
However, the additional electrical equipment between the diesel engine and the propeller increases the losses in the transmission system due to the multiple transformations of energy in the system.
The total efficiency is a product of the efficiencies of typical equipment of an electric propulsion system in series:
(10)
(10)
The typical efficiency values of each component of the system are as follows (Adnanes Citation2003; Balashov Citation2011; MAN Citation2012b):
Generator: 95–97%;
Switchboard: 99.99%;
Transformer: 99.1–99.7%;
Frequency converter: 98–99%;
Electric motor: 95–97%
Required power for the generators: 21.3 kW
Required electrical demand (Electrical Balance):10.4 kW
Installed power = 21.3 kW + 10.4 kW = 31.7 kW
Finally, with the application of service margins, the required power in the generator sets was 45.7 kW. As the proposed configuration for this system has two generator sets, the required power per generator is:
PGe = = 22.9 kW or 30.7 hp.
3.6. Estimate and comparison of fuel consumption of different propulsion systems for a typical longliner fishing vessel
3.6.1. Diesel system
In this case, an engine MWM model MWM – D229 4 was chosen (MWM Citation2017) :
Continuous Power: 54 kW (72.4 hp)
||RPM: 2500
SFC 75% load: 0.21 l/hp-h
Power Percentage = Required Power/Installed Power
Thus, the fuel consumption is:
(11)
(11)
where Preq is the power required in a given step; Pi is the power of selected motor = 54 kW (72.4 hp); t is the operating time of a vessel in a given step, (h).
During the capture phase, the average number of launching fishing was considered which includes the setting, waiting and hauling steps, multiplied by the average number of fishing days.
The power required at each stage of a typical fishing trip was calculated using the same methodology as in Section 2.7. However, to estimate Rt and Preq based on (Holtrop and Mennen Citation1982) the average vessel speeds at each stage obtained in the field survey were used.
The required power was considered as the sum of the power required for propulsion with the power required to meet the electric demand of the vessel at each fishing step. In addition, the SFC of 0.21 l/hp-h was considered for all fishing stages.
3.6.2. Diesel-electric system
Two Cummins Onan generators were selected to supply the required power of 51 kW in the diesel-electric system. Its standard features are presented in Table .
Table 8. Selected marine generator set features.
The calculation of the consumption for each step of a typical fishing trip was made taking into account the hourly consumption claimed by the manufacturer (Table ), as well as the percentage of power required of each generator set in each step:
(12)
(12)
The percentage of power required in each generator was calculated by the ratio
, where Pinst represents the generator power of 27.5 kW.
The fuel consumption of the generators at each step will depend on their operating load as well as the length of time the vessel will be in that particular step.
Figure shows the percentage of the maximum load that the generators will operate under in each step, in order to optimise their specific fuel consumption.
The final estimate of the fuel consumed in a typical fishing trip for both diesel and diesel-electric system is shown in Table .
Table 9. Calculation of consumption by stages on a typical fishing trip.
3.6.3. Comparison of the energy consumption of different propulsion systems
The estimate of the total installed power of 57 hp for the diesel system indicated that the fleet is using engines much larger than necessary, reflecting higher fuel consumption.
The results showed that, for the typical vessel with a 72.4 hp engine, fuel consumption was 45% lower than the average fleet consumption obtained in field research.
However, the operational profile observed in the longline fleet indicated the possibility of significant savings by using diesel-electric propulsion. The vessels remain, on average, 83% of the fishing trip time in the capture phase with their engines operating at low loads. The step of hauling fishing gear represents only 41% of the total time of a fishing trip.
In the case of a typical vessel with 72.4 hp diesel engine, the load on the engine will be 26% for 41% of the time, resulting in fuel consumption 70% higher than that estimated for the diesel-electric system at the hauling step.
Thus, despite the slightly higher consumption in the access steps, the total consumption of the diesel-electric system was 14% lower than the estimated consumption of the diesel system and 23% lower than the consumption estimated by method 3 for the analysed fleet, optimising the overall operational performance of the vessel.
The reduction in total consumption occurred due to the lower consumption observed in the capture stage, where the vessel operates at reduced speeds allowing the engine to operate closer to its ideal design point.
3.7. Economical comparison
The following values included in the cost comparison are presented as follows:
For the total cost of installed power, the cost factor of 739.00 US$/kW is used, and accounts for diesel engine chosen: MWM – D229 4; Continuous power = 54 kW and price equal to US$ 39,900.00 obtained from the manufacturer.
For the total cost of installed power generator sets, the cost factor of 1652.00 US$/kW is used and accounts for generator chosen: Cummins: 27.5 MDKBT; Electrical Power = 27.5 kW; with an average price of about R$ 145,00.00 obtained from consults with dealers. The price of the generator sets is converted to US$ 45,420.00 using the monthly average exchange rate for 2017 of 3.193 R$/US$, taken from (IPEADATA Citation2018).
For the cost of power electronics, including frequency converter and an electrical motor, the values of the following equipment were chosen as reference:
Electrical Motor with Frequency converter: Elco EP – 40; Continuous power = 17 kW and price equal to US$ 14,990.00, obtained from the manufacturer.
When implementing these values, the following initial investment cost for diesel and diesel-electric systems is estimated (Table ):
Table 10. Estimated investment cost for the two propulsion systems.
For calculations of fuel costs per fishing trip, the price of US$ 1.04 for diesel was considered, relative to the average monthly price of 2017/2018 practiced in the State of Rio de Janeiro (ANP Citation2018). Further, the lifetime of the investment is stated for 15 years, where the calculation of annual fuel costs is done considering a total of 12 fishing trips per year.
Table reveals that diesel-electric system should be saving about 14% with regards to the annual fuel costs compared to the diesel system.
Table 11. Total diesel consumption and cost of a typical trip for the two propulsion systems.
Applying the NPV method (Equation (Equation8(8)
(8) )) and considering the discount rate, average fuel price and expected lifetime of the investment from data, the minimum time required to recover the additional investment cost when comparing the different design alternatives is about nine years. Figure shows when it is feasible to make the additional investment to the diesel system.
Figure 4. Breakeven for additional investment cost of diesel-electric system compared to diesel system.
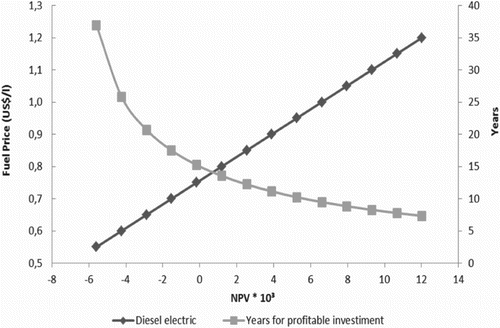
As can be seen, the diesel price has a huge impact on the NPV parameters and the breakeven average fuel price obtained is about US$ 0.76 per litre.
It should be noted that maintenance costs of the different systems are excluded, if these costs were considered, the diesel-electric system would be even more economical, since its maintenance requirements are lower due to flexible and smooth operating, avoiding jerky torque and speed change (Balashov Citation2011).
3.8. GHG emissions
Based on estimated fuel consumed using method 3, the bottom longliner fleet emitted a total of 105 tonnes of carbon dioxide equivalent (CO2e). The average fleet emission (Table ) for all fishing steps was approximately 6.2 tonnes of CO2e, where the hauling step was responsible for the largest amount of emissions (2.86 tonnes of CO2e).
Table 12. GHG emissions by steps for a fishing trip.
The use of diesel-electric propulsion in a typical vessel would theoretically decrease the emissions by approximately 23% or 1.4 tonnes of CO2e compared to average fleet emissions.
Considering the replacement of all vessels of the fleet by a typical diesel-electric vessel, the total emissions would be 81 tonnes of CO2e. This would represent a reduction of approximately 24 tonnes of CO2e emitted compared to the current estimates of 105 tonnes of CO2e.
Comparing the two propulsion systems in a typical vessel, this reduction would be 14%. The low average engine load during line setting and hauling steps leads to higher fuel consumption and, consequently, overall higher emission of GHG. Only in these phases can it be seen that 0.91 tonnes of CO2e could be avoided by using diesel-electric propulsion.
Conclusion
The results showed that Method 3 was the one that presented the best result among the evaluated methods. It showed that only with total fuel consumption data on a fishing trip and with data on power and operating time at each stage is it possible to make more precise estimates.
By means of the engine sizing methodology, carried out by estimating the installed power in the diesel and diesel-electric systems, fuel use and the GHG emissions from the longliner fishing vessel was assessed.
This process aimed to evaluate if the fishing vessels are using engines with adequate power for the fishing operations, in order to help fishers achieve low fuel consumption with a more rational use of energy. This will lead to the reduction of power installed, selecting an engine suitable for the operational profile of longline fishing or the use of other propulsion technologies, such as the diesel-electric system.
The results showed that the engines used are much larger than needed, indicating a potential for reduction in fuel consumption just with the installation of smaller engines.
Comparing the use of diesel and diesel-electric systems in a typical vessel, there was a 14% lower consumption for the diesel-electric system, due to the lower consumption observed in the setting, waiting and hauling steps. In these steps, the use of the diesel-electric system in which the vessel operates at reduced speeds is justified.
When the economical evaluation is considered it can be seen that today with the current diesel prices investment in diesel-electric system would be feasible. However, for prices lower than US$ 0.76/l and keeping all other parameters constant such as discount rate, expected lifetime of the investment and annual cost reduction due to fuel savings, investment in diesel-electric would not be feasible.
The results also showed that this reduction in fuel consumption by using a diesel-electric system indicated a potential reduction of approximately 0.8 tonnes of CO2e in total GHG emissions, due to the considerable reduction in the setting and hauling steps. In these two steps alone, almost a tonnes of CO2e could be avoided.
Acknowledgements
The authors wish to acknowledge the Fishing Institute Foundation of the State of Rio de Janeiro and crew of the longliner fleet for cooperation and assistance in the field research and data collection. R.F.Bastos is grateful to Agência Nacional do Petróleo (ANP) for his Doctoral Scholarship.
Disclosure statement
No potential conflict of interest was reported by the authors.
ORCID
David Alves Castelo Branco http://orcid.org/0000-0002-9053-7033
Notes
1 The FIPERJ is an agency associated to Secretary of State for Regional Development, Supply and Fishing of Rio de Janeiro. For further information, see (FIPERJ Citation2017).
References
- ABNT. 1982. NBR 7567: Execução de Balanço Elétrico.
- Adnanes AK. 2003. Maritime electrical installations and diesel electric propulsion. Oslo: ABB AS Marine.
- ANP. 2018. Agência Nacional do Petróleo, Gás Natural e Biocombustíveis - Série histórica do levantamento de preços e de margens de comercialização de combustíveis. [accessed 2018 May 29]. Available from: http://www.anp.gov.br/precos-e-defesa/234-precos/levantamento-de-precos/868-serie-historica-do-levantamento-de-precos-e-de-margens-de-comercializacao-de-combustiveis
- Balashov S. 2011. Design of marine generators for alternative diesel-electric power system. Faculty of Technology – Department of Electrical Engineering: Lappeenranta University of Technology.
- Basurko OC, Gabiña G, Uriondo Z. 2012. Energy audits of fishing vessels: lessons learned and the way forward. Second International Symposium on Fishing Vessel Energy Efficiency E-Fishing; Vigo, Spain. p. 7.
- Basurko OC, Gabiña G, Uriondo Z. 2013. Energy performance of fishing vessels and potential savings. J Clean Prod. 54:30–40. doi: 10.1016/j.jclepro.2013.05.024
- Bernitsas M, Ray D, Kinley P. 1981. KT, KQ and Efficiency Curves for the Wageningen B-series Propellers. Department of Naval Architecture and Marine Engineering, College of Engineering, University of Michigan: University of Michigan.
- Brasil. 2011. Ministério da Pesca e Aquicultura. Instrução Normativa Interministerial n° 10, de 14 de Outubro de 2011. Diário Oficial da União.
- Onan C. 2017. Folha de Especificações: Marine generator set Quiet DieselTM Series 22.5/27.5 QD Model MDKBT. [accessed 2017 January 22]. Available from: http://www.cumminsonan.com.br/maritimo_modelo_gerador_27-5.asp.
- Driscoll J, Tyedmers P. 2010. Fuel use and greenhouse gas emission implications of fisheries management: the case of the new England Atlantic herring fishery. Mar Policy. 34:353–359. doi: 10.1016/j.marpol.2009.08.005
- El-Gohary MM, El-Sherif HA. 2004. MR351 - Ship Propulsion Systems Third Year. Faculty of Engineering Naval Architecture and Marine Engineering Department: Alexandria University.
- EPE. 2017. Plano Decenal de Expansão de Energia 2026. Brasília (Brasil): Empresa de Pesquisa Energética – EPE.
- Fernández AS, Rodríguez DR, García MS, Iturralde MM, Gandoy JD. 2010. Potential of hybrid systems with permanent magnet motors for propulsion improvement on surface longliners. First International Symposium on Fishing Vessel Energy Efficiency E-Fishing; May; Vigo, Spain.
- FIPERJ, editor. 2013. Diagnóstico da Pesca do Estado do Rio de Janeiro. Projeto de Monitoramento da Pesca no Estado do Rio de Janeiro – Estatística Pesqueira. Niterói: FIPERJ, Fundação Instituto de Pesca do Estado do Rio de Janeiro.
- FIPERJ. 2017. Fundação Instituto de Pesca do Estado do Rio de Janeiro. [accessed 2017 Jan 6]. Available from: http://www.fiperj.rj.gov.br/.
- Gabiña G, Basurko OC, Notti E, Sala A, Aldekoa S, Clemente M, Uriondo Z. 2016. Energy efficiency in fishing: are magnetic devices useful for use in fishing vessels? Appl Therm Eng. 94:670–678. doi: 10.1016/j.applthermaleng.2015.10.161
- Geertsma RD, Negenborn RR, Visser K, Hopman JJ. 2017. Design and control of hybrid power and propulsion systems for smart ships: A review of developments. Appl Energy. 194:30–54. doi: 10.1016/j.apenergy.2017.02.060
- Gulbrandsen O. 2012. Fuel savings for small fishing vessels: a manual. Rom: FAO.
- Holtrop J, Mennen GG. 1982. An approximate power prediction method. Int Shipbuild Prog. 29:166–170. doi: 10.3233/ISP-1982-2933501
- Hua J, Wu Y. 2011. Implications of energy use for fishing fleet – Taiwan example. Energy Policy. 39:2656–2668. doi: 10.1016/j.enpol.2011.02.034
- IPCC, Eggleston S, Buendia L, Miwa K, Ngara T, Tanabe K. Task Force on National Greenhouse Gas Inventories. 2006. 2006 IPCC guidelines for national greenhouse gas inventories. Kanagawa (Japan): Institute for Global Environmental Strategies.
- IPCC, Houghton J, Filho LM, Lim B, Treanton K, Mamaty I, Bonduki Y, Griggs D, Callender B. 1997. Revised 1996 IPCC guidelines for national greenhouse gas inventories. Paris: IPCC/OECD/IEA.
- IPEADATA. 2018. Instituto de Pesquisa Econômica Aplicada - Base de dados macroeconômicos. [accessed 2018 May 29]. Available from: http://www.ipeadata.gov.br/Default.aspx.
- Karlsen L. 2012. Energy saving aspects for fishing operations. Second International Symposium on Fishing Vessel Energy Efficiency E-Fishing; Vigo, Spain. p. 8.
- Lewis EV. 1988. Principles of naval architecture. 2nd revision, 3rd ed. Jersey City: Society of Naval Architects and Marine Engineers.
- Logason AAK. 2015. Feasibility study of hybrid propulsion systems for long-liner fishing vessels [Master’s Thesis in the International Master’s Programme in Naval Architecture and Ocean Engineering]. Department of Shipping and Marine Technology Division of Marine Technology. Göteborg, Sweden: Chalmers University of Technology.
- MAN. 2012a. Basic principles of ship propulsion. Augsburg: Man Diesel and Turbo.
- MAN. 2012b. Diesel-electric propulsion plants: A brief guideline how to engineer a diesel-electric propulsion system. Augsburg: MAN Diesel & Turbo.
- Molland AF, Turnock SR, Hudson DA. 2011. Ship resistance and propulsion: practical estimation of ship propulsive power. New York: Cambridge University Press.
- Muir JF. 2015. Fuel and energy use in the fisheries sector approaches, inventories and strategic implications. Rome, Italy.
- MWM. 2017. MWM Motores Diesel. [accessed 2017 Jan 22]. Available from: http://www.mwm.com.br
- Notti E, Sala A. 2012. On the opportunity of improving propulsion system efficiency for Italian fishing vessels. Second International Symposium om Fishing Vessel Energy Efficiency E-Fishing; Vigo, Spain.
- Notti E, Sala A, Martinsohn J, Damalas D, European Commission, Joint Research Centre, Institute for the Protection and the Security of the Citizen. 2012. Effects of engine replacement on the fuel consumption reduction in fisheries. Information Collection in Energy Efficiency for Fisheries (ICEEF), CNR-ISMAR; Ancona, Italy.
- Park J-A, Gardner C, Chang M-I, Kim D-H, Jang Y-S. 2015. Fuel Use and greenhouse Gas emissions from offshore fisheries of the Republic of Korea. PLOS ONE. 10(8):e0133778.
- Parker RWR, Tyedmers PH. 2015. Fuel consumption of global fishing fleets: current understanding and knowledge gaps. Fish Fish. 16:684–696.
- Sala A, de Carlo F, Buglione G. 2010. Coriolis fuel mass flow metering for fishing vessels. First International Symposium on Fishing Vessel Energy Efficiency E-Fishing; Vigo, Spain. p. 5.
- Schau EM, Ellingsen H, Endal A, Aanondsen SA. 2009. Energy consumption in the Norwegian fisheries. J Clean Prod. 17:325–334. doi: 10.1016/j.jclepro.2008.08.015
- Suuronen P, Chopin F, Glass C, Løkkeborg S, Matsushita Y, Queirolo D, Rihan D. 2012. Low impact and fuel efficient fishing – looking beyond the horizon. Fish Res. 119–120:135–146. doi: 10.1016/j.fishres.2011.12.009
- Thrane M. 2004. Energy consumption in the Danish fishery: identification of key factors. J Ind Ecol. 8:223–239. doi: 10.1162/1088198041269427
- Tyedmers P. 2001. Energy consumed by North Atlantic fisheries. In: Zeller D, Watson R, Pauly D, editors. Fisheries impacts on North Atlantic ecosystems: catch, effort, and national/regional data sets. Vol. 9. The Fisheries Centre Research Reports. Vancouver: University of British Columbia; p. 12–34.
- Van Beek T, Van der Steenhoven S. 2005. Fishing vessels, fishing technology & fisheries. International conference; 13–14 April; Newcastle, UK. London: Royal Institution of Naval Architects, School of Marine Science and Technology.
- Wilson JDK. 1999. Fuel and financial savings for operators of small fishing vessels. Rome: FAO.