Abstract
The current research was a comparative study of reinforced ceramic particles and mixing proportion on the microstructural and slurry erosive characteristics for fabricated composites. Dual reinforced particle composites were fabricated by adding combinations of numerous wt% of titanium carbide (TiC) and zircon sand (ZrSiO4) to aluminium die-casting (ADC12) alloy matrix composites. ADC12 alloy composite with titanium carbide and zircon sand samples were also prepared for comparison of dual reinforcement particles on microstructural features and wear behaviour in various slurry concentrations. Titanium carbide (44–60 µm) and zircon sand (20–33 µm) particles are reinforced in the alloy by the two-step stir-casting method. Slurry erosion wear study reveals that the dual particle reinforcement enhances the wear resistance as compared to single-particle reinforcement if mixed in a definite proportion. The study also indicates that a combination of 15% reinforcement of titanium carbide and zircon sand particles in the ratio of 10 and 5 wt% into the composite exhibits better microhardness and wear resistance as compared to other combination.
Introduction
Aluminium alloy matrix composites (AMCs) with multiple reinforcements or hybrid AMCs are finding increased applications in various sectors because of improved mechanical and tribological properties and hence are better substitutes for single reinforced composites (Kumar, Panwar, et al. Citation2012; Singh et al. Citation2012). Aluminium and its alloys when reinforced with hard ceramic particulates impart a combination of properties not achievable in either of the constituents individually. Aluminium alloys provide a good matrix for the development of particulate reinforced composites owing to their low density, high specific strength, high corrosion resistance, ease of manufacture, and low cost (Kumar, Sharma, et al. Citation2012). In recent years many processing techniques have been developed to prepare particulate reinforced aluminium matrix composites. Among the variety of processing techniques available for particulate or discontinuous reinforced metal matrix composites, stir casting is one of the methods accepted for the production of large quantity composites. It is attractive because of simplicity, near net shaping, flexibility and is most economical for large size components to be fabricated. However, it suffers from poor distribution of the reinforcement particles in the aluminium matrix composite. These problems become especially significant as the reinforcement size decreases due to greater agglomeration tendency and reduced wettability of the particles with the melt (Desale et al. Citation2012; Thangarasu et al. Citation2012; Asuquo et al. Citation2013). The dendritic growth and porosity also exist in stir cast composites. Therefore, innovations in the processing method of stir casting are of great importance. Stir-casting process is modified by various researchers to achieve the desired properties in the composite (Lloyd and Rulfs Citation2008; Henein et al. Citation2011; Singh and Mer Citation2013; Rajan et al. Citation2014; Valdez et al. Citation2016). Two-step stir casting has an advantage in terms of promoting wettability, reduction of porosity, and homogeneous distribution of particles. Moreover, the microstructure is also refined which provide better mechanical properties (Lloyd and Rulfs Citation2008). In earlier reported two-step stir-casting method the mixing of particle is done in semisolid melt by manually stirring with steel rod, which is cumbersome process and also the agglomeration of fine particles occurs. Moreover, mixing is not efficient in reinforcement of fine particle as total volume occupied is increased and semisolid melt solidifies before mixing is completed. ZrO2–fibre-reinforced ZrO2–SiO2 aerogel composite with superior thermal and mechanical properties is fabricated via a rapid gelation method (Mazahary and Shabani Citation2012). In the present study the conventional stir cast processing is bifurcated. In the first step the melting and mixing is done properly as is being followed in stir casting and melt is solidified in crucible. In the second step the solidified mass is re-melted for stirring process followed by casting. In recent years, numerous research works have been reported on the production of multiparticle-reinforced or hybrid AMCs reinforced with TiC, ZrSiO4, and graphite or in combination of the above, but very limited research work has been done on the reinforcement of dual hard particles (Garcia et al. Citation2010; Assadi et al. Citation2013). Moreover, no work is reported on the slurry erosion wear behaviour of aluminum alloy-reinforced composites fabricated by stir casting. It was investigated by the room temperature sliding wear behaviour of reinforced aluminium matrix hybrid composites under both the dry and lubricant conditions. The study shows that wear behaviour is related to the fiber orientation of alumina fiber in both dry and lubricated conditions. SiO2, Al2O3, and B2O3 additives were used to synthesise an alumino-boro-silicate glass on the grain boundaries with the long-term goal to enhance fracture resistance (Viswanathan and Mandal Citation2013). Suresh kumar et al. studied the dry sliding wear behaviour of Al matrix composites reinforced with zircon sand and SiC particulate up to 12%. The study shows that LM-13 alloy/TiC/ZrSiO4 hybrid composite exhibited better wear resistance, but the optimal addition of graphite particles is necessary (Kumar, Panwar, et al. Citation2012). Metal–metal and metal abrasive wear tests revealed that wear resistance of the composites increased with increasing Mg addition (Bobic et al. Citation2010). The present study is aimed to analyse the combined effect of both the hard particles reinforcement in aluminium alloy composites fabricated by the modified two-step stir casting. Our study is mainly focused on investigating the microstructural features and wear properties of dual particle reinforced (titanium carbide and zircon sand) ADC12 alloy, which has not been studied so far.
Material selection and experimental processes
In the present investigation, the well-known piston alloy ADC12 is used as the matrix material and high purity titanium carbide (TiC) and zircon sand (ZrSiO4) as the reinforcement. ADC12 alloy was obtained in the form of ingots. The chemical compositions of the ADC12 alloy are given in Table . The various wt% of titanium carbide and zircon sand was successfully fabricated into ADC12 alloy through stir casting. The required quantity of ADC12 alloy was taken in a graphite crucible and melted in an electric furnace. The temperature of the melt was raised to 850°C. This molten metal was stirred using a graphite impeller at a speed of 630 rpm. At this, 630-rpm vortex is created in the melt, which facilitates to suck the reinforced particles inside the melt. The ceramic particles used as the reinforcement were taken in defined proportion and mixed properly by spatula. Particles prior to mixing were preheated at 4500°C to off the moisture. After the formation of vortex in the melt, the particles were charged inside the vortex at the rate of 20–25 g/min into the melt during stirring by impeller with the help of funnel kept on the top of vortex. Zircon sand and titanium carbide particles of fine grade were selected for the present work. After mixing of particles, the melt slurry is allowed to solidify in a graphite crucible at room temperature conditions. After solidification, the mixture is again re-melted in a furnace to ensure that slurry is in fully liquid condition and then melt is stirred with impeller for 12–15 min. A similar type of synthesis of the composite was reported earlier by various researchers (Henein et al. Citation2011; Singh and Mer Citation2013; Rajan et al. Citation2014). During the production of composite, the amount of ADC12 alloy, stirring duration and the position of stirrer in the crucible were kept constant to minimise the contribution of variables related to stirring on the distribution of the second phase particles (Viswanathan and Mandal Citation2013). In the earlier work, it was observed that 15% reinforcement of zircon sand-reinforced composite has given better property, so we have restricted the reinforcement up to 15% only (Patel et al. Citation2018). In order to compare and correlate the effect of dual particle reinforcement on the mechanical and tribological properties, three different composites containing a total of 15 wt% reinforcement in different proportion (as given in Table ) were fabricated and have been designated by alphabets.
Table 1. Chemical compositions of ADC12 aluminum alloy.
Table 2. Combination of reinforcements (numerous composites).
Results and discussion
Microhardness and density
The various reinforcement micron-sized particles were selected for fabricated into ADC12 alloy by stir casting. To improve the contact surface of the added particles into the matrix, which is more effective for aqueous and heat-resistive environments (Kumar, Sharma, et al. Citation2012). Microhardness has been measured at different phases of composite through developed composites, as shown in Table . Stiffness extent has been agreed on the embedded reinforced particles as well as in the vicinity of particles and matrix.
Table 3. Deviation of microhardness (Hv) and density of composites.
Erosive wear characteristics of the developed composites
Erosive wear test was performed, 1-h duration at 500, 1000, 1500 rpm using coarse particle sand at numerous (25%, 50% and 75%) concentrations, the following observations were through. Accordingly, Figure (a–c) shows the loss of materials at 500, 1000 and 1500 rpm at several slurry concentrations. The comparison of slurry erosion wear of the composites (A, B and C) at different speeds (500, 1000 and 1500 rpm) and different slurry concentrations (25%, 50% and 75% by weight) on coarse sand illustrates that wear rate increases almost linearly with increased speeds and slurry concentrations. Because of martensitic phase generation into matrix shows a refined solidity and better wear resistance (Hou et al. Citation2018). Also, the coating layer developed in high-temperature water will improve wear resistance (Rana et al. Citation2014). It was observed that at low speed and low slurry concentration, all the composites exhibit almost similar types of wear behaviour. The wear curve trend shown in Figure is almost similar due to speeds and slurry concentration, but it is higher for composites B and C, but lower for composite ‘A’. Because wear coefficients increase linearly with the ratio of abrasive to alloy hardness (Behler et al. Citation2018). The outcomes resulted improved microhardness and wear resistance of developed composites due to better interfacial bonding between alloy and reinforcements.
Figure 1. The mass loss of composites, (a) composite A, (b) composite B, (c) composite C at one-hour rotation in slurry (25%, 50% and 75% by weight) at 500, 1000 and 1500 rpm., respectively.
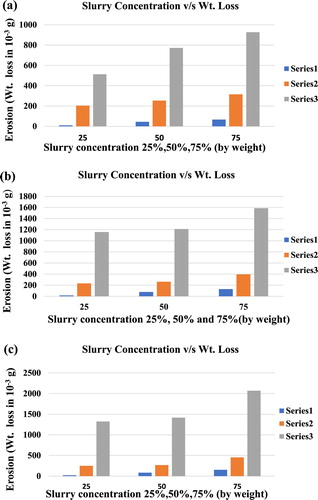
Figure 2. SEM micrograph of the wear surface of composite A, (a) 500 rpm, (b) 1000 rpm, (c) 1500 rpm and slurry concentrations at different magnifications.
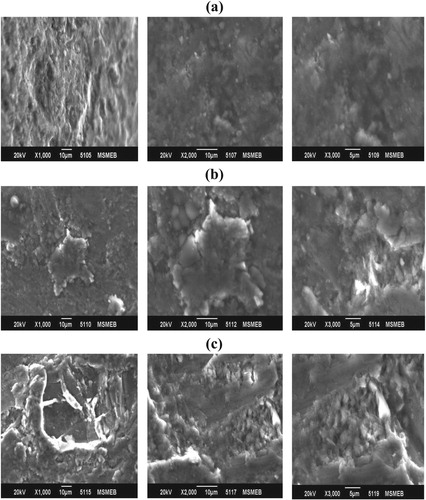
On the other hand, the composite has equal quantities of both reinforcements. , Composite ‘B’ provides improved wear properties as the same as composite ‘C’. Also, the composite ‘A’ shows decreased wear rate as compared to composites B and C. Dual particles have different roles in the matrix, titanium carbide particles refine the eutectic silicon, whereas zircon sand particles afford good bonding features to the matrix. By evaluating the wear of composites, it is clear that reinforcement mixed in a definite proportion is only effective for enhancing wear resistance. Titanium carbide particles show better reinforcement for wear resistance in the aluminium alloy matrix. Also, they exhibit better wear resistance due to the microhardness developed in the interface between the matrixes.
Morphological analysis of the eroded surface
The secondary electron microscopy (SEM) micrograph of the eroded surface of composite ‘A’ at different rpm and slurry concentrations is shown in Figure . At 500 rpm, composite B shows high wear rate, as shown in Figure (a). Long and wider craters along the erosion wear are observed in the worn particles. At 1000 rpm wear resistance was decreases with inhomogeneous TiCp (titanium carbide particles) distribution into matrix so matrix seems to be softer shown in Figure (b) excessive as craters become deeper. Flankes wear was visible over eroded surface, because life prolongation of hard metals and nano-particle reinforced develop matrix up to 3 times (Zhao et al. Citation2018) and this mechanism was originated to friction-caused flank wear (Ghosh et al. Citation2014). The nanostructures produced in the near-surface layer are mostly collected on low angle boundaries (Ramachandra and Radhakrishna Citation2007). if hard particles were scattered over the matrix, the matrix becomes harder, as shown in Figure . At 1500 rpm, the loss of mass is excessive due to the swelling of the striking slurry on the composite. The subsurface cracks are visible in Figure (c). The crack circulation was increased along the increasing rpm and slurry concentrations (Hou et al. Citation2018), which result in material removal by delimitation. Several microcracks are also propagating, resulting in the removal of material by delimitation. The rupture of the mechanically mixed layer enhanced slurry erosive wear. So, with the temperature decreasing from room to low temperature, the wear mechanism changes from abrasive, adhesive and surface fatigue wear (Ramachandra and Radhakrishna Citation2006). The slurry was a mixture of melted and compact lubrication under runny lubrication state (Saraswathi et al. Citation2006). Loss of materials increases with the increase in rpm respectively, but at 75% slurry concentration loss of materials decreases as compared to 50% slurry concentrations. Because the high-speed slurry particles are collided to each other, the striking rate of slurry particles decreases on the test sample. The wear rate also decreases from composite ‘B’, but composite ‘A’ has better wear resistance; in this way, composite A has better wear resistance than composites B and C. The SEM images of slurry erosion surface of composite ‘B’ at different slurry concentrations are shown in Figure . At 500 rpm, composite ‘B’ shows high wear rate, as shown in Figure (a). Long and wider craters along the erosion wear are observed with erosive particles of impact crater because under the sliding wear, the surface microstructure and abrasiveness undergo changes (Patel et al. Citation2018). At 1000 rpm, the particles of TiCp (titanium carbide particles) are reduced in distribution then the matrix seems to be softer, as shown in Figure (b). The subsurface and surface cracks are visible in Figure (c). The crack propagation is increased with the increasing rpm and slurry concentrations, which result in material removal by delimitation. The particles get deboned, which results in the increased loss of material. This investigation reported that first at 25–50% slurry concentration loss of materials increases with the increasing the rpm but at 75% slurry concentration loss of materials decreases as compared to 50% slurry concentrations because at high-speed striking slurry particles were collided with each other. Wear rate and friction are relational to each other during early sliding (Gupta et al. Citation2012). Unstable, non-adherent changeover oxides that form below 750°C act as a source of abrasive agents (Ramesh et al. Citation2009), so the impinging rate of slurry on composite decreases, and wear rate also some decreases from composite ‘B’ but composite ‘A’ has better wear resistance. In this way, composite ‘A’ has shown superior wear resistance than composite ‘B’. The SEM micrograph of slurry erosion surface of composite ‘C’ at different rpm and slurry concentrations of coarse silica quartz sand is shown in Figure . At 500 rpm, composite ‘C’ shows high wear rate, as shown in Figure (a). Long and wider craters along the sliding direction are observed with erosive particles of mechanically mixed layer. At 1000 rpm, the particles of adhesive and erosive mixed layer are shown in Figure (b). Material removal is excessive as craters become deeper. Flakes of wear surface are also visible in this micrograph. At 1500 rpm, the material removal is excessive due to high rpm. The subsurface and surface cracks are visible in Figure (c). The crack propagation is along the rpm and slurry concentrations, which result in material removal by delimitation. The particles get deboned, which results in an increased loss of material. In present research reported that at 25–50% slurry concentration loss of materials increases with the increasing the rpm but at 75% slurry concentration loss of materials decreases as compared to 25–50% slurry concentrations. These types of wear intensive care of a diesel engine were performed using this on-line wear fragments monitoring system (Ozguc Citation2018). In this way, composite A has better wear resistance than composites B and ‘C’. So, the potential applications of the wear model for the design of composites subject to dry abrasive rotary wear are debated (Karabay Citation2014).
Figure 3. SEM micrograph of the eroded surface of composite B, (a) 500 rpm, (b) 1000 rpm, (c) 1500 rpm and slurry concentrations at different magnifications.
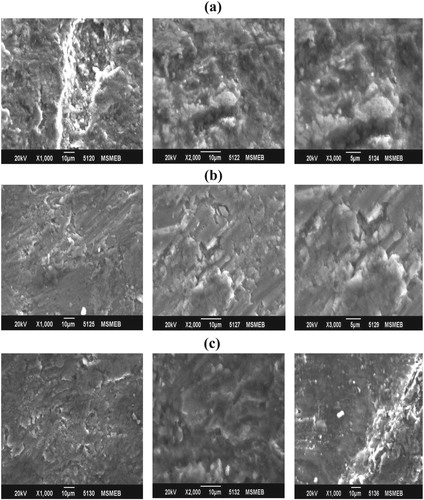
Figure 4. SEM micrograph of the wear surface of composite C, (a) 500 rpm, (b) 1000 rpm, (c) 1500 rpm and slurry concentrations at different magnifications.
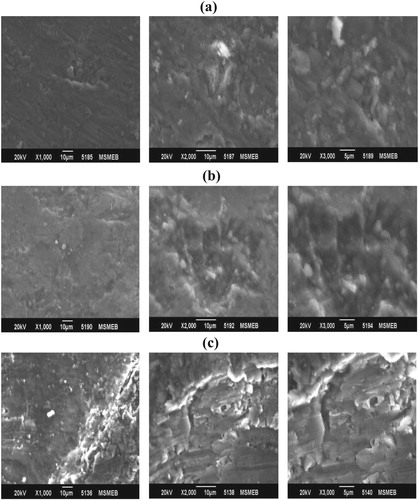
The composite with an equal amount of dual reinforcement, i.e. composite B provides superior wear properties than composite ‘C’. But, the composite A shows better wear resistance than composites B and C. Dual particles change the part in the matrix, titanium carbide particles improve the eutectic titanium, whereas zircon sand atoms provide good bonding features in the matrix, the SEM inside the wear scar specifies a grooving abrasion wear mechanism (Li et al. Citation1995). Composite A shows better wear resistance having a combination of 10% titanium carbide particles and 5% zircon sand particles, which was a new approach for wear state characterisation using inclusive wear debris features (Sivaprasad et al. Citation2008). The composite ‘B’ is grouped as 7.5% titanium carbide and 7.5% zircon sand particles, showing better wear resistance associated with composite ‘C’ which has 10% zircon sand and 5% titanium carbide particles.
After investigating the wear performance of composites, it is clear that dual particle reinforcement assorted in definite proportions is only operative for enhancing wear resistance. Titanium carbide particles are better reinforcement for wear behaviour than zircon sand particles. Composite ‘A’ exhibits better wear resistance and microhardness of the interface between the particle and matrix.
Conclusions
The present investigation is done to determine the influence of dual particle reinforcement on microhardness; microstructural analysis and slurry erosion wear behaviour of stir cast ADC12 alloy composites. The comparison of wear behaviour and microstructural micrographs of the fabricated composite, the following conclusion can be obtained:
Aluminium alloy (ADC12) composites of various wt% reinforced with TiC and ZrSiO4 particles can be successfully synthesised by the stir-casting method.
Microstructural observations show that the reinforced particles, TiC and ZrSiO4, are uniformly distributed in ADC12 aluminium alloy matrix and good interfacial bonding between particles and matrix.
The dual particle reinforcement enhances the better erosion wear resistance in ADC12 aluminium alloy composites.
The combination of reinforcement in aluminium alloy composites, 10% TiC and 5% zircon sand-reinforced particles composite (composite A), yields better wear resistance than composites ‘B’ and ‘C’ of ADC12 aluminium alloy composites.
The reinforcement up to 15 wt% yields better mechanical properties in ADC12 aluminium alloy composites. Increase in speed (rpm) and slurry particle size (coarse) increases the erosion and abrasion wear in ADC12 aluminium alloy composites.
The wear rate increases with an increase in slurry concentrations, first at 25–50% slurry concentration loss of materials increases with the increase in rpm but at 75% slurry concentration loss of materials decreases as compared to 25–50% slurry concentrations.
Acknowledgements
The authors acknowledge the MSME Department Laboratory, Maulana Azad National Institute of Technology (MANIT) Bhopal, India, for providing innumerable characterisation of as-synthesised samples.
Disclosure statement
No potential conflict of interest was reported by the authors.
References
- Assadi H, Alidokht SA, Abdollah-Zadeh A. 2013. Effect of applied load on the dry sliding wear behaviour and the subsurface deformation on hybrid metal matrix composite. Wear. 305(1–2):291–298. doi: 10.1016/j.wear.2012.11.043
- Asuquo LO, Bassey EN, Ihoms AP. 2013. Characteristics of zircon sand the effect on foundry casting. J Mech Ind Res. 1:27–32. doi: 10.12966/jmir.05.06.2013
- Behler KD, Marvel CJ, La JCS, Walck SD, Harmer MP. 2018. Observations of grain boundary chemistry variations in a boron carbide processed with oxide additives. Scr Mater. 142:106–110. doi: 10.1016/j.scriptamat.2017.08.033
- Bobic B, Vencl A, Arostegue S, Marinkovic A, Babic M. 2010. Structural, mechanical and tribological properties of A356 aluminium alloy reinforced with Al2O3, SiC and SiC + graphite particles. J Alloys Compd. 506(2):631–639. doi: 10.1016/j.jallcom.2010.07.028
- Desale GR, Gandhi BK, Jain SC. 2012. Slurry erosion of ductile materials under normal impact condition. Wear. 264:322–330. doi: 10.1016/j.wear.2007.03.022
- Garcia A, Valdez B, Schorr M, Zlatev R, Eliezer A, Hadad J. 2010. Assessment of marine and fluvial corrosion of steel and aluminium. J Mar Eng Technol. 9(3):3–9. doi: 10.1080/20464177.2010.11020235
- Ghosh S, Sahoo P, Sutradhar G. 2014. Wear characteristics optimization of Al–7.5%SiC metal matrix composite using Taguchi method. Adv Mater Manuf Charact. 4(2):93–99.
- Gupta AK, Prasad BK, Pajnoo RK, Das S. 2012. Effects of T6 heat treatment on mechanical, abrasive and erosive-corrosive wear properties of eutectic Al–Si alloy. Trans Nonferrous Metals Soc China. 22(5):1041–1050. doi: 10.1016/S1003-6326(11)61281-8
- Henein H, Kaftelen H, Unlu N, Goller G, Ovecoglu ML. 2011. Comparative processing-structure–property studies of Al–Cu matrix composites reinforced with TiC particulates. Composites Part A: Appl Sci Manuf. 42(7):812–824. doi: 10.1016/j.compositesa.2011.03.016
- Hou X, Zhang R, Fang D. 2018. An ultralight silica-modified ZrO2–SiO2 aerogel composite with ultra-low thermal conductivity and enhanced mechanical strength. Scr Mater. 143:113–116. doi: 10.1016/j.scriptamat.2017.09.028
- Karabay S. 2014. The effect of heat treatments on the solid-particle erosion behaviour of the aluminium alloy AA2014. Mater Technol. 48:141–147.
- Kumar S, Panwar RS, Pandey OP. 2012. Wear behavior at high temperature of dual-particle size zircon-sand-reinforced aluminum alloy composite. Miner Metals Mater Soc ASM Int. 44:548–1565.
- Kumar S, Sharma V, Panwar RS, Pandey OP. 2012. Wear behavior of dual particle size (DPS) zircon sand reinforced aluminum alloy. Tribol Lett. 47:231–251. doi: 10.1007/s11249-012-9983-y
- Li Y, Burstein GT, Hutchings IM. 1995. The influence of corrosion on the erosion of aluminium by aqueous silica slurries. Wear. 186–187:515–522. doi: 10.1016/0043-1648(95)07181-4
- Lloyd DCGGAG, Rulfs H. 2008. Influence of transient operating conditions on propeller shaft bearings. J Mar Eng Technol. 7(2):3–11. doi: 10.1080/20464177.2008.11020209
- Mazahary A, Shabani MO. 2012. Study on microstructure and abrasive wear behaviour of sintered Al matrix composite. Ceram Int. 38:4263–4269. doi: 10.1016/j.ceramint.2012.02.008
- Ozguc O. 2018. A new risk-based inspection methodology for offshore floating structures. J Mar Eng Technol. doi:10.1080/20464177.2018.1508804.
- Patel SK, Kuriachen B, Kumar N, Nateriya R. 2018. The slurry abrasive wear behavior and microstructural analysis of A2024–SiC–ZrSiO4 metal matrix composite. Ceram Int. 44(6):6426–6432. doi: 10.1016/j.ceramint.2018.01.037
- Rajan MHB, Ramabalan S, Dinaharan I, Vijay SJ. 2014. Effect of TiB2 content and temperature on sliding wear behavior of AA7075/TiB2 in situ aluminum cast composites. Arch Civil Mech Eng. 4:72–79. doi: 10.1016/j.acme.2013.05.005
- Ramachandra M, Radhakrishna K. 2006. Sliding wear, slurry erosive wear, and corrosive wear of aluminium/SiC composite. Mater Sci-Wroclaw. 24:333–341.
- Ramachandra M, Radhakrishna K. 2007. Effect of reinforcement of fly ash on sliding wear, slurry erosive wear and corrosive behaviour of aluminium matrix composite. Wear. 262(11):1450–1462. doi: 10.1016/j.wear.2007.01.026
- Ramesh AS, Keshavamurthy R, Channabasappa BH, Pramod S. 2009. Influence of heat treatment on slurry erosive wear resistance of Al6061 alloy. Mater Des. 30(9):3713–3722. doi: 10.1016/j.matdes.2009.02.009
- Rana RS, Purohit R, Sharma AK, Rana S. 2014. Optimization of wear performance of aa 5083/10 Wt. % SiCp composites using Taguchi method. 3rd International Conference on Materials Processing and Characterisation (ICMPC). Procedia Mater Sci. 6:503–511. doi: 10.1016/j.mspro.2014.07.064
- Saraswathi YL, Das S, Mondal DP. 2006. Influence of microstructure and experimental parameters on the erosion–corrosion behavior of Al alloy composites. Mater Sci Eng: A. 425(1):244–254. doi: 10.1016/j.msea.2006.03.083
- Singh V, Kumar S, Panwar RS, Panday OP. 2012. Microstructural and wear behaviour of dual reinforced particle (DRP) aluminum alloy composite. J Mater Sci. 47:6633–6646. doi: 10.1007/s10853-012-6551-7
- Singh C, Mer KKS. 2013. Abrasion wear characterization of Al–Al2O3 in-situ particulate composite synthesized in open hearth furnace with manually controlled stirring method. Int J Adv Mater Manuf Charact. 3:227–230.
- Sivaprasad K, Babu SK, Natarajan S, Narayanasamy R, Kumar BA, Dinesh G. 2008. Study on abrasive and erosive wear behaviour of Al 6063/TiB2 in situ composites. Mater Sci Eng: A. 498(1):495–500. doi: 10.1016/j.msea.2008.09.003
- Thangarasu A, Murugan N, Vijay SJ, Dinaharan I. 2012. Fabrication of AA1050/10wt.% TiC surface composite using friction stir processing. Proceedings of International Conference on Advanced Materials; Coimbatore. p. 277–281.
- Valdez B, Ramirez J, Eliezer A, Schorr M, Ramos R, Salinas R. 2016. Corrosion assessment of infrastructure assets in coastal seas. J Mar Eng Technol. 15(3):124–134. doi: 10.1080/20464177.2016.1247635
- Viswanathan S, Mandal D. 2013. Effect of re-melting on particle distribution and interface in SiC reinforced 2124Al matrix composite. Mater Charact. 86:21–27. doi: 10.1016/j.matchar.2013.09.008
- Zhao M, Yao X, Zhu YF, Jiang Q. 2018. Effect of the interface energy on the pressure-induced superheating of metallic nanoparticles embedded in a matrix. Scr Mater. 142:23–27. doi: 10.1016/j.scriptamat.2017.07.009