ABSTRACT
Toxoplasma gondii infection in the central nervous system commonly occurs among immunodeficient patients. Its prevalence is high in countries with a high burden of HIV and low coverage of antiretroviral drugs. The brain is one of the predilections for T. gondii infection due to its low inflammatory reaction, and cerebral toxoplasmosis occurs solely due to the reactivation of a latent infection rather than a new infection. Several immune elements have recently been recognized to have an essential role in the immunopathogenesis of cerebral toxoplasmosis. Although real-time isothermal amplification, next-generation sequencing, and enzyme-linked aptamer assays from blood samples have been the recommended diagnostic tools in some in-vivo studies, a combination of clinical symptoms, serology examination, and neuroimaging are still the daily standard for the presumptive diagnosis of cerebral toxoplasmosis and early anti-toxoplasma administration. Clinical trials are needed to find a new therapy that is less likely to affect folate synthesis, have neuroprotective properties, or cure the latent phase of infection. The development of a vaccine is being extensively tested in animals, but its efficacy and safety for humans are still not proven.
Introduction
Toxoplasma gondii infects approximately one-third of the world’s population [Citation1], including >13 million HIV-infected people, causing brain inflammation and various other diseases [Citation2]. The highest prevalence of T. gondii infection in the HIV-infected occurs in North Sudan 75% (62.2–87.8%), DR Congo 73.7% (59.7–87.7%), Ethiopia 72.4% (42.1–100%), Iran 60.7% (24.1–97.3%), Papua New Guinea 59.7% (52.5–66.8%), Brazil 57% (26.5–37.6%), Mexico 48.7% (41.5–55.8%), and Indonesia 43.6% (39.6–47.5%) [Citation3]. Hospital data from Bandung, Indonesia, revealed that the prevalence of cerebral toxoplasmosis was 52/404 (12.9%) among all central nervous system (CNS) infections in 2019 (data unpublished). The Acquired Immune Deficiency Syndrome (AIDS) epidemic, since the first cases were reported in June 1981, has caused many changes in the disease pattern, including CNS infection [Citation4]. Cerebral toxoplasmosis has become one of the primary CNS problem among people living with human immunodeficiency virus (HIV)/-AIDS (PLWH) [Citation5–12], especially in some areas with a high burden of HIV infection and low access to highly active antiretroviral (HAART) therapy [Citation13]. It commonly manifests in PLWH with HIV viral load above 50 copies/ml and CD4+ cell counts <100/mm3, p < 0.05 [Citation14,Citation15]. The incidence declines in countries with better access and adherence to antiretroviral therapy, early HIV diagnosis, good care retention, and low antiretroviral drug resistance [Citation16]. The most common terminologies for brain T. gondii infection are ‘toxoplasmic encephalitis’, ‘toxoplasma encephalitis’, or ‘cerebral toxoplasmosis’ rather than ‘toxoplasma abscess’, which referred to pus formation that is usually delayed in the immunocompromised host [Citation16]. According to MeSH terms, cerebral toxoplasmosis refers to ‘neurotoxoplasmosis’, ‘toxoplasmosis central nervous system’, or ‘intracranial toxoplasmosis’. We reviewed original papers and systematic reviews published since 1 January 2018, for new developments in the pathophysiology, diagnosis, treatment, and prognosis of cerebral toxoplasmosis in HIV-infected patients.
Pathogenesis
Toxoplasma gondii is a protozoan parasite and foodborne pathogen, in which the direct contact with cat litter or consumption of contaminated water or food by sporulated oocysts may also serve as the route of infection, as well as consumption of undercooked meat harboring tissue cyst of the parasite ().
Figure 1. Summary of the pathogenesis of cerebral toxoplasmosis. (A) Infection in humans occurs via consuming food or water contaminated with oocyst excreted in cat feces or bradyzoites-containing cysts in raw or poorly cooked meat. (B) Maturation of oocyst in the open air makes it infectious and ready to penetrate the host’s epithelium of the intestine. (C) The tachyzoites are replicated inside the dendritic cells (DCs) and can transverse to the blood-brain barrier. Blood-cerebrospinal fluid barrier through a mechanism that enables the invasion of and migration through cerebral vascular endothelial cells (paracellularly and transcellular) or are carried into the CNS by infected peripheral innate immune cells, i.e. dendritic cells (A trojan mechanism) that dependent to the increase of CCR7r, GABA, GABA-Ar, Ca2+ channel. (D) In the CNS, tachyzoites replication occur inside the neuron and antigen recognition and internalization by microglia, neurons, and astrocytes occurs – and stimulates the release of proinflammatory cytokines and chemokines and other immune mediators that contribute to immune responses and parasite control. (E) T. gondii stimulates the production of GABA, EGFR, GRA-15 effector, P2X7, and ROS to suppress the host’s immune mechanism and facilitate rapid parasite transfer between cells in the brain parenchyma. (F) Reactivation of bradyzoites in cerebral cysts induced by decrease of CD4+ and CD8 + T cells, IFN- γ, MMP-8, or MMP10.
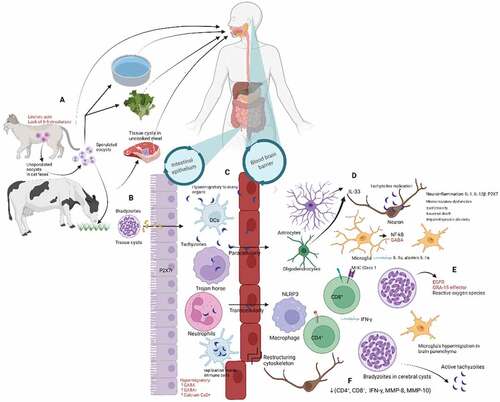
A non-sporulated oocyst excreted in cat feces transforms into a highly infectious sporulated form in fresh air [Citation17,Citation18]. The sexual reproductive phase of T. gondii occurs only in cats, its definitive host, facilitated by the intrinsic abundance of linoleic acid and the absence of the enzyme delta-6-desaturase. The intermediate host, including humans, mammals, and birds are infected after consuming water or food contaminated by infectious oocyst or after ingesting prey containing tissue cysts [Citation18]. The cyst wall is later digested in the host’s stomach and intestine, releasing bradyzoites, which penetrate further into the epithelium of the intestine. Inside the cysts, several hundred bradyzoites remain covered from the host immune system. Host immune responses and anti-toxoplasmosis can limit their growth and force the tachyzoite to transform into slowly replicating bradyzoites. Bradyzoites become active when the immune system becomes compromised, leading to clinical manifestation [Citation18].
In vivo and in vitro experiments suggest that T. gondii invades the host blood–brain barrier through three possible mechanisms (). First, it uses immune cells, such as macrophage, neutrophils, dendritic cells (DCs), and monocytes, to spread and persistently reside in neural and other brain cells through the Trojan horse mechanism [Citation14,Citation18,Citation19]. Second, the extracellular tachyzoites transmigrate paracellularly through tight junctions between endothelial cells [Citation20]. Third, T. gondii infects endothelial cells during transcellular dissemination and is released into the neural parenchyma. The role of lymphatic system in systemic dissemination in both the mesenteric and brain is still uncertain. The fact that T. gondii is difficult to detect in the cerebrospinal fluid (CSF) by polymerase chain reaction (PCR) test suggests that blood circulation is involved in its arrival at the brain parenchyma [Citation20]. Chronic infection of T. gondii causes neuroinflammation, and microcirculatory dysfunction leads to decreased angiogenesis and possibly takes part in the hemorrhagic process and invasion of parasites to the brain parenchyma [Citation21].
The balance between immune responses and parasite control in the brain is strictly regulated to avoid organ damage. Hyperinflammation leads to impaired synaptic plasticity, excitotoxicity, neuronal dysfunction, and death [Citation22,Citation23]. Microglia plays an essential role in defense mechanisms against T. gondii by producing nuclear factor kappa-light-chain-enhancer of activated B cells (NF-kB) and inflammatory cytokine, especially interleukin 1α (IL-1α) and alarmin IL-1α. The host immune system, including Toll-like receptor, microglia, CD4+ and CD8 + T cells, produces interferon-γ (IFN-γ) to inhibit T. gondii proliferation and control chronic infection [Citation16,Citation18,Citation24]. CD8+ cells – mediated by major histocompatibility complex (MHC) class I presentation – can penetrate the T. gondii cysts perforin-mediated manner to induce morphological deterioration and destruction of the cysts [Citation25,Citation26]. Oligodendrocytes and astrocytes might also have a role in releasing IL-33 into the cerebrospinal fluid to control infection [Citation27]. The P2X7 receptor induces parasitic control by increasing ROS production NLRP3 inflammasome activation [Citation28,Citation29], and IL1-β, IL-12, IFN-γ, TNF-α secretion in human [Citation30] or mice [Citation31]. Extracellular vesicles (EV) in serum and CSF of cerebral toxoplasmosis patients were significantly higher than the standard control. T. gondii promotes the immune system to produce host EV, including micro-vesicles and exosomes that induce modifications in uninfected cells or serve as antigen presenters for the immune system [Citation32]
On the other hand, T. gondii produces virulence factors to suppress this immune mechanism, including the effector secreted by dense granule protein (GRA)15-dependent virulence mechanism, which indirectly inhibits indoleamine 2,3-dioxygenase 1(IDO1)-dependent anti-T. gondii in the brain cells [Citation33,Citation34]. Microglia are considered to participate in disseminating T. gondii in the brain parenchyma by modulating gamma-aminobutyric acid (GABA) levels. Infected microglia undergo morphological changes and increase GABA levels, facilitating microglia’s hypermigration, leading to rapid parasite transfer between cells in the brain parenchyma [Citation35]. T. gondii likely activates epidermal growth factor receptor (EGFR) during the invasion and allows it to survive from autophagy-dependent lysosomal degradation of the parasite [Citation20].
T. gondii possesses dihydrofolate reductase (DHFR) and dihydropteroate synthetase (DHPS) enzymes necessary for folate synthesis. The parasites also have been suggested to lack alternative mechanisms for folate acquisition. Folate is central for DNA synthesis and repair and is a crucial factor in the cell cycle (). The brain requires a supply of dietary folate for neurogenesis and the production of neurotransmitters. Therefore, decreased availability of folate is associated with neurodevelopmental disorders. People without enough folate in their diet or who cannot metabolize folate efficiently may have a neuron that is generally and permanently deprived of this essential nutrient [Citation16].
Figure 2. Summary of folic acid pathway. Folate contained in natural food or folic acid as a synthetic form in supplements is a critical substance in the metabolism of nucleic acid precursors and several amino acids. P-aminobenzoic acid (PABA) is a cofactor of enzymes dihydropteroate synthase needed to synthesize folic acid. Sulfonamides act as a competitor of PABA, which prevents the synthesis of dihydrofolic acid. In combination with sulfamethoxazole, Trimethoprim is a structural analog to dihydrofolic acid, which competitively inhibits the conversion of the dihydrofolic acid to tetrahydrofolic acid. Eventually, the DNA synthesis will be interrupted.
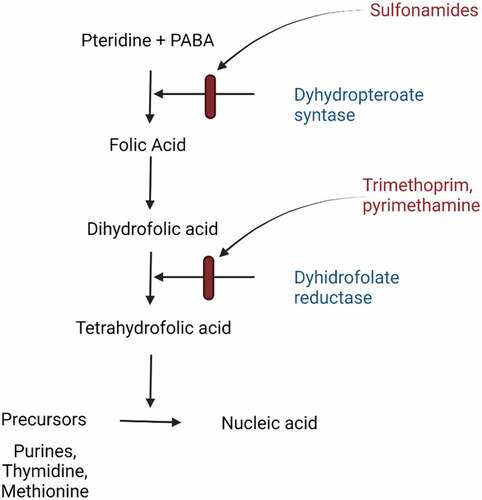
In immunocompetent individuals, toxoplasmosis is an asymptomatic infection or manifests only as mild clinical symptoms such as fever, malaise, or lymphadenitis. However, when immunocompromised patients, such as those with AIDS, are infected, bradyzoite can reactivate and transform into cytotoxic tachyzoites and cause an intracerebral mass lesion. Lau et al. reported the median time from HIV diagnosis to cerebral toxoplasmosis diagnosis was 22.1 months (0–338.7) in 38 HIV-infected patients in Dallas, USA [Citation36]. There is doubt about the neurotropism of T. gondii since the concentration in the brain is low compared to other organs in mice. Slow shedding of the parasite from the CNS, allowing chronic reactivation infection, could be considered neurotropism. Brain tissue is one of the significant structures in which abnormalities caused by the multiplying parasites have been noted. The parasite infection leads to necrotic foci and profound inflammation that can block the Sylvian aqueduct and cause hydrocephalus in the lateral ventricles [Citation2,Citation3].
Diagnosis
Diagnosis of cerebral toxoplasmosis comprises four categories: histology confirmed, laboratory-confirmed, probable or presumptive, and possible cerebral toxoplasmosis. The first two categories are considered definite diagnoses and used if patients have the compatible clinical syndrome, focal brain lesion in the neuroimaging, and either evidence of T. gondii in brain biopsy or T. gondii DNA in the CSF by nucleic acid amplification assays [Citation37]. Probable or presumptive diagnosis is more common and usually applied when patients have compatible clinical signs, one or more mass lesions in the neuroimaging, and unequivocal radiological response to 10–14 days of empiric anti-toxoplasma therapy or presence of serum T. gondii immunoglobulin G (IgG) antibodies, and no other alternative diagnosis. The possible cerebral toxoplasmosis applied if patient eventually died or left the hospital without radiological confirmation [Citation16].
Clinical manifestations of the disease depend mainly on the topography and size of the lesions. The most common signs and symptoms are headaches, seizures [Citation38], focal neurological deficit, fever, mental confusion, psychomotor or behavioral changes, cranial nerve palsy, ataxia, and visual abnormalities. Patients may also present with intracranial hypertension syndrome and involuntary movements. Progression of neurological abnormalities results in stupor and coma, and death might occur in untreated patients. Nuchal rigidity is rare, as it mainly causes encephalitis without meningeal involvement [Citation39].
Magnetic resonance imaging (MRI) is superior to CT scan in cerebral toxoplasmosis diagnosis. However, despite its lower sensitivity, CT scan is more readily accessible in the most low-resourced settings. Typical neuroradiology findings are multiple ring-enhancing lesions in the basal ganglia, frontal lobe, and parietal lobe, with perifocal edema. The occipital lobe, temporal lobe, and brain stem/cerebellum are less frequently involved. Nodular-enhancing lesions with perilesional edema and non-enhancing lesions with expansive effects are observable. Isolated cerebral edema without focal lesion is possible. The most specific neuroradiology signs for cerebral toxoplasmosis are ‘eccentric target sign,’ a ring-shaped alternating zone of peripheral enhancement with innermost enhancing core, an intermediate hypointense zone, and an outer peripheral hyperintense enhancing rim (on postcontrast CT or T1-weighted MRI) with a slight eccentric nodule along the wall (30%), and ‘concentric target sign’ on T2-weighted MR imaging, described as concentric alternating zones of hypo- and hyperintensities [Citation18,Citation19]. Typically, one or both signs are present. Differential diagnosis of ring-enhancing brain lesions in patients with advanced HIV includes lymphoma which is usually located at subependymal and subarachnoid, progressive multifocal leukoencephalopathy, and other brain infections (tuberculoma, bacterial abscess, Nocardia infection, or cryptococcus). Magnetic resonance spectroscopy (MRS) provides information about metabolic signal with 70–100% sensitivities and 27–83% specificities. Toxoplasma lesions show lipid and lactate elevation with resonance peaks at 0.9–1.4 ppm ().
Figure 3. Magnetic resonance spectroscopy of patient with cerebral toxoplasmosis. (A) Lesion at left midbrain and pons. (B) Multiple cortical and subcortical ring enhanced lesions at frontoparietalis dextra, bilateral basal ganglia, right amygdala, and left cerebellum causing midline shift 0.45 cm to the left. There is an increment of lactate peak 54.5 intralesional (normal value: 6.01) and decrease of NAA, choline, and creatinine peak 1.8, 7.14, and 1.06 (normal value: 18.1, 11, and 13.6).
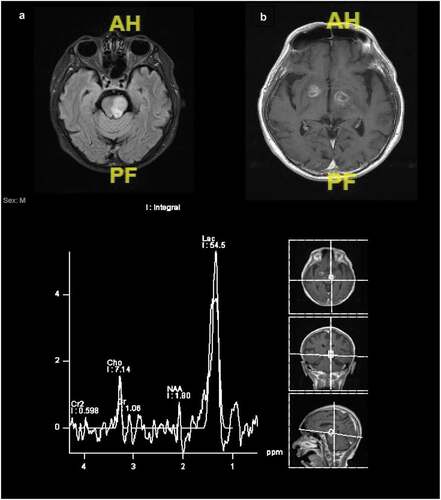
Lumbar puncture should be performed only if safe and feasible, and the basic features of the CSF are usually insignificant, with only subtle abnormalities such as increased cell count and normal or vaguely increased protein levels. Positivity of CSF T. gondii PCR is related to the intracellular location of T. gondii, the number of lesions, and their distance from the CSF paths; therefore, a negative CSF T. gondii PCR does not exclude the diagnosis of cerebral toxoplasmosis. Specificity of CSF PCR is 96–100%, but sensitivity is below 50% [Citation40]. Antiparasitic treatment reduces sensitivity of the PCR test, but primary prophylaxis does not. Even though blood DNA concentration of T. gondii is very low compared to its concentration in the CSF and, therefore, sensitivity of the molecular blood test is lower, it is a feasible alternative for patients contraindicated for lumbar puncture [Citation18].
Several other diagnostic methods are tested and developed, e.g. the use of blood clot for quantitative PCR detection of T. gondii on the B1 and REP529 genes [Citation41], nested PCR assay using B1 gene in serum and peripheral blood mononuclear cell [Citation42], real-time isothermal amplification [Citation43], metagenomic analysis through next-generation sequencing [Citation44]. Blood microRNA (miR), especially miR-21-5p and miR-146a-5p, were modulated in cerebral toxoplasmosis and might offer a new marker for diagnostic purposes [Citation45]. Increase in sensitivity was also observed when a high-resolution melting analysis technique employing RE gene was performed in human blood samples, equivalent to the TaqMan real-time PCR [Citation45].
Anti T. gondii immunoglobulin G antibodies titters values above 7IU/ml were considered seropositive for T. gondii exposure and indicate past toxoplasma exposure. Seroprevalence of T. gondii was higher in CD4 counts <200 cells/mm3 compared to >200 cells/mm3 of HIV-infected patients [Citation46]. Single neuroradiology lesions and negative anti-toxoplasma IgG antibodies do not negate diagnosis of cerebral toxoplasmosis, especially in the endemic area. Seronegativity may result from several conditions, including primary infection, reactivation of latent disease in individuals who cannot produce detectable antibodies, or testing with insensitive assays [Citation37]. A comparison between CSF/blood anti-toxoplasma and blood/CSF albumin was proposed to improve the accuracy of cerebral toxoplasmosis diagnosis [Citation40].
Diagnostic testing of aptamers-based assay are being developed, including for toxoplasmosis. An Enzyme-linked aptamer assay (ELAA) was developed using two aptamers to detect total antigen from RH strain and recombinant protein of Rhoptry protein 18 (ROP18). One study showed significant association between the ELAA test for human serum samples and severe congenital toxoplasmosis [Citation47]; while another study showed that the aptamer against surface antigen 1 (SAG1) protein of T. gondii used in a new direct enzyme-linked aptamer assay (DELAA) had higher specificity and sensitivity than the ELISA for T. gondii’s circulating agent when used on human sera that had been verified for Toxoplasma DNAs by PCR method.
Treatment
Treatment response is a significant part of cerebral toxoplasmosis diagnosis. Treatment should be given once the diagnosis of probable/presumptive cerebral toxoplasmosis has been made. Recent treatment for cerebral toxoplasmosis is available only for tachyzoites eradication, in both acute and reactivated infections. [Citation19] No regimen has yet been effective against the latent stage of T. gondii.
Sulfonamide and dapsone, two effective drugs for toxoplasmosis, act on parasite DHPS – an enzyme produced by both T. gondii and humans for blocking folic acid synthesis – is the main target of anti-toxoplasma drugs (). Pyrimethamine (PYR) and trimethoprim (TMP) are important anti-toxoplasmosis that inhibits DHFR that plays a part in the synthesis of tetrahydrofolate [Citation48]. Current regimens for toxoplasmosis tend to have side effects and low compliance for several reasons. First, DHFR is the part of the folate pathway involved in DNA synthesis in humans (hDHFRw) and parasites (TgDHFR), and results in myelotoxicity. Second, as a single antibiotic is not powerful enough for treating toxoplasmosis, a combination is needed. PYR/TMP is often combined with clindamycin, atovaquone, clarithromycin, or azithromycin [Citation48].
Several drug combinations were tested in clinical trials and showed similar efficacy. PYR/sulfadiazine (SDZ) was studied at various doses (PYR from 50 to 100 mg/day) and compared with PYR/clindamycin (CLD) or trimethoprim (TMP)/sulfamethoxazole (SMX) [Citation49]. PYR/SDZ emerged as the most effective treatment with fewer relapses during maintenance therapy than PYR/CLD, but it caused more side effects than PYR/CLD or TMP/SMX [Citation37].
For induction phase, the Infectious Disease Society of America (IDSA) lauds a 200 mg loading dose of PYR on day 1, then 75 mg/day if the patient weighs >60 kg or 50 mg/day if the patient weighs <60 kg. This treatment is also combined with SDZ (1500 mg twice daily if the patient weighs ≥60 kg, or 1000 mg/day if the patient weighs <60 kg) and folinic acid (10–25 mg/day) (Level AI recommendation) [Citation39]. The alternative regimens are PYR/CLD (600–900 mg four times a day) + folinic acid (grade AI) or TMP (5 mg/kg twice a day) combined with pyrimethamine (and folinic acid) or with sulfadiazine. In comparison, azithromycin (900–1200 mg) can be administered along with pyrimethamine (and folinic acid), grade BII. The use of clindamycin as a single drug to prevent the side effect of PYR lacks a solid recommendation and since it leads to a high relapse rate. Induction therapy should be taken for at least 6 weeks (until clinical and radiographic improvement) followed by maintenance therapy until CD4+ cell count stays above 200 cells/mm3 and HIV viral load is undetectable for up to 6 months () [Citation37,Citation48].
Table 1. Treatment of cerebral toxoplasmosis.
Pyrimethamine is not always available in many countries, and its bioavailability decreases in a malnourished patient, resulting in broader use of TMP/SMX as the first line [Citation50]. PYR/SDZ and TMP/SMX present good bioavailability (70% and 90%, respectively) and have a long half-life (about 4 days), underlying the need for a loading dose. A meta-analysis did not show a superior antiparasitic association among PYR/SDZ, PYR/CLD, or TMP/SMX, reinforcing the value of choosing TMP/SMX when PYR/SDZ is not available [Citation51]. However, long-term use of TMP/SMX for prophylaxis or curative treatment could raise drug resistance. In contrast, Pellegrino reported the efficacy of TMP/SMX in treating relapses in both patients with and without prior use, pointing to the small possibility of resistance to this drug [Citation50]. A randomized controlled trial is ongoing to evaluate the efficacy and safety of TMP/SMX plus azithromycin compared to sulfonamides plus clindamycin for cerebral toxoplasmosis ChiCTR1900021195 [Citation52].
The main side effects of toxoplasma drugs are myelotoxicity, including leukopenia and thrombocytopenia. PYR/SDZ side effects occur in more than half of patients and result in treatment cessation in 45% of patients. Including leucovorin/folinic acid in long-term antifolate treatment regimens for T. gondii is now standard to avoid hematologic toxicity. The addition of leucovorin is assumed to help maintain host folate pools but not induce an antagonistic effect on the antiparasitic activity of the antifolate compounds since the parasites are supposedly incapable of salvaging the reduced folate. Leucovorin or folinic acid is not similar to folic acid.
No regimen has yet been effective against the latent stage of T. gondii. Several antibiotics have been used in clinical practice for eradicating T. gondii, i.e. sulfonamides since the 1940s, pyrimethamine plus sulfonamides since 1950, and trimethoprim/sulfamethoxazole since 1970 [Citation2]. Several new candidates including para-hydroxynaphthoquinones, endochin-like quinolones (ELQ-271, ELQ-316), artemisone and artemiside, guanabenz, and miltesofine, which are effective against both tachyzoites and bradyzoites in in-vivo studies, are under investigation. However, clinical trials in patients with cerebral toxoplasmosis are warranted [Citation48].
Corticosteroids may be necessary to reduce brain edema but can sometimes be detrimental by increasing immunosuppression. Centers for Disease Control and Prevention and IDSA recommended its use only for cerebral lesions with mass effect [Citation53]. In this case, 7 days of dexamethasone 4 mg every 6 h may be administered. Several clinical trials have investigated corticosteroid as an adjunctive treatment with PYR/SDZ and it is considered the treatment of choice in ocular but not cerebral toxoplasmosis [Citation48]. A double-blind, randomized control trial is underway in Bandung to evaluate the efficacy and safety of dexamethasone in cerebral toxoplasmosis (NCT04341155). Immune reconstitution inflammatory syndrome (IRIS) is not common in cerebral toxoplasmosis patients. Although the treatment of IRIS in cerebral toxoplasmosis has not been sufficiently studied, steroids remain the therapeutic aid since they inhibit the cell destruction due to immune recovery.
HAART in cerebral toxoplasmosis usually starts within 2 weeks after the initiation of cerebral toxoplasmosis treatment. Primary prophylaxis therapy is given to PLWH with CD4+ cell level <100 cells/mm3 or <200 cells/mm3. In a phase IV, multicenter, prospective, randomized open-label clinical trial, Schafer et al. found similar safety between immediate (within 7 days) and deferred (after completing OI-therapy) for starting antiretroviral therapy in cerebral toxoplasmosis patients (3–6 weeks) [Citation54]. A randomized controlled trial is ongoing to evaluate the optimal timing for HAART in PLWH with cerebral toxoplasmosis ChiCTR1900021195 [Citation55]. Wang et al. reported that most HAART compounds had no significant interaction with sulfadiazine and pyrimethamine [Citation56].
Immunotherapy affecting cellular effectors, mainly IFNγ-producing T lymphocytes, inhibiting specific interleukins such as IL-10, or transferring the immune CD8+ have been proposed to control the multiplication and spread of T. gondii and maintain latency. An in vivo study proposed rosuvastatin [Citation57] and resveratrol [Citation58] as neuroprotective agents, but clinical trials are absent.
Several animal models have been extensively investigated in trials to find a safe and effective vaccine. Microneme antigens (MICs), dense granule antigens (GRAs), surface antigens (SAGs), and ROP, are constantly investigated as protein sequences that may produce ideal antigens [Citation49,Citation59,Citation60]. The ideal vaccine would be directed against antigens implicated in attachment or penetration in the host cells at the different T. gondii stages [Citation61]. It is to be noted that any predictive protective effect estimated in animal models cannot be extrapolated to humans.
Prognosis
Only about 15% of patients with anti-toxoplasma treatment showed no clinical improvement within the first week, and only 10% of patients were not improved after 2 weeks. Therefore, the absence of a reaction within the first 1 to 2 weeks should raise suspicion of another underlying etiologies[Citation62]. Notably, radiologic improvement lags behind clinical improvement and does not necessarily signify unsuccessful therapy. Drug resistance is not an issue in treating toxoplasmosis. Failure to respond to appropriate therapy is related to delayed diagnosis and host factors such as poor immune response. Approximately 11–19% of the patients who had recovered after taking pyrimethamine-based and 16.4% after TMP-SMX as secondary prophylaxis will later experience a recurrency [Citation63,Citation64]. The most critical risk factor for relapse is inadequate adherence to secondary prophylaxis treatment.
Cerebral toxoplasma generally leads to death or disability in HIV-infected patients [Citation5,Citation10,Citation65]. Mortality during hospitalization can be as high as 11–30%. Li et al. developed a scoring system consisting of fever, dizziness, CD4+ cell count <25 cells/µl, memory deficits, patchy brain lesions, unconsciousness, and time from onset of symptoms to presentation ≥15 days for prognosis in patients with cerebral toxoplasmosis, of which the value of 9 or more was significantly associated with 6-week mortality with sensitivity and specificity of 100% and 86.9%, respectively; the area under the receiver operating characteristic (ROC) curve was 0.976 (p < 0.001) [Citation66].
Conclusion
Toxoplasmosis remains a global burden and more troublesome in immunosuppressive conditions such as HIV-infected patients. Definite diagnosis of cerebral toxoplasmosis from the brain tissue or cerebrospinal fluid is frequently impossible despite fast clinical improvement after a recently available regimen. Trial-supported vaccination for adults and the discovery of more potent drugs that do not interfere with hematopoiesis are needed.
Authorship
All named authors meet the International Committee of Medical Journal Editors (ICMJE) criteria for authorship of this article, accept responsibility for the integrity of the work as a whole, and have given their consent to the publication of this version.
Ethical approval statement
This article is based on the most recent studies and does not include studies with human participants or animals conducted by any of the authors.
Contributions
All the authors worked collectively to develop the final version of the manuscript. SD wrote the first draft. AR and SE provided contributions and suggestions.
Acknowledgments
created in Biorender.com. The authors would like to thank Winston Sanjaya who helped with the data collection in “Dexamethasone for Cerebral Toxoplasmosis (DeTox)” trial.
Disclosure statement
No potential conflict of interest was reported by the author(s).
Additional information
Funding
References
- Safarpour H, Cevik M, Zarean M, et al. Global status of Toxoplasma gondii infection and associated risk factors in people living with HIV. AIDS. 2020;34(3):469–474.
- CDC. Global health, division of parasitic diseases and malaria. Accessed 1 April 2021. https://www.cdc.gov/parasites/toxoplasmosis/epi.html
- Wang ZD, Wang SC, Liu HH, et al. Prevalence and burden of Toxoplasma gondii infection in HIV-infected people: a systematic review and meta-analysis. Lancet HIV. 2017;4(4):e177–e188.
- WHO. AIDS epidemic update. USAIDS; 2000.
- Lakoh S, Jiba DF, Kanu JE, et al. Causes of hospitalization and predictors of HIV-associated mortality at the main referral hospital in Sierra Leone: a prospective study. BMC Public Health. 2019;19(1):1320.
- Nacher M, Adenis A, Guarmit B, et al. What is AIDS in the Amazon and the Guianas in the 90-90-90 era? PLoS One. 2020;15(7):e0236368.
- Telles JP, Fernandes R, Barros TD, et al. Neurological manifestations in people living with HIV/AIDS in the late cART era: a prospective observational study at a tertiary healthcare center in São Paulo, Brazil. HIV Res Clin Pract. 2021;22(4): 87–95.
- Jianu C, Bolboacă SD, Topan AV, et al. A view of human immunodeficiency virus infections in the North-West Region of Romania. Medicina (B Aires). 2019;55(12):765.
- Imran D, Estiasari R, Maharani K, et al. Presentation, etiology, and outcome of brain infections in an Indonesian hospital: a cohort study. Neurol-Clin Pract. 2018;8(5):379–388.
- Raberahona M, Razafinambinintsoa T, Andriananja V, et al. Hospitalization of HIV positive patients in a referral tertiary care hospital in Antananarivo Madagascar, 2010–2016: trends, causes and outcome. PLoS One. 2018;13(8):e0203437.
- El Fane M, Sodqi M, Lamdini H, et al. Central neurological diagnosis in patients infected with HIV in the Infectious Diseases Unit of University Hospital of Casablanca, Morocco. Bull Soc Pathol Exot. 2018;111(1):24–30.
- Gams Massi D, Mintyene Mintyene MRR, Magnerou AM, et al. Spectrum of central nervous system infections in a tertiary health care centre in Cameroon. Egypt J Neurol Psychiatr Neurosurg. 2022;58(1):18.
- Pacheco PRG, Zara ALSA, Souza SE, et al. Late onset of antiretroviral therapy in adults living with HIV in an urban area in Brazil: prevalence and risk factors. J Trop Med. 2019;2019:5165313.
- Seo HH, Han HW, Lee SE, et al. Modelling. Emerg Microbes Infect. 2020;9(1):1943–1954.
- Azovtseva OV, Viktorova EA, Bakulina EG, et al. Cerebral toxoplasmosis in HIV-infected patients over 2015-2018 (a case study of Russia). Epidemiol Infect. 2020;148:e142.
- Vidal JE. HIV-related cerebral toxoplasmosis revisited: current concepts and controversies of an old disease. J Int Assoc Provid AIDS Care. 2019;18:2325958219867315.
- Attias M, Teixeira DE, Benchimol M, et al. The life-cycle of Toxoplasma gondii reviewed using animations. Parasit Vectors. 2020;13(1):588.
- Elsheikha HM, Marra CM, Zhu XQ. Epidemiology, pathophysiology, diagnosis, and management of cerebral toxoplasmosis. Clin Microbiol Rev. 2021;34(1):e00115–19.
- Graham AK, Fong C, Naqvi A, et al. Toxoplasmosis of the central nervous system: manifestations vary with immune responses. J Neurol Sci. 2021;420:117223.
- Corcino YL, Portillo JC, Subauste CS. Epidermal growth factor receptor promotes cerebral and retinal invasion by Toxoplasma gondii. Sci Rep. 2019;9(1):669.
- Schlüter D, Barragan A. Advances and challenges in understanding cerebral toxoplasmosis. Front Immunol. 2019;10:242.
- El Saftawy EA, Shash RY, Aboulhoda BE, et al. Insights into immunopathology and triggering of apoptosis in chronic cerebral toxoplasmosis using murine models. Trop Biomed. 2021;38(2):53–62.
- Moreira-Souza ACA, Rangel TP, Silva SRBD, et al. Disruption of purinergic receptor P2X7 signaling increases susceptibility to cerebral toxoplasmosis. Am J Pathol. 2019;189(4):730–738.
- Ihara F, Tanaka S, Fereig RM, et al. Involvement of Toll-like receptor 2 in the cerebral immune response and behavioral changes caused by latent Toxoplasma infection in mice. PLoS One. 2019;14(8):e0220560.
- Salvioni A, Belloy M, Lebourg A, et al. Robust control of a brain-persisting parasite through MHC I presentation by infected neurons. Cell Rep. 2019;27(11):3254–3268.e8.
- Suzuki Y. The immune system utilizes two distinct effector mechanisms of T cells depending on two different life cycle stages of a single pathogen, Toxoplasma gondii, to control its cerebral infection. Parasitol Int. 2020;76:102030.
- Still KM, Batista SJ, O’Brien CA, et al. Astrocytes promote a protective immune response to brain Toxoplasma gondii infection via IL-33-ST2 signaling. PLoS Pathog. 2020;16(10):e1009027.
- Moreira-Souza ACA, Almeida-da-Silva CLC, Rangel TP, et al. The P2X7 receptor mediates Toxoplasma gondii control in macrophages through canonical NLRP3 inflammasome activation and reactive oxygen species production. Front Immunol. 2017;81257. DOI:10.3389/fimmu.2017.01257
- Alves VS, Leite-Aguiar R, Silva JPD, et al. Purinergic signaling in infectious diseases of the central nervous system. Brain Behav Immun. 2020;89:480–490.
- Huang SW, Walker C, Pennock J, et al. P2X7 receptor-dependent tuning of gut epithelial responses to infection. Immunol Cell Biol. 2017;95(2):178–188.
- Corrêa G, Marques da Silva C, de Abreu Moreira-Souza AC, et al. Activation of the P2X(7) receptor triggers the elimination of Toxoplasma gondii tachyzoites from infected macrophages. Microbes Infect. 2010;12(6):497–504.
- da Cruz Ab, Maia MM, Pereira IDS, et al. Human extracellular vesicles and correlation with two clinical forms of toxoplasmosis. PLoS One. 2020;15(3):e0229602.
- Bando H, Lee Y, Sakaguchi N, et al. Effector GRA15-dependent suppression of IFN-γ-induced antiparasitic response in human neurons. Front Cell Infect Microbiol. 2019;9:140.
- Bando H, Sakaguchi N, Lee Y, et al. Effector TgIST targets host IDO1 to antagonize the IFN-γ-induced anti-parasitic response in human cells. Front Immunol. 2018;9:2073.
- Bhandage AK, Kanatani S, Barragan A. Induced hypermigration of primary cortical microglia implicates GABAergic signaling. Front Cell Infect Microbiol. 2019;9:73.
- Lau A, Jain MK, Chow JY, et al. Toxoplasmosis encephalitis: a cross-sectional analysis at a U.S. safety-net hospital in the late cART era. J Int Assoc Provid AIDS Care. 2021;20:23259582211043863.
- Panel on Opportunistic Infections in Adults and Adolescents with HIV. Guidelines for the prevention and treatment of opportunistic infections in adults and adolescents with HIV: recommendations from the Centers for Disease Control and Prevention, the National Institutes of Health, and the HIV Medicine Association of the Infectious Diseases Society of America. 2020.
- Amare A. Seizure in HIV-infected patients: clinical presentation, cause and treatment outcome in Ethiopia-a retrospective study. BMC Infect Dis. 2021;21(1):790.
- Kaplan, Jonathan , Benson, Constan , Holmes, King CDC. Guidelines for prevention and treatment of opportunistic infections in HIV-infected adults and adolescents. Vol. 58. 2009. Accessed 2021 4 2021. Recommendations for CDC, the National Institutes of Health, and the HIV Medicine Association of the Infectious Diseases Society of America. http://aidsinfo.nih.gov/contentfiles/lvguidelines/adult_oi.pdf.
- Bondarenko AV, Katsapov DV, Gavrylov AV, et al. Immunodiagnostics of cerebral toxoplasmosis depending on permeability of blood-brain barrier. Wiad Lek. 2020;73(2):285–288.
- Gutierrez-Loli R, Ferradas C, Diestra A, et al. Development of a novel protocol based on blood clot to improve the sensitivity of qPCR detection of. Am J Trop Med Hyg. 2019;100(1):83–89.
- Bokharaei-Salim F, Esteghamati A, Khanaliha K, et al. Evaluation of a PCR assay for diagnosis of toxoplasmosis in serum and peripheral blood mononuclear cell among HIV/AIDS patients. J Parasit Dis. 2020;44(1):159–165.
- Wang ZH, Zhang W, Zhang XZ, et al. Development of a real-time recombinase-aided amplification (RT-RAA) molecular diagnosis assay for sensitive and rapid detection of Toxoplasma gondii. Vet Parasitol. 2021;298:109489.
- Xu M, Gao J, Li S, et al. Metagenomic analysis and identification of emerging pathogens in blood from healthy donors. Sci Rep. 2020;10(1):15809.
- de Faria Junior GM, Murata FHA, Lorenzi HA, et al. The role of microRNAs in the infection by. Front Cell Infect Microbiol. 2021;11:670548.
- Yenilmez E, Çetinkaya RA. Difference in. Turkiye Parazitol Derg. 2019;43(Suppl 1):1–7.
- Vargas-Montes M, Cardona N, Moncada DM, et al. Enzyme-Linked Aptamer Assay (ELAA) for detection of. Front Cell Infect Microbiol. 2019;9:386.
- Konstantinovic N, Guegan H, Stäjner T, et al. Treatment of toxoplasmosis: current options and future perspectives. Food Waterborne Parasitol. 2019;15:e00036.
- Foroutan M, Ghaffarifar F, Sharifi Z, et al. Bioinformatics analysis of ROP8 protein to improve vaccine design against Toxoplasma gondii. Infect Genet Evol. 2018;62:193–204.
- Pellegrino D, Gryschek R, de Oliveira ACP, et al. Efficacy and safety of trimethoprim-sulfamethoxazole in HIV-infected patients with cerebral toxoplasmosis in Brazil: a single-arm open-label clinical trial. Int J STD AIDS. 2019;30(12):1156–1162.
- Hernandez AV, Thota P, Pellegrino D, et al. A systematic review and meta-analysis of the relative efficacy and safety of treatment regimens for HIV-associated cerebral toxoplasmosis: is trimethoprim-sulfamethoxazole a real option? HIV Med. 2017;18(2):115–124.
- Li Y, Zeng YM, Lu YQ, et al. A study for precision diagnosing and treatment strategies in difficult-to-treat AIDS cases and HIV-infected patients with highly fatal or highly disabling opportunistic infections. Medicine (Baltimore). 2020;99(20):e20146.
- Azoulay É, de Castro N, Barbier F. Critically ill patients with HIV: 40 years later. Chest. 2020;157(2):293–309.
- Schäfer G, Hoffmann C, Arasteh K, et al. Immediate versus deferred antiretroviral therapy in HIV-infected patients presenting with acute AIDS-defining events (toxoplasmosis, Pneumocystis jirovecii-pneumonia): a prospective, randomized, open-label multicenter study (IDEAL-study). AIDS Res Ther. 2019;16(1):34.
- Zeng YM, Li Y, He XQ, et al. A study for precision diagnosing and treatment strategies in difficult-to-treat AIDS cases and HIV-infected patients with highly fatal or highly disabling opportunistic infections: study protocol for antiretroviral therapy timing in AIDS patients with toxoplasma encephalitis. Medicine (Baltimore). 2020;99(29):e21141.
- Wang JL, Elsheikha HM, Li TT, et al. Efficacy of antiretroviral compounds against Toxoplasma gondii in vitro. Int J Antimicrob Agents. 2019;54(6):814–819.
- Nishi L, Santana PL, Evangelista FF, et al. Rosuvastatin reduced brain parasite burden in a chronic toxoplasmosis. Parasitology. 2020;147(3):303–309.
- Bottari NB, Reichert KP, Fracasso M, et al. Neuroprotective role of resveratrol mediated by purinergic signalling in cerebral cortex of mice infected by Toxoplasma gondii. Parasitol Res. 2020;119(9):2897–2905.
- Foroutan M, Ghaffarifar F, Sharifi Z, et al. Rhoptry antigens as. Clin Exp Vaccine Res. 2019;8(1):4–26.
- Warner RC, Chapman RC, Davis BN, et al. Review of DNA vaccine approaches against the parasite toxoplasma gondii. J Parasitol. 2021;107(6):882–903.
- Mévélec MN, Lakhrif Z, Dimier-Poisson I. Key limitations and new insights into the. Front Cell Infect Microbiol. 2020;10:607198.
- Dunay IR, Gajurel K, Dhakal R, et al. Treatment of toxoplasmosis: historical perspective, animal models, and current clinical practice. Clin Microbiol Rev. 2018;31(4):e00057–17.
- Connolly MP, Haitsma G, Hernández AV, et al. Systematic review and meta-analysis of secondary prophylaxis for prevention of HIV-related toxoplasmic encephalitis relapse using trimethoprim-sulfamethoxazole. Pathog Glob Health. 2017;111(6):327–331.
- Connolly MP, Goodwin E, Schey C, et al. Toxoplasmic encephalitis relapse rates with pyrimethamine-based therapy: systematic review and meta-analysis. Pathog Glob Health. 2017;111(1):31–44.
- Letang E, Rakislova N, Martinez MJ, et al. Minimally invasive tissue sampling: a tool to guide efforts to reduce AIDS-related mortality in resource-limited settings. Clin Infect Dis. 2021;73(Suppl_5):S343–S350.
- Li Y, Zeng YM, Liu M, et al. Development of a risk scoring system for prognostication in HIV-related toxoplasma encephalitis. BMC Infect Dis. 2020;20(1):923.