Abstract
UK law permits parents to use mitochondrial replacement (MR) to have genetically-related children without serious mitochondrial disease. However, long-term follow-up is required for each case. Whether this follow-up should be left to physicians, parents, or offspring has not been established. Due to the experimental status of MR, physicians must inform parents of the risks and the importance of follow-up tailored to a specific mitochondrial disease. Given that the use of MR is a responsible exercise of reproductive freedom, parents should ensure that the follow-up is performed properly and in the best interests of their offspring. On becoming legally competent, the resulting children should be entitled to refuse follow-up provided that the prevention of mitochondrial disease with no adverse effects has been evident till then. This offspring-centred long-term follow-up approach might also be applied to the use of MR for infertility treatment, even though the primary endpoint is healthy live births.
Introduction
Human cells contain both nuclear and mitochondrial genomes, respectively located in the nucleus (nDNA) and mitochondria (mtDNA). Approximately 100,000 mitochondria exist in the cytoplasm of mature oocytes, resulting in as many as 200,000–300,000 copies of mtDNA per oocyte (Ishii Citation2016). The organelle’s most important function is probably the production of adenosine triphosphate (ATP) through the respiratory chain, in which protons and electrons are tightly transported, while regulating the leakage of free radicals, via five enzymatic complexes that are coordinately encoded by the dual genomes. Human mtDNA is, in general, maternally transmitted to offspring. Mutations at 13 mtDNA genes as well as at least 228 nDNA genes have been linked to mitochondrial diseases (Koopman et al. Citation2012, OMIM_data_base).
Since 1996, clinics have performed several different reproductive techniques based on the transfer of different amounts of cytoplasm derived from donor oocytes to alter the composition of mitochondria (containing mtDNA) in resultant oocytes or zygotes (Ishii Citation2018). Due to the experimental status of these reproductive techniques, China, the USA and other countries have prohibited such mitochondrial manipulations, including cytoplasmic transfer (CT), maternal spindle transfer (MST) and pronuclear transfer (PNT) after controversial consequences were reported (Ishii and Hibino Citation2018). In 2015, the UK became the first country to legalize MST and PNT (collectively termed mitochondrial replacement [MR] in this article) for the sole purpose of safeguarding genetically-related offspring from serious mitochondrial disease (UK_Department_of_Health, Citation2015). Currently, the UK’s first case of MR is being awaited at Newcastle University that was granted permission to perform MR in 2017 (Newcastle_University Citation2017, Sample Citation2018). This UK legalization is encouraging similar legislative amendments in other countries, as expected (Ishii Citation2014). At present, Singapore and Australia are considering the legalization of MR for the prevention of mitochondrial disease in offspring (Winkler Citation2018). Meanwhile, MR has already been performed for infertility treatment in Ukraine, and for disease prevention in Mexico, without being subject to appropriate regulations, (Ishii Citation2017, Palacios-Gonzalez and Medina-Arellano Citation2017, Ishii and Hibino Citation2018).
However, research reports that were published after the legalization of MR in the UK suggest that the reproductive use of experimental MR can fail to prevent the onset of mitochondrial disease in offspring (Hyslop et al. Citation2016, Kang et al. Citation2016, Yamada et al. Citation2016). Furthermore, MR can unexpectedly adversely affect the first and subsequent generations if the resultant female individuals reproduce (Ishii Citation2018). Accordingly, the need for long-term and even intergenerational follow-up of resultant offspring born via MR has been underscored as one of the major issues surrounding the clinical implementation of MR (Nuffield_Council_on_Bioethics Citation2012b, Barber and Border Citation2015, Dupras-Leduc et al. Citation2018). Decade-long health outcomes in adult survivors of childhood cancer have been reported; however, in many cases, the survivors consented to the follow-up at 18 years or older (Hudson et al. Citation2013). In reproductive medicine, long-term follow-up has not been common due to the importance of live births as a clinical endpoint as well as its relatively short history (Lu et al. Citation2013). In MR, as well as other reproductive procedures such as in vitro fertilization, those who give consent are not unborn children, but prospective parents. Thus, some have suggested making parental consent to long-term follow-up a condition for undergoing MR (Hens et al. Citation2015). However, the parents can withdraw such consent based on the principle for respect for autonomy. Others have recommended that the UK Government should establish a central register facilitating long-term follow-up post-MR (Hawkes Citation2012, Nuffield_Council_on_Bioethics Citation2012a). After consideration of these different recommendations, the UK Human Fertilisation and Embryology Authority (HFEA) ruled only that a long-term follow-up plan must be present in each MR protocol, but the implementation of such plans is not mandatory (HFEA Citation2017).
In regard to the clinical use of CT, clinics in several countries have conducted research into the effectiveness of this procedure in female infertility cases (Ishii Citation2018). In the USA, several adverse events were observed following CT and the procedure was subsequently prohibited (Ishii and Hibino Citation2018). Regarding follow-up after CT, there has been only one report; however, according to that report, one of the families did not respond to repeated requests for the follow-up survey (Chen et al. Citation2016). This report on follow-up post-CT raises the question of whether follow-up post-MR should be left up to physicians, parents, or resultant children.
The present article primarily considers follow-up from practical and ethical standpoints after MR for safeguarding offspring from mitochondrial disease. It rejects mandatory follow-up, instead asserting a voluntary long-term follow-up approach should be tailored to each specific mitochondrial disease in the best interest of the offspring born via MR. It further discusses the wider implications of this follow-up approach in cases of MR for infertility treatment.
Possible risks and consequences of MR
CT, which has the longest history among mitochondrial manipulations, is a modified form of intracytoplasmic sperm injection (ICSI, a treatment for male infertility), in which the cytoplasm from a donor oocyte is aspirated and infused together with a husband’s spermatozoon into oocytes of poor quality using a micropipette (typically 5–6 μm) (Ishii Citation2018). CT has been performed in several countries that had or have no relevant regulations (Ishii and Hibino Citation2018). However, only a single US clinic has conducted a follow-up survey, according to which (Chen et al. Citation2016), the use of CT for infertility treatment helped 13 couples have 17 genetically-related children, with 1 child being diagnosed with a developmental disorder at 18 months of age. Despite its limited scope, the survey concluded that no effect of CT was discernible in the13 surveyed teenagers (1 couple with quadruplets did not respond). However, two different pregnancies were affected by Turner syndrome (one foetus was miscarried, and the other was selectively reduced in a twin pregnancy). Indeed, this high incidence of Turner’s essentially led to the procedure being abandoned in the USA (Ishii Citation2018).
MST replaces most of the cytoplasm in a patient’s oocyte with that from a donor oocyte through transfer of the karyoplast (the nucleus and a small portion of cytoplasm) (Zhang et al. Citation2017) (). PNT also uses karyoplast transfer to largely replace the cytoplasm of a zygote created using a patient’s oocyte with that of a zygote made using a donor oocyte (Zhang et al. Citation2016) (). Compared with CT, MST and PNT are more radical procedures as they involve karyoplast transfer using a larger micropipette (typically 30–40 μm) (Ishii Citation2018), which can disrupt the cytoplasmic organelles and cytoskeleton crucial to the viability of oocytes (Mao et al. Citation2014), when transferring a patient’s nDNA into an enucleated oocyte or zygote. Furthermore, in both PNT and MST, the transferred karyoplast must be fused with the enucleated cytoplasm using electrofusion or treatment with inactivated Sendai virus particles (also called HVJ-E) (Zhang et al. Citation2016, Zhang et al. Citation2017, Ishii Citation2018)(). Data regarding the safety of such fusion techniques in reproductive medicine remain insufficient at present.
Figure 1. The procedures of maternal spindle transfer (MST) and pronuclear transfer (PNT). MST and PNT require oocytes donated from a third-party woman. The both reproductive procedures involve karyoplast biopsy and fusion of a karyoplast and a cytoplasm. After MST, most of the cytoplasm of a reconstituted oocyte derived from a patient’s oocyte is replaced with the cytoplasm of a donor oocyte. The cytoplasm of a reconstituted zygote is largely replaced with that of a zygote created using a donor oocyte and a spermatozoon of patient’s husband. HVJ-E: Hemagglutinating virus of Japan envelope, PB1: first polar body, PB2: second polar body.
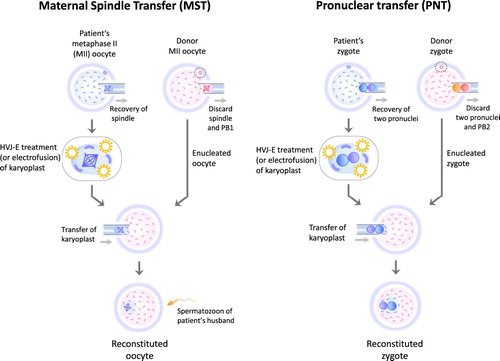
Regarding PNT and MST, only one clinical case of each has been reported. A case of PNT in China was performed for an infertile woman who had experienced embryo arrest in previous IVF cycles (Zhang et al. Citation2016). In that case, electrofusion was used, leading to a triplet pregnancy. However, it ended in no live births; after selective foetal reduction, one of the two remaining foetuses died of respiratory distress and the other of cord prolapse. The sole clinical case of MST was performed, as a bilateral project between the USA and Mexico, for a woman who desired a healthy offspring; electrofusion was also used in this case (Zhang et al. Citation2017). She had suffered four miscarriages for unknown reasons and had two children who had died due to a transmitted MTATP6 mutation in mtDNA that can cause Leigh syndrome (a mitochondrial disease) (). The MST resulted in the birth of a boy who remained healthy at seven months of age. Since 2017, his condition has not been reported. His mtDNA mutation load in tested tissues ranged from 2.36% to 9.23%, which is markedly lower than the over 95% load in the tissues of the mother’s 2 deceased children. However, the residual pathogenic mtDNA may propagate in the future (Kang et al. Citation2016, Yamada et al. Citation2016), potentially leading to reversed heteroplasmy in the child. Moreover, some have warned that mismatches between a patient’s nDNA and donor mtDNA might impair ATP production and lead to uncontrolled leakage of free radicals, (Reinhardt et al. Citation2013) (A). Others disagree with this prediction, arguing that resultant offspring can tolerate mismatches between their parents’ nDNA and donor mtDNA (Kang et al. Citation2016, Yamada et al. Citation2016).
Figure 2. The structure and functions of respiratory chain and its relevance to mitochondrial diseases. ATP is mainly produced through the oxidative phosphorylation (OXPHOS) pathway in mitochondria, where respiratory chain complexes I-IV act in coordination to create a proton (H+) gradient that drives ATP production by the complex V. Electrons (e-) flow from complex I and II to complex III through coenzyme Q (CoQ), from complex III to cytochrome c (Cyt. c), and from cytochrome c to complex IV, where four electrons and protons are used to reduce O2 to H2O. OXPHOS can leak electrons, which may generate free radicals, such as reactive oxygen species (ROS: O. 2-). However, the leakage of free radical production is modulated by the rate of the electron flow. The subunits of the respiratory chain complexes are encoded by nuclear and/or mitochondrial genes. NADH: nicotinamide adenine dinucleotide. The maternally inheritable mitochondrial disease-associated genes in mtDNA that encode the subunits of respiratory complexes are shown. LS: Leigh syndrome, MELAS: mitochondrial myopathy, encephalopathy, lactic acidosis, and stroke-like episodes, MERRF: myoclonus epilepsy associated with ragged-red fibers. Note that there are other mtDNA genes that are known to cause LS (MTFMT, MTTV, MTTK, MTTW, MTTL1), MELAS (MTTL1, MTTQ, MTTH, MTTK, MTTC, MTS1, MTTS2) and MERRF (MTTK, MTTF, MTTL1, MTTH, MTTS1, MTTS2). The mitochondrial disease-associated genes are based on the information in the OMIM database (https://www.omim.org as of 9 April, 2019). Under each disease name, the timing of onset is also indicated, which is based on the information in the aforementioned OMIM data base and the website of the Japan Muscular Dystrophy Association (https://www.jmda.or.jp/mddictsm/mddictsm2/mddictsm2-13/ last accessed 9 April, 2019).
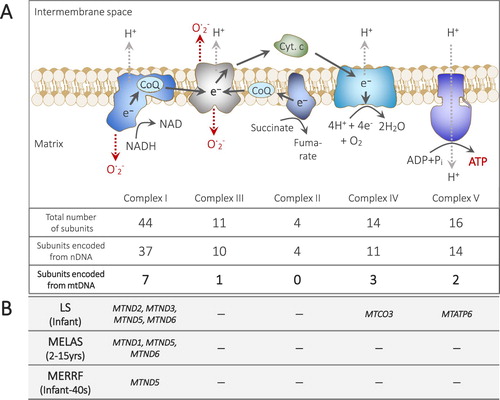
The aforementioned considerations on the risks of mitochondrial manipulations in the oocytes and zygotes suggest that the negative consequences immediately after MR for preventing mitochondrial disease include aneuploid foetuses, miscarriages and stillbirths. Even if live births occur, some of the resultant offspring might later develop mitochondrial or other unexpected diseases. In addition, it has been suggested that the resultant children born via MR and their families may later confront difficulties with their identity because the children are born via ‘three-parent-IVF’ (Scully Citation2017). Thus, follow-up is indispensable for ensuring the physical and mental health of individuals born from MR.
Intergenerational follow-up and transfer of exclusively male embryos
The resultant females born via MR may be at risk of transmitting unremoved pathogenic mtDNA mutations to subsequent generations via maternal inheritance. For this reason, the need for intergenerational follow-up has been emphasized in previous and on-going discussions on the clinical use of MR (Nuffield_Council_on_Bioethics Citation2012b, Barber and Border Citation2015, Dupras-Leduc et al. Citation2018). Conversely, to completely avoid this, a policy of transferring only male embryos in initial MR trials has been proposed (Board_on_Health_Sciences_Policy_Institute_of_Medicine. Citation2016).
One could compare this policy to the use of a preimplantation genetic diagnosis (PGD) to avoid sex-linked diseases by selecting embryos of a particular chromosomal sex. However, the aforementioned risks of MR may be more substantial than those of the embryonic cell biopsies performed in PGD (Heijligers et al. Citation2018). In addition, this policy of only transferring male embryos could send a problematic message to society that only male children, as the first generation, should be placed at the risk of potential MR hazards (Ishii and Palacios-González Citation2017). Although the policy of transferring only male embryos was also discussed in the UK (HFEA Citation2013), it was eventually abandoned in the Human Fertilisation and Embryology (Mitochondrial Donation) Regulations 2015 (UK_Department_of_Health Citation2015).
If counties permit MR for the purpose of safeguarding genetically-related offspring from serious mitochondrial disease, this reproductive technique should be implemented with due consideration of the potential risks and the need of appropriate follow-up, regardless of the future child’s gender. Moreover, the cases of CT in the USA and PNT in China suggest that regulators will intervene in the clinical use of MR when adverse events occur in the first generation (Ishii and Hibino Citation2018). Likewise, the HFEA requires licensed clinics to report adverse incidents after MR (HFEA Citation2017). The follow-up of first-generation individuals born from MR is further discussed below.
Mandatory follow-up of offspring born via MR
Even if prospective parents consent to follow-up post-MR, such consent is unlikely to guarantee that the follow-up will actually be performed in the resultant children for several reasons. Some follow-up investigations may be suspended due to the withdrawal of parental consent. Indeed, parents who joined the bilateral MST study ultimately requested that no further genetic testing be undertaken for their child (Zhang et al. Citation2017). In addition, some families may become lost to follow-up due to relocating, thereby preventing clinics from contacting them.
Possible measures for ensuring follow-up include legally enforcing it upon all families concerned (UK_Department_of_Health Citation2014). For instance, a detailed neurodevelopmental assessment at 18 months old, at which point all children would be expected to be walking and talking (Craven et al. Citation2018), appears to be feasible as a mandatory follow-up for all children born via MR in the UK. However, such neurodevelopmental assessment at 18 months old would constitute only a single and immediate follow-up of children born from MR in the UK (HFEA Citation2017). Longer follow-up would also be crucial, because some of the resultant children might later develop mitochondrial or unexpected diseases, as mentioned above.
Nevertheless, mandatory follow-up of all families concerned may become problematic over time. Accumulating travel and medical expenses impose great burdens in the absence of financial aid. Families must continue to visit the clinic regularly, which is time-consuming and laborious. More importantly, repeated medical examinations could impose a serious burden on children born from MR. In the bilateral MST study, whole blood and tissue samples, including buccal swabs, skin tissues, hair follicles and urine precipitates, were provided for testing (Zhang et al. Citation2017). Although mtDNA profiling of multiple tissues is necessary to systemically assess mtDNA mutation loads, such ongoing and invasive sampling could cause pain or distress in the resultant offspring. In addition, such long-term follow-up could infringe on the family’s privacy or dignity. The parents of quadruplets who did not respond to repeated requests for follow-up after CT (Chen et al. Citation2016) might have considered that their participation could lead to unwanted attention in light of their delivery of four children using ‘three-parent-IVF’.
Mandatory follow-up violates the autonomy of parents and offspring born via MR. Additionally, longer such follow-up is likely to be even more problematic, due to the aforementioned financial, physical, and psychological burdens imposed upon the families. These ethical and practical considerations lend support to the HFEA policy of requiring only that a long-term follow-up plan be put in place for each MR protocol. Nonetheless, appropriate follow-up post-MR should be carried out to ensure the welfare of the resultant individuals.
Voluntary follow-up post-MR
It is essential to consider voluntary follow-up post-MR for a long but realistic period. To reduce the physical, psychological and financial burdens of follow-up, it is necessary to establish the minimum follow-up period to ensure the health of offspring born via MR. MR can be used prenatally to prevent certain mitochondrial diseases that are associated only with mtDNA gene mutations (B). Leigh syndrome and mitochondrial myopathy, encephalopathy, lactic acidosis, and stroke-like episodes (MELAS) are primarily observed in infants and teenagers (OMIM_data_base). Similarly, two different myoclonus epilepsy associated with ragged-red fibres (MERRF) cases that were respectively associated with a MTTS1 mutation and a MTND5 mutation also developed in teenagers (B and legend)(Nakamura et al. Citation1995, Naini et al. Citation2005). On the other hand, MERRF can develop around 40 years of age (OMIM_data_base)(B). Therefore, in cases of Leigh syndrome, MELAS and earlier-onset types of MERRF, the clinically required follow-up period can be determined as approximately two decades to confirm the prevention of mitochondrial disease and the absence of adverse events for several years after the presumed timing of onset of mitochondrial disease. In this follow-up post-MR, mental health care can be provided for the adolescents who confront difficulties with their identity. The period of two decades corresponds with the initial plan of the bilateral MST project for follow-up until the boy reached 18 years of age (Zhang et al. Citation2017). Nonetheless, although this follow-up plan appears to be appropriate, the parents decided that they wanted for their child to undergo no further testing, possibly because the aforementioned risks of MR had not been sufficiently explained to them (Alikani et al. Citation2017). Therefore, before prospective parents give their consent to MR, they must be carefully informed of the aforementioned risks, including aneuploid foetuses, miscarriages, stillbirths and later onset of mitochondrial or other unexpected diseases, in addition to the fact that there are other uncertainties arising from the practice of MR involving genetic intervention to the human eggs or embryos. In addition, physicians should give an unequivocal explanation to the prospective parents as to why their children born via MR must be monitored at least until the presumed timing of onset of the relevant mitochondrial disease.
In the case of women with a pathogenic mtDNA mutation, and their husbands, who do not insist on being genetically related to their future children, other family-building options are available, such as directly using donor oocytes or adopting a child. Given that using MR to safeguard genetically-related children from serious mitochondrial disease is a responsible way to exercise one’s reproductive freedom (Ishii and Palacios-González Citation2017), prospective parents should reinforce their responsible decision by ensuring that their children receive adequate follow-up which exceeds the presumed timing of the onset of a specific mitochondrial disease.
We also have to consider the rights of the offspring born via MR. The moral acceptability of MR depends on whether or not the procedure safeguards the child's right to an open future (Bredenoord et al. Citation2011). However, even if offspring are born healthy after experimental MR, it is important to perform appropriate follow-up in order to ensure the child’s open future without mitochondrial disease. In this regard, it is noteworthy that, based on the above-mentioned follow-up survey after CT, only 1 out of 12 parents disclosed the use of CT to their children, which is lower than the previously reported disclosure rate after the direct use of oocyte donation (Chen et al. Citation2016). To ensure that the children understand the need of long-term follow-up and continue undergo it, parents must inform them of the fact that they were born via experimental MR (Appleby Citation2015).
Given that the resultant individuals may suffer from late side effects of MR, an adequate follow-up plan might extend several decades, particularly in cases in which MR is used to prevent later-onset types of MERRF and even Leigh syndrome and MELAS. In contrast, the resultant children will likely wish to suspend such long-term follow-up in order to pursue higher education, start a career, or relocate, and this desire could clash with and indeed override the parental consent to engage in follow-up. What is best for reproductive scientists is not necessarily best for individuals born via experimental reproductive medicine (Cwik Citation2017). Because the offspring in this scenario would no longer be minors, they should be, in principle, allowed to exercise their own autonomy regarding follow-up post-MR. Again, since MR intends to safeguard the child’s right to an open future without mitochondrial disease, the fundamental requirement of autonomy for these young adults must also be premised on the fact that they have been adequately prevented from having mitochondrial disease till then. Therefore, provided that there is medical proof of absence of symptoms in the central nervous system, muscles and other tissues, as well as evidence of sustained low mtDNA mutation loads (OMIM_data_base), the resultant children who become legally competent should be entitled to refuse further follow-up that they find inappropriately burdensome. This offspring-centred long-term follow-up approach would be applicable to the clinical research of MR to safeguard future children from different types of mitochondrial disease ().
Figure 3. An offspring-centred long-term follow-up approach for the clinical research of mitochondrial replacement (MR) to safeguard future children from different types of mitochondrial disease. LS: Leigh syndrome, MELAS: mitochondrial myopathy, encephalopathy, lactic acidosis, and stroke-like episodes, MERRF: myoclonus epilepsy associated with ragged-red fibers.
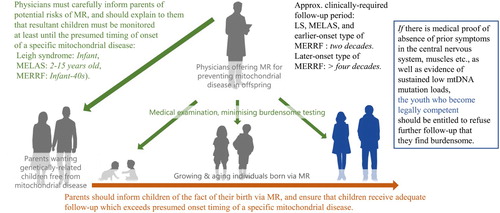
MR for infertility treatment and follow-up
Women who have had children affected by mitochondrial disease frequently experience miscarriages (White et al. Citation1999, Zhang et al. Citation2017). This fact as well as the clinical outcomes of CT in the USA suggest that MR can be effective for infertile women who have only experienced embryonic arrests, implantation failures or miscarriages due to their mtDNA variant and have not had children affected with mitochondrial disease.
In the UK, the use of MR for that purpose is currently unlawful (UK_Department_of_Health Citation2015). However, in the era of reproductive tourism, the unregulated, widespread use of MR to treat infertility might cause many health problems internationally. Indeed, MR has already been practiced for infertility treatment in countries without specific legislation pertinent to MR, such as Ukraine (Ishii and Hibino Citation2018). In regard to the clinical research of CT for treating female infertility, the only reported follow-up survey was not planned initially; it was later approved in 2013, approximately a decade after the research was suspended, with the results being reported in 2016 (Chen et al. Citation2016). In countries with no regulations pertinent to MR, professional societies as well as institutional review boards must confirm whether an appropriate follow-up plan is included in the protocol of MR for treating female infertility. Any such plan should an immediate follow-up of the neurodevelopmental assessment at 18 months of age, provided that the fertility physicians can obtain the cooperation of paediatricians. In addition, longer follow-up should also be considered in cases in which MR is used for infertility treatment, due to its experimental status of this application. Meanwhile, the primary endpoint of a healthy live birth in the treatment of infertility, in principle, renders long-term follow-up unnecessary. Nonetheless, if the use of experimental MR by infertile women who want genetically-related children is truly to be a responsible exercise of reproductive freedom, then prospective parents and physicians should consider long-term follow-up post-MR.
Again, the most important question is how long the resultant offspring born via MR should be followed up. To some extent, the offspring-centred long-term follow-up approach that was developed above could also be applicable to the use of MR for infertility treatment. As the child's right to an open future includes health and fertility as fundamental features for all their life, offspring born via MR for infertility treatment should be monitored up to adolescence to assess their fertility. Such a follow-up plan would seem to be both reasonable and feasible, since a similar follow-up has been carried out for the offspring born via ICSI (Pereira et al. Citation2017).
Finally, we should also consider that the widespread introduction of MR for infertility treatment could instantly lead to potentially controversial applications, such as for lesbian couples who desire a genetic link of both partners to the child (Baylis Citation2018, Cavaliere and Palacios-Gonzalez Citation2018). In many countries other than the UK, it is morally imperative for physicians, prospective parents, and policy-makers to deliberate on whether or not each use of MR is a responsible exercise of reproductive freedom, and whether each use avoids any infringement on the child's right to an open future. If a use of experimental MR is justifiable, the ethics of follow-up post-MR must be carefully discussed to protect and respect the resulting individuals’ human rights.
Conclusion
Long-term follow-up post-MR to safeguard children from serious mitochondrial disease should not simply be left up to physicians, parents, or the resultant children. Physicians and parents should make a coordinated effort to ensure a practical and humane offspring-centred follow-up, while also conditionally entitling the resulting children to refuse follow-up. Physicians must carefully inform couples of the risks of MR and the importance of long-term follow-up tailored to their specific mitochondrial disease. Given that the use of MR is a responsible exercise of one’s reproductive freedom, parents should reinforce this responsible decision by ensuring that the follow-up is performed properly and in the best interests of their offspring. Upon becoming legally competent, the resulting children should be entitled to refuse follow-up if there is medical proof that mitochondrial disease has been adequately prevented till then. This offspring-centred long-term follow-up approach could also be applicable to the use of MR for infertility treatment, which is currently offered and practiced in countries other than the UK.
Funding details
This work was supported by the Uehiro Foundation on Ethics and Education and the Japan Society for the Promotion of Science KAKENHI Project Innovative Ethics (Graduate School of Humanities, Kobe University, Kobe, Japan).
Disclosure statement
No potential conflict of interest was reported by the author.
ORCID
Tetsuya Ishii http://orcid.org/0000-0002-7085-7750
Notes on contributor
Tetsuya Ishii is a professor at the Office of Health and Safety, Hokkaido University, Japan. His interests are the ethics of genetic engineering, stem cells, and reproductive medicine. Ishii was a speaker at the First and Second International Summit on Human Genome Editing in 2015 and 2018.
Correspondence to: Tetsuya Ishii, Office of Health and Safety, Hokkaido University, Sapporo 060-0808, Japan. Email: [email protected]
References
- Alikani, M., et al., 2017. First birth following spindle transfer for mitochondrial replacement therapy: hope and trepidation. Reproductive biomedicine online, 34, 333–336. doi: 10.1016/j.rbmo.2017.02.004
- Appleby, J.B., 2015. The ethical challenges of the clinical introduction of mitochondrial replacement techniques. Medicine, health care and philosophy, 18, 501–514. doi: 10.1007/s11019-015-9656-3
- Barber, S., and Border, P., 2015. Mitochondrial donation. House of Commons Library SN/SC/6833.
- Baylis, F., 2018. ‘No’ to lesbian motherhood using human nuclear genome transfer. Journal of medical ethics, 44, 835–842. doi: 10.1136/medethics-2018-104860
- Board_on_Health_Sciences_Policy_Institute_of_Medicine. 2016. In: Claiborne, A., English, R. & Kahn, J. (eds.) Mitochondrial Replacement Techniques: Ethical, Social, and Policy Considerations. Committee on the, Ethical Social Policy Considerations of Novel Techniques for Prevention of Maternal Transmission of Mitochondrial, D. N. A. Diseases. National Academies of Sciences, Engineering Medicine. Washington (DC): National Academies Press (US). Copyright 2016 by the National Academy of Sciences. All rights reserved.
- Bredenoord, A.L., et al., 2011. Ethics of modifying the mitochondrial genome. Journal of medical ethics, 37, 97–100. doi: 10.1136/jme.2010.037481
- Cavaliere, G. and Palacios-Gonzalez, C., 2018. Lesbian motherhood and mitochondrial replacement techniques: reproductive freedom and genetic kinship. Journal of medical ethics, 44, 835–842. doi: 10.1136/medethics-2017-104450
- Chen, S.H., et al., 2016. A limited survey-based uncontrolled follow-up study of children born after ooplasmic transplantation in a single centre. Reproductive biomedicine online, 33, 737–744. doi: 10.1016/j.rbmo.2016.10.003
- Craven, L., et al., 2018. Scientific and ethical issues in mitochondrial donation. The new bioethics, 24, 57–73. doi: 10.1080/20502877.2018.1440725
- Cwik, B., 2017. Designing ethical trials of Germline gene Editing. New England journal of medicine, 377, 1911–1913. doi: 10.1056/NEJMp1711000
- Dupras-Leduc, R., Birko, S., and Ravitsky, V., 2018. Mitochondrial/nuclear transfer: A Literature review of the ethical, Legal and Social issues. Canadian journal of bioethics/revue canadienne de bioéthique, 1, 1–17.
- Hawkes, N., 2012. Intervention to prevent transmission of mitochondrial disorders should be allowed, subject to safeguards, report says. BMJ, 344, e4089. doi: 10.1136/bmj.e4089
- Heijligers, M., et al., 2018. The cognitive and socio-emotional development of 5-year-old children born after PGD. Human Reproduction, 33, 2150–2157. doi: 10.1093/humrep/dey302
- Hens, K., Dondorp, W., and de Wert, G., 2015. A leap of faith? An interview study with professionals on the use of mitochondrial replacement to avoid transfer of mitochondrial diseases. Human reproduction, 30, 1256–1262. doi: 10.1093/humrep/dev056
- HFEA. 2013. Human Fertilisation and Embryology Authority (HFEA). Mitochondria replacement consultation: Advice to Government. Available from: https://www.hfea.gov.uk/media/2618/mitochondria_replacement_consultation_-_advice_for_government.pdf [Accessed 30 October 2018].
- HFEA, 2017. Human Fertilisation and Embryology Authority (HFEA). Code of Practice 8th Edition, follow-up arrangements (p290). Available from: https://ifqlive.blob.core.windows.net/umbraco-website/1561/2017-05-02-code-of-practice-update-may-2017-final.pdf [Accessed 31 October 2018].
- Hudson, M.M., et al., 2013. Clinical ascertainment of health outcomes among adults treated for childhood cancer. Jama, 309, 2371–2381. doi: 10.1001/jama.2013.6296
- Hyslop, L.A., et al., 2016. Towards clinical application of pronuclear transfer to prevent mitochondrial DNA disease. Nature, 534, 383–386. doi: 10.1038/nature18303
- Ishii, T., 2014. Potential impact of human mitochondrial replacement on global policy regarding germline gene modification. Reproductive biomedicine online, 29, 150–155. doi: 10.1016/j.rbmo.2014.04.001
- Ishii, T., 2016. Mitochondrial Manipulation for infertility treatment and disease prevention. In: H. Schatten, ed. Human Reproduction: Updates and New Horizons. New Jersey: Wiley Blackwell, 205–230.
- Ishii, T., 2017. Mitochondrial replacement techniques and Mexico's rule of law: on the legality of the first maternal spindle transfer case. Journal of law and the biosciences, 4, 384–390. doi: 10.1093/jlb/lsx015
- Ishii, T., 2018. Reproductive medicine involving mitochondrial DNA modification: Evolution, legality, and ethics. EMJ Repro health, 4, 88–99.
- Ishii, T., and Hibino, Y., 2018. Mitochondrial manipulation in fertility clinics: Regulation and responsibility. Reproductive biomedicine & society online, 28, 93–109. doi: 10.1016/j.rbms.2018.01.002
- Ishii, T., and Palacios-Gonz Lez, C., 2017. Mitochondrial replacement techniques: genetic Relatedness, gender implications, and Justice. Gender and the genome, 1, 129–134. doi: 10.1089/gg.2017.0016
- Kang, E., et al., 2016. Mitochondrial replacement in human oocytes carrying pathogenic mitochondrial DNA mutations. Nature, 540, 270–275. doi: 10.1038/nature20592
- Koopman, W.J., Willems, P.H., and Smeitink, J.A., 2012. Monogenic mitochondrial disorders. New England journal of medicine, 366, 1132–1141. doi: 10.1056/NEJMra1012478
- Lu, Y.H., Wang, N., and Jin, F., 2013. Long-term follow-up of children conceived through assisted reproductive technology. Journal of zhejiang university science B, 14, 359–371. doi: 10.1631/jzus.B1200348
- Mao, L., et al., 2014. Behaviour of cytoplasmic organelles and cytoskeleton during oocyte maturation. Reproductive BioMedicine Online, 28, 284–299. doi: 10.1016/j.rbmo.2013.10.016
- Naini, A.B., et al., 2005. Novel mitochondrial DNA ND5 mutation in a patient with clinical features of MELAS and MERRF. Archives of neurology, 62, 473–476. doi: 10.1001/archneur.62.3.473
- Nakamura, M., et al., 1995. A novel point mutation in the mitochondrial tRNA(Ser(UCN)) gene detected in a family with MERRF/MELAS overlap syndrome. Biochemical and biophysical research communications, 214, 86–93. doi: 10.1006/bbrc.1995.2260
- Newcastle_University. 2017. Newcastle awarded world's first mitochondrial licence. Published on: 16 March 2017. Available from: https://www.ncl.ac.uk/press/articles/archive/2017/03/mitochondrialicence/ [Accessed 9 April 2019].
- Nuffield_Council_on_Bioethics. 2012a. Novel techniques for the prevention of mitochondrial DNA disorders: an ethical review. Available from: http://nuffieldbioethics.org/project/mitochondrial-dna-disorders/ [Online].
- Nuffield_Council_on_Bioethics, 2012b. Novel techniques for the prevention of mitochondrial DNA disorders: an ethical review. Available from: http://nuffieldbioethics.org/project/mitochondrial-dna-disorders/ [Accessed 17 August, 2018].
- OMIM_Data_Base Content #256000 (Leigh syndrome), #540000 (MELAS), and #545000 (MERRF). Available from: https://www.omim.org/ [Accessed 9 April 2019].
- Palacios-Gonzalez, C., and Medina-Arellano, M.J., 2017. Mitochondrial replacement techniques and Mexico's rule of law: on the legality of the first maternal spindle transfer case. Journal of law and the biosciences, 4, 50–69. doi: 10.1093/jlb/lsw065
- Pereira, N., et al., 2017. The safety of intracytoplasmic sperm injection and long-term outcomes. Reproduction, 154, F61–f70. doi: 10.1530/REP-17-0344
- Reinhardt, K., Dowling, D.K., and Morrow, E.H., 2013. Medicine. mitochondrial replacement, evolution, and the clinic. Science, 341, 1345–1346. doi: 10.1126/science.1237146
- Sample, I. 2018. UK doctors select first women to have ‘three-person babies’. The Guardian on 1 February 2018. Available from: https://www.theguardian.com/science/2018/feb/01/permission-given-to-create-britains-first-three-person-babies [Accessed 31 October 2018].
- Scully, J.L., 2017. A mitochondrial Story: mitochondrial replacement, identity and Narrative. Bioethics, 31, 37–45. doi: 10.1111/bioe.12310
- UK_Department_of_Health. 2014. Mitochondrial donation: A consultation on draft regulations to permit the use of new treatment techniques to prevent the transmission of a serious mitochondrial disease from mother to child. Available from: https://www.gov.uk/government/uploads/system/uploads/attachment_data/file/285251/mitochondrial_donation_consultation_document_24_02_14_Accessible_V0.4.pdf [Last Accessed 17 August, 2018].
- UK_Department_of_Health, 2015. The Human Fertilisation and Embryology (Mitochondrial Donation) Regulations 2015 No. 572. Available from: https://www.legislation.gov.uk/ukdsi/2015/9780111125816/contents [Acessed 17 August, 2018].
- White, S.L., et al., 1999. Genetic counseling and prenatal diagnosis for the mitochondrial DNA mutations at nucleotide 8993. The American journal of human genetics, 65, 474–482. doi: 10.1086/302488
- Winkler, J. 2018. Australia and Singapore Work Towards Legal Mitochondrial Replacement Therapy. The Pop Topic. July 2, 2018. Available from: https://thepoptopic.com/australia-and-singapore-work-towards-legal-mitochondrial-replacement-therapy/ [Accessed 17 August, 2018].
- Yamada, M., et al., 2016. Genetic Drift can compromise mitochondrial replacement by nuclear transfer in human oocytes. Cell Stem cell, 18, 749–754. doi: 10.1016/j.stem.2016.04.001
- Zhang, J., et al., 2016. Pregnancy derived from human zygote pronuclear transfer in a patient who had arrested embryos after IVF. Reproductive biomedicine online, 33, 529–533. doi: 10.1016/j.rbmo.2016.07.008
- Zhang, J., et al., 2017. Live birth derived from oocyte spindle transfer to prevent mitochondrial disease. Reproductive BioMedicine Online, 34, 361–368. doi: 10.1016/j.rbmo.2017.01.013