Abstract
The successful formulation of two-part condensation-curing silicone materials via purely organic catalysis is here developed and investigated as fouling-release coating. Several non-toxic organic catalysts and their ‘onium’ salts were studied as potential purely organic catalysts on the silicone condensation reaction but also on the properties of the resulting cured resins. The trifluoroacetic acid was pointed suitable for the production of condensation-curing silicone coatings. To assess favorable fouling-release properties, multi-wall carbon nanotubes (0.05–0.2 wt-%) were added to the resin. Interestingly, the catalytic activity of the trifluoroacetic acid proved not affected by the presence of the nanocharge. The properties of the coatings prepared using 0.1 wt-% acid revealed constant degree of crosslinking independently of the carbon nanotubes loading and pronounced surface nanostructuring suitable for fouling release. This final property was tested against Ulva linza sporelings and revealed that the coatings are highly suitable for fouling-release applications.
Graphical abstract
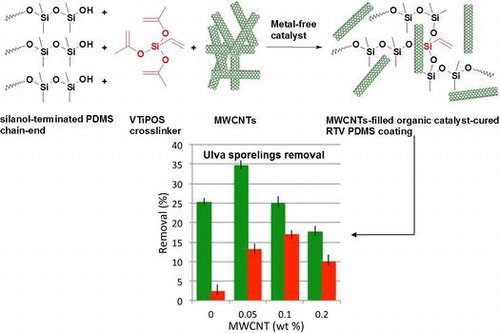
Introduction
Marine biofouling, the settlement and colonization of micro- and macro-organisms on submerged surfaces is a major problem in the maritime and aquaculture industries with shipping, infrastructure, and scientific monitoring equipment being adversely affected.Citation1–3 For ships, marine biofouling may result in up to an 86% penalty in power output,Citation4 decreased vessel speed, an increased fuel consumption of up to ~40%,Citation2 elevated dry-dock cleaning expenses, hull corrosion, and loss of hull strength.Citation3 Combating biofouling routinely includes the use of antifouling paints (AFPs). To date, the most common mode-of-action of AFPs is based on the slow release of biocide with time. Such coatings are described as self-polishing (erode with time) and possess excellent antifouling properties.Citation5 Introduced in the late 1950s, commercial AFPs with the broad-spectrum biocide tributyltin were banned in 2008 by the IMO due to their high toxicity toward non-target species.Citation6 In response, industry has reacted by replacing tin-containing compounds with zinc or copper systems, which may also be toxic toward non-target aquatic organisms.Citation7 One alternative to AFPs is biocide-freeCitation8 fouling-release coatings (FRCs). Based mainly on fluorine or silicone-containing formulations, these low-adhesion coatings promote low adhesion/removal of organisms by hydrodynamic forces as a result of their low elastic modulus and surface energy, but may also disrupt marine organism settlement by the formation of a hydrated layer close to their surface.Citation9
For silicone-based formulations, commercially available FRCs are a duplex system consisting of a tie-coat applied on an anticorrosive epoxy primer, which ensures adhesion, and a fouling-release top-coat, based on a filler-reinforced, crosslinked poly(dimethyl siloxane) (PDMS) elastomer.Citation10 Fillers are often inorganic particles, such as silica (SiO2), calcium carbonate (CaCO3), or titanium dioxide (TiO2), but novel compounds, such as multi-wall carbon nanotubes (MWCNTs) have been used previously with good efficacy.Citation11a,b While the addition of MWCNTs did not markedly reinforce the PDMS, the hydrophilicity of the elastomer and the critical removal stress of adult barnacles increased sharply. This ‘detachment of biofoulants’ strategy has led to the development of efficient and durable new silicone-based FRCs.Citation12 Crosslinking of PDMS resin usually proceeds via two types of curing reaction, either hydrosilylation or condensation, involving respectively platinum- or tin-based catalysts which are potentially toxic and are found to bioaccumulate in fish, seal, and other marine organisms.Citation13 If the potential of nanofillers as efficient additives in FRCs is to be realized, there is an urgent need to develop alternatives to metal-based catalysts for the PDMS curing reactions. Organic compounds, (1,8-diazabicyclo[5.4.0]undec-7-ene (DBU), p-toluenesulfonic acid (PTSA), trifluoroacetic acid (TFA)), and their mixtures, may provide an alternative as they are known to combine catalytic activity and low toxicity.Citation14–22
This study describes the preparation of new metal-free carbon nanotube-based PDMS nanostructured coatings via purely organic catalysis and discusses their applicability to the strategy of fouling release.
Experimental methods
Materials
Silanol-terminated PDMS ( = 26 500 g/mol, Ð = 1.83, Biocyl 2000), a premix of silanol-terminated PDMS with 0.5 wt-% MWCNTs (average diameter = 10 nm, length = 0.1–10 μm, carbon purity = min. 90%, Biocyl 2105, Nanocyl, Belgium), and a crosslinker (vinyl triisopropenoxysilane, VTiPOS, MW = 226.36 g/mol; ρ = 0.926 g/cm3, Nanocyl) were used as received. Trimethylsiloxy-terminated PDMS (PDMS-CH3, MW = 5970 g/mol), dibutyltin dilaurate (DBTL, MW = 631.56 g/mol, ρ = 1.066 g/cm3), 1,8-diazabicyclo[5.4.0]undec-7-ene (MW = 152.24 g/mol, ρ = 1.019 g/cm3), p-toluenesulfonic acid (PTSA, MW = 172.2 g/mol, ρ = 1.24 g/cm3), and trifluoroacetic acid (TFA, MW = 114.02 g/mol, ρ = 1.489 g/cm3) were supplied by VWR, Belgium. Amorphous hexamethyldisilazane-treated silicone dioxide nanoparticles (SiO2, d = 20 nm, SA = 150–200 m2/g, ρ = 2.2 g/cm3, ABCR, Belgium) were used as additives for PDMS reinforcement.
Formulations
In all experiments, formulations consisting of two parts, A and B, were prepared. In the absence of MWCNTs, part A was prepared as follows: the silanol-terminated PDMS (Biocyl 2000) was mixed with SiO2 ([silanol-terminated PDMS]/[SiO2] = 99/1, 98/2 or 97/3 (wt/wt)) and crosslinker (VTiPOS, [silanol-terminated PDMS]/[crosslinker] = 95/5 (wt/wt)). It is further denoted as Biocyl. The amount of Si was varied in order to regulate formulation viscosity. In the presence of MWCNTs, part A consisted of a premix of silanol-terminated PDMS (Biocyl 2105) containing 0.5 wt-% MWCNTs, diluted with Biocyl 2000 to a [MWCNTs] = 0.05, 0.1 or 0.2 wt-%; 2 wt-% SiO2 and 5 wt-% VTiPOS. It is further denoted MWCNTs/Biocyl.
Part B consists of PDMS-CH3 with a predetermined amount of catalyst. DBTL was used as reference and DBU, PTSA, TFA, and mixtures of DBU/TFA were studied as possible organic catalysts for PDMS crosslinking in the presence or not of MWCNTs. In accordance with the supplier recommendations, parts A and B were mixed together mechanically (1200 rpm, 30 min) at a weight ratio of 10:1.
Equipment
Viscosity (η, cP) measurements were performed with a DV-II viscometer (Brookfield, USA) at 25 ± 0.1 °C and a rate of 1 rpm. Samples were allowed to stabilize for 10 min prior to measurement.
For the swelling tests, pre-weighed dry rectangular samples (1 cm2) of the crosslinked formulations were immersed in 100 ml of heptane at 25 ± 0.1 °C. At regular time intervals, samples were taken out, the excess surface heptane was gently removed with filter paper, and the swollen samples were weighed. After reaching equilibrium, the samples were dried overnight at 70 °C under vacuum. The equilibrium degree of swelling (αeq, %) was calculated in grams of solution per gram of dry polymer at time t, according to Equation (1):(1)
where wt is the weight of the sample at time t and w0 the weight of the dried sample. The values of αeq are the average of at least three samples.
The tensile modulus (MPa) of the samples was analyzed at room temperature with a dynamic mechanical analyzer DMA (TA Instrument 2980) operating in tensile strain-sweep mode. A frequency of 1 Hz, a preload of 0.1 N, and amplitude from 0.5 to 27 mm were used. The results are the average of at least three measurements.
Static contact angle measurements were performed on nanofilled silicone-coated microscopic slides using a DSA 10 apparatus (Kruss, Germany) and the sessile drop technique. The hydrophobic/hydrophilic character of the PDMS layer was evaluated by measuring the contact angle between the surface of the coating and drops of demineralized water (50 μl). The results are the average of at least 10 determinations.
Fouling-release assays
Fertile thallus tissue of Ulva linza was collected from Llanwit Major beach, Glamorgan (52° 23′ N; 3° 30′ W) and zoospores were released into ‘Tropic Marin’ artificial seawater (ASW; Aquarientechnik GmbH). Assays were based on those described previously,Citation11,23,24 with experimental surfaces being leached in recirculating de-ionized water for 20 weeks prior to assay (to allow DBTL to leach out of the coating). Briefly, five replicate slides of leached experimental coatings were added to individual compartments of Quadriperm dishes. Ten milliliters of a suspension of zoospores (1.5 × 106 spores ml−1) were added to each compartment and dishes were incubated for 1.5 h in the dark to allow spores to settle. Slides were then washed to remove non-attached (swimming) spores, before growth medium was added and spores were allowed to germinate and grow on a 16:8 light:dark cycle at 18 °C for 6 days. This culturing regime produced a green ‘lawn’ of small plants (sporelings) over the surface of each experimental coating. The sporeling biomass on each coating was determined, both prior to and post the removal step, via the autofluorescence of chlorophyll a using an adapted plate reader (Tecan, Reading, UK). The adhesion strength of sporelings to the experimental surfaces was determined by exposure to a 41.9 Pa wall shear stress in a proprietary flow-channel apparatus.Citation24 Adhesion strength is represented as a percentage removal of the initial sporeling biomass on each coating.
Results and discussion
Condensation curing of silicone coatings via purely organic catalysis
Two types of reaction are mostly used for curing silicone elastomers: high-temperature hydrosilylation curing and room-temperature vulcanizing (RTV) via condensation reaction (Scheme ).Citation13 While hydrosilylation curing is applicable in the rubber industry, where the equipment for mixing, transfer, and especially heating is available, its applicability for direct ship-hull coating appears much more difficult. For this particular application, the RTV PDMS resins seem more appropriate. Therefore, the formulation of an applicable (in coatings) condensation curing-PDMS material via purely organic catalysis was targeted in this research.
From previous studies on the hydrosilylation curing of commercially available Sylgard 184,Citation11 the condensation-curing PDMS resin, Biocyl 2000, was selected for the study. PTSA, TFA, DBU, and mixtures DBU/TFA (‘onium’ salts) were studied as potential organic catalysts. Their chemical structures are presented in Fig. .
Figure 1 a Chemical structure of p-toluenesulfonic acid (PTSA); b 1,8-diazabicyclo[5.4.0]undec-7-ene (DBU); c trifluoroacetic acid (TFA) and d DBU/TFA ‘onium’ salt
![Figure 1 a Chemical structure of p-toluenesulfonic acid (PTSA); b 1,8-diazabicyclo[5.4.0]undec-7-ene (DBU); c trifluoroacetic acid (TFA) and d DBU/TFA ‘onium’ salt](/cms/asset/493224f5-db6a-41cd-83c8-f49a4fc6f0df/ynan_a_1190167_f0001_b.gif)
Figure 2 Viscosity of the MWCNT/Biocyl mixture and the bulk properties of the resulting cured materials as a function of the MWCNT content. The blue line shows viscosity prior to curing; bars – tensile modulus of MWCNT/Biocyl cured using 0.5 wt-% DBTL (red) or 0.1 wt-% TFA (green) as the catalyst; data labels give the respective αeq
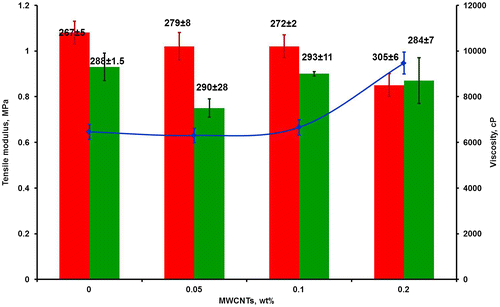
Figure 3 Static contact angles of TFA-catalyzed MWCNT/Biocyl coatings as a function of the MWCNT content. Red bars – before immersion, green bars – after immersion in deionized water for 7 days
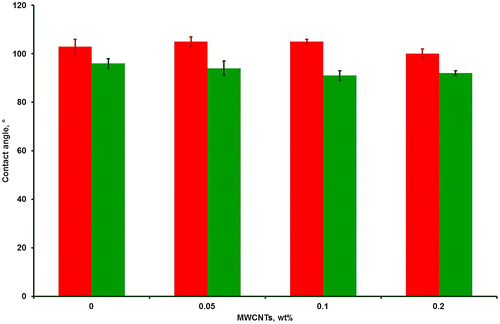
Figure 4 Removal of 6-day-old sporelings of U. linza from surfaces cured using 0.5 wt-% DBTL (red) or 0.1 wt-% TFA (green) by 49 Pa wall shear stress. Expressed as a percentage of the initial density. N = 1080, error bars are ± 2 x Standard Error
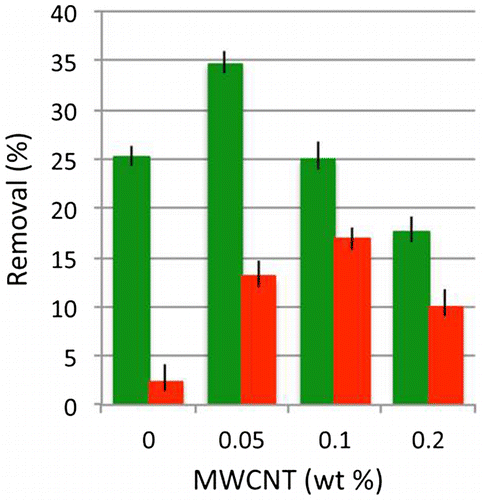
The formulations were prepared as two-part systems in order to facilitate applicability and storage and their viscosity, mechanical, and swelling properties were investigated. For the sake of comparison, condensation curing of Biocyl 2000 was catalyzed by DBTL, a more conventional organo-metallic catalyst. The resin viscosity was adjusted to that of Sylgard 184 in addition to the mechanical and swelling properties of the resulting crosslinked materials, i.e. η = 4980 cP, tensile modulus = 2.64 ± 0.09 MPa and αeq = 92 ± 3%Citation11 by adding SiO2 at weight percentages varying from 0 to 3 wt-%Citation25 (Table ).
Table 1 Viscosity (η) of Biocyl 2000 as a function of SiO2 content as well as the tensile modulus and equilibrium degree of swelling (αeq, %) of the cured materials obtained using DBTL as a catalyst
As expected, the addition of 2 wt-% SiO2 increased the viscosity of the resin before curing to the range measured for Sylgard 184.Citation11 Concerning the bulk properties of the cured silicone, the equilibrium degrees of swelling decreased slightly, in agreement with the increase in tensile modulus, but remained double that of the αeq value recorded for cured Sylgard 184.Citation11 Even in the presence of 2 wt-% SiO2, lower tensile moduli were recorded, probably as a result of lower degrees of crosslinking in the condensation-curing resin. At 3 wt-% loadings of SiO2, the viscosity of the PDMS increased further to a value greater than the applicability range for Sylgard 184, and this formulation was therefore not pursued. Thus, further development of PDMS condensation-curing coatings focused on the 2 wt-% SiO2 containing Biocyl 2000. The results obtained using the catalysts PTSA, TFA, DBU, and mixtures of DBU/TFA at 1:3 or 3:1 (wt/wt) are summarized in Table . The results in Table indicate that TFA, DBU, and their respective mixtures are suitable organic catalysts for the condensation curing of Biocyl. The use of PTSA as a catalyst resulted in zero to poorly crosslinked (tacky) coatings, even after two weeks of curing and very high catalyst concentrations, and it was thus excluded. The concentration of the catalyst markedly altered the crosslinking process with the optimum addition varying depending on the nature of the catalyst.
Table 2 Crosslinking and bulk properties of the resin as a function of the organic catalyst type and concentration (Biocyl 2000, 2 wt.% Si, η = 6460 cP)
For TFA, very fast curing and even fuming were observed at concentrations higher than 0.1 wt-%. Considering the condensation mechanism shown in Scheme b, fuming was ascribed to rapid evaporation of the byproduct (most probably acetone) during crosslinking. For DBU, a similar effect was observed only at very high concentrations (4 wt-%). Notably, no fuming occurred for the ‘onium’ salts formed by the DBU/TFA mixture, at least up to a 2 wt-% loading. These results are consistent with published data on polymerization/condensation reactions catalyzed by DBU and other ‘onium’ salts (DBU/benzoic acid).Citation14,26 In general, the bulk properties of the cured resins improved with increasing catalyst concentration, reaching values comparable to those of the DBTL-cured Biocyl. However, the tensile modulus remained lower than the value recorded for systems obtained in the presence of DBTL and the corresponding equilibrium degree of swelling was higher (Entry 12, Table : tensile modulus = 1.03 ± 0.02 MPa, αeq = 300 ± 7%). As a suitable compromise between catalyst concentration and the recorded bulk properties of the cured resins, TFA was selected for the production of MWCNT-filled silicone coatings.
The effect of MWCNTs on the condensation curing of PDMS organo-catalyzed by TFA
Previous studies have shown the favorable fouling-release properties of MWCNT/PDMS hydrosilylation-curing coatings (Sylgard 184) at MWCNTs loading from 0.05 to 0.2 wt-%.Citation11 Here condensation-cured PDMS coatings of similar MWCNT content were prepared with the organic catalyst TFA. The results are shown in Fig. .
Data in Fig. show that the loading of MWCNT markedly influenced the viscosity and bulk properties of Biocyl 2000. At low loadings (0.05 and 0.1 wt-%) of MWCNTs, the viscosity of the Biocyl was relatively constant, before increasing sharply at a MWCNT content of 0.2 wt-%. These observations are consistent with previous data on MWCNT-filled Sylgard 184Citation27 and are explained by the favorable CH-π interactions. Crosslinking was performed using the organic catalyst TFA at a concentration of 0.1 wt-%. As a comparator, formulations were also prepared using DBTL. In DBTL-cured coatings, the tensile modulus was found to decrease slightly with increasing MWCNT loading and this was accompanied by the respective increase in αeq. Such behavior was unexpected and suggests a lower degree of crosslinking at higher MWCNT loadings. This may be explained by Sn(IV)/MWCNTs interactions. Indeed, MWCNTs have already been functionalized with Sn(IV) oxides by chemical methods.Citation28
This behavior was not observed when TFA was used as the catalyst. In this case, no significant change in either the tensile modulus or the equilibrium swelling was found, thus revealing a constant degree or crosslinking independent of the MWCNT loading.
Since the surface properties of FRCs are of crucial importance, static contact angle measurements were performed on TFA-catalyzed cured MWCNT/Biocyl coatings before and after immersion in demineralized water for 7 days (Fig. ). All data are compared to the values obtained for the unfilled TFA-cured Biocyl. In agreement with published data,Citation11 unfilled Biocyl gave a contact angle of 103 ± 3 ° before immersion in water confirming its hydrophobic nature. The contact angle of all the MWCNT/Biocyl coatings gave similar results, irrespective of the nanofiller loading. The surface wettability of Biocyl and MWCNT/Biocyl increased after immersion in water for 7 days, which suggests that reorganization of the surface had occurred. Such surface reorganization of PDMS in prolonged contact with water is reported to result from conformational changes of the flexible PDMS chains, leading to exposure of Si-O-Si-bonds at the PDMS–water interface.Citation29 Such conformational changes are also known to be reversibleCitation11 and, in this study, appear to be slightly more pronounced for MWCNT-filled FRC coatings than for the unfilled Biocyl 2000 (Fig. ).
Fouling-release performance of MWCNT Biocyl 2000 coatings
Spores of U. linza settled on all coatings and a continuous green ‘lawn’ of sporelings (young plants) was visible after 6 days.
The percentage removal of sporelings of U. linza by 49 Pa wall shear stress in the flow channel is summarized in Fig. . Biomass removal is greater from the coatings cured with the TFA catalyst compared to removal from the DBTL-cured Biocyl 2000. Sporeling removal does not show a linear relationship with increasing loadings of MWCNTs. For the coatings cured with TFA, the maximum average removal (34.7 ± 1.2%) is achieved from the silicone with the lowest MWCNT loading (0.05 wt-%), with the extent of removal decreasing thereafter as MWCNT content rises. In general, DBTL-cured coatings have worse fouling-release properties for U. linza than the organic cured TFA coatings, showing a maximum removal of 16.8 ± 1.1% at a MWCNT loading of 0.1 wt-%. However, the inclusion of MWCNTs in the DBTL-cured system markedly increases the performance of the coatings over the respective unfilled control. This is only the case in the TFA-cured system at the optimal MWCNT loading of 0.05 wt-% which might probably be explained by surface roughness alteration through bulk properties modulation and/or surface restructuring. However, as far as no data on surface structure/roughness are available, any other hypothetical speculations need to be avoided.
Conclusions
The formulation of an applicable in coatings condensation-curing PDMS material via purely organic catalysis was here developed. All formulations were prepared as two-part systems and their viscosity, mechanical, and swelling properties were studied. At first, a formulation with properties close to those of an already applicable for coatings industrial resin was prepared using a classical tin-based catalyst and the addition of 2 wt-% SiO2. Then, PTSA, TFA, DBU, and DBU/TFA ‘onium’ salts depending on their concentrations were investigated as potential purely organic catalysts. As a suitable compromise between catalyst concentration and resin bulk properties, TFA was selected for the production of condensation-curing silicone coatings via purely organic catalysis. Finally, in order to obtain favorable fouling-release properties, MWCNTs at loadings from 0.05 to 0.2 wt-% were added to the resin. The bulk properties and the surface wettability of the coatings prepared using 0.1 wt-% TFA as catalyst revealed a constant degree or crosslinking independently of the MWCNT loading and pronounced surface reorganization suitable for fouling release. Indeed, the percentage removal of U. linza sporelings revealed greater from coatings cured with the TFA catalyst compared to removal from the DBTL-cured Biocyl 2000. Moreover, a maximum in average biomass removal was achieved from TFA-cured coatings containing only 0.05 wt-% MWCNTs. The as-obtained results suggest that the TFA-cured coatings containing only 0.05 wt-% MWCNTs are suitable for applications as FRCs.
Funding
The work was funded by the EC Framework 6 Integrated Project “AMBIO” (Advanced Nanostructured Surfaces for the Control of Biofouling). CIRMAP is grateful to the Région Wallonne and the European Union (FEDER) for financial support through the Phasing-Out of Objectif-1 Hainaut: Materia Nova.
Acknowledgments
This article reflects only the authors’ views and the European Commission is not liable for any use that may be made of information contained therein. The authors also thank Nanocyl S.A. (Sambreville, Belgium) for kindly supplying the PDMS and the carbon nanotubes premix. Michael Claes (Nanocyl) is warmly thanked for numerous relevant discussions and advices. CIRMAP is grateful to the Région Wallonne and the European Union (FEDER) for financial support through the Phasing-Out of Objectif-1 Hainaut: Materia Nova. Ms. Christine Beveridge is thanked for technical assistance with the algal assays.
Disclosure statement
No potential conflict of interest was reported by the authors.
References
- (a) I. Banerjee, R. C. Pangule and R. S. Kane: ‘Antifouling coatings: recent developments in the design of surfaces that prevent fouling by proteins, bacteria, and marine organisms’, Adv. Mater., 2011, 23, 690–718. (b) M. P. Schultz: ‘Effects of coating roughness and biofouling on ship resistance and powering’, Biofouling, 2007, 23, 331–341.10.1002/adma.201001215
- M. A. Champ: ‘A review of organotin regulatory strategies, pending actions, related costs and benefits’, Sci. Total Environ., 2000, 258, 21–71.10.1016/S0048-9697(00)00506-4
- Z. Chen, W. Zhao, J. Xu, M. Mo, S. Peng, Z. Zeng, X. Wu and Q. Xue: ‘Designing environmentally benign modified silica resin coatings with biomimetic textures for antibiofouling’, RSC Adv., 2015, 5, 36874–36881.10.1039/C5RA04658K
- M. P. Schultz: ‘Effects of coating roughness and biofouling on ship resistance and powering’, Biofouling, 2007, 23, 331–341.10.1080/08927010701461974
- R. A. Braithwaite and L. A. McEvoy: ‘Marine biofouling on fish farms and its remediation’, Adv. Mar. Biol., 2005, 47, 215–252.
- International Convention on the Control of Harmful Anti-fouling Systems on Ships. Available from http://www.imo.org/en/About/Conventions/ListOfConventions/Pages/International-Convention-on-the-Control-of-Harmful-Anti-fouling-Systems-on-Ships-(AFS).aspx (accessed 12 December 2015).
- D. A. Borg and L. D. Trombetta: ‘Toxicity and bioaccumulation of the booster biocide copper pyrithione, copper 2-pyridinethiol-1-oxide, in gill tissues of Salvelinus fontinalis (brook trout)’, Toxicol. Ind. Health, 2010, 26, 139–150.10.1177/0748233710362381
- M. Lejars, A. Margaillan and C. Bressy: ‘Fouling release coatings: A nontoxic alternative to biocidal antifouling coatings’, Chem. Rev., 2012, 112, 4347–4390.10.1021/cr200350v
- G. D. Bixler and B. Bhushan: ‘Biofouling: lessons from nature’, Phyl. Trans. R. Soc., 2012, A370, 2381–2417.10.1098/rsta.2011.0502
- E. Robbart: ‘Ship’s hull coated with anti-fouling silicone resin and method of coating’, US Patent 2986474, 30 May 1961.
- (a) A. Beigbeder, P. Degee, S. L. Conlan, R. J. Mutton, A. S. Clare, M. E. Pettit, M. E. Callow, J. A. Callow and P. Dubois: ‘Preparation and characterisation of silicone-based coatings filled with carbon nanotubes and natural sepiolite and their application as marine fouling-release coatings’, J. Biofouling, 2008, 24, 291–302. (b) E. Martinelli, M. Suffredini, G. Galli, A. Glisenti, M. E. Pettitt, M. E. Callow, J. A. Callow, D. Williams and G. Lyall: ‘Amphiphilic block copolymer/poly(dimethylsiloxane) (PDMS) blends and nanocomposites for improved fouling-release’, Biofouling, 2011, 27, 529–541.10.1080/08927010802162885
- A. G. Nurioglu, A. C. C. Esteves and G. de With: ‘Non-toxic, non-biocide-release antifouling coatings based on molecular structure design for marine applications’, J. Mater. Chem. B, 2015, 3, 6547–6570.10.1039/C5TB00232J
- P. D. Folland and J. G. Matisons: ‘Siloxane elastomers’, in ‘Polymeric materials encyclopedia’, Twelve Volume Set, Vol. 1(A-B), (ed. J. C. Salamone), 7759–7768; 1996, Boca Raton, FL, CRC Press.
- G. K. Kakade, B. R. Madje and R. U. Pokalwar: ‘DBU catalyzed Knoevenagel condensation under ultrasonic irradiation’, Indian J. Het. Chem., 2008, 17, 379–380.
- ORL-RAT LD50 820 mg kg−1. Oxford University Chemical Safety Data. http://msds.chem.ox.ac.uk/index2.html. ( No longer updated).
- B. Baghernejad: ‘Application of p-toluenesulfonic acid (PTSA) in organic synthesis’, Curr. Org. Chem., 2011, 15, 3091–3097.10.2174/138527211798357074
- United States Environmental Protection Agency: http://www.epa.gov/hpv/pubs/summaries/ptolacid/c16597.pdf (accessed on 12 December 2015).
- M. R. Mohammadizadeh, A. Hasaninejad and M. Bahramzadeh: ‘Trifluoroacetic Acid as an Efficient Catalyst for One-Pot, Four-Component Synthesis of 1,2,4,5-Tetrasubstituted Imidazoles Under Microwave-Assisted, Solvent-Free Conditions’, Synth. Commun., 2009, 39, 3232–3242.10.1080/00397910902737122
- S. Fletcher and P. T. Gunning: ‘Mild, efficient and rapid O-debenzylation of ortho-substituted phenols with trifluoroacetic acid’, Tetrahedron Lett., 2008, 49, 4817–4819.10.1016/j.tetlet.2008.06.022
- Y. C. Wu, L. Liu, Y. L. Liu, D. Wang and Y. J. Chen: ‘TFA-mediated Tandem Friedel−Crafts alkylation/cyclization/hydrogen transfer process for the synthesis of flavylium compounds’, J. Org. Chem., 2007, 72, 9383–9386.10.1021/jo7015604
- A. G. Berends, J. C. Boutonnet, C. G. de Rooij and R. S. Thompson: ‘Toxicity of trifluoroacetate to aquatic microorganisms’, Environ. Toxicol. Chem., 1999, 18, 1053–1059.10.1002/etc.v18:5
- M. Braun and U. Claassen, Solvay Fluor Und Derivate Gmbh: ‘Method of synthesizing alkenone compounds’, US Patent 7057079 B2, 6 June 2006.
- B. R. Yasani, E. Martinelli, G. Galli, A. Glisenti, S. Mieszkin, M. E. Callow and J. A. Callow: ‘A comparison between different fouling-release elastomer coatings containing surface-active polymers’. Biofouling, 2014, 30, 387–399.10.1080/08927014.2013.878864
- M. E. Callow, J. A. Callow, J. D. Pickett-Heaps and R. Wetherbee: ‘Primary adhesion of Enteromorpha (Chlorophyta, Ulvales) propagules: quantitative settlement studies and video microscopy’. J. Phycol., 1997, 33, 938–947.10.1111/j.0022-3646.1997.00938.x
- G. Momen and M. Farzaneh: ‘Survey of micro/nano filler use to improve silicone rubber for outdoor insulators’, Rev. Adv. Mater. Sci., 2011, 27, 1–13.
- D. J. Coady, K. Fukushima, H. W. Horn, J. E. Rice and J. L. Hedrick: ‘Catalytic insights into acid/base conjugates: highly selective bifunctional catalysts for the ring-opening polymerization of lactide’, Chem. Commun., 2011, 47, 3105–3107.10.1039/c0cc03987j
- A. Beigbeder, M. Linares, M. Devalckenaere, P. Degee, M. Claes, D. Beljonne, R. Lazzaroni and P. Dubois: ‘CH-π interactions as the driving force for silicone-based nanocomposites with exceptional rheological and thermal properties’, Adv. Mater., 2008, 20, 1003–1007.10.1002/(ISSN)1521-4095
- L. P. Zhao and L. Gao: ‘Novel in situ synthesis of MWNTs-hydroxyapatite composites’, Carbon, 2004, 42, 1858–1861.10.1016/j.carbon.2004.02.013
- M. Morra, E. Occhiello, R. Marola, F. Garbassi, P. Humphrey and D. Johnson: ‘On the aging of oxygen plasma-treated polydimethylsiloxane surfaces’, J. Colloid Interface Sci., 1990, 137, 11–24.10.1016/0021-9797(90)90038-P