Abstract
Natural rubber latex (NRL) is mainly used around traditional industrial products, but currently their target application is continuously expanding into tissue engineering. To broaden our knowledge of application in tissue engineering of NRL, we have focused on the surface modification of NRL nanoparticles through the biomineralization of hydroxyapatite (HA) using simulated body fluid in order to create a better cytocompatibility with controlled cell adhesion and mineralization properties in osteogenic culture to determine the effect on bone outcomes. Using MC3T3-E1 mouse osteoblastic-like cells incubated with NRL nanoparticles coated with HA layer, we have examined the osteogenic differentiation and expressions of multiple proteins and characteristic genes of mature osteoblast. We have successfully prepared the biocomposites composed of NRL and bone tissue.

Introduction
Natural rubber latex (NRL) is a type of high molecular weight polymeric substance. Currently, more than 90% of NRL comes from one single tropical tree species – Hevea brasiliensis (para rubber tree). NRL is mainly used around traditional industrial products, such as gloves, condoms, balloons, medical equipment, and tires, but currently their target application is continuously expanding into tissue engineering. Recently, promising results in the use of NRL from Hevea brasiliensis rubber tree to produce replacement and regeneration tissues have been reported.Citation1–3 Among them, wound healing in cutaneous tissues, eardrum replacement, bone regeneration, and dental alveolus replacement afford a unique means to repair or replace the failing organs or tissues.Citation2 The primary particle diameter of NRL is in the range of 100–300 nm, which is promising for nanoparticles used in biotechnology including pharmaceutics, tissue engineering, and regenerative medicine. The nanoparticles are usually taken up through endocytosis. The particle size between 20 and 200 nm is suitable for drug delivery applications based on in vivo studies.Citation4
In our preliminary study, we have demonstrated the latex cytocompatibility in vitro against cultured human lung carcinoma (A549) and mouse calvaria preosteoblast (MC3T3-E1) cells. The NRL nanoparticles enhance MC3T3-E1 cell proliferation and viability at concentrations of 10.0 μg/mL in comparison with that of the control. In contrast, the NRL particles are found to be toxic to the A549 cells (cell viabilities are below 60% at concentration of 100 μg/mL). The administration of NRL particles could effectively inhibit the proliferation of A549 cells within 1 day. The estimated values of half maximal inhibitory concentration (IC50) are 330 ± 20 μg/mL for A549 cells and 3.97 ± 0.10 mg/mL for MC3T3-E1 cells in the range of 10 μg/mL–10 mg/mL (Figure S1, Supplementary information). Our data showed that the addition of even as much as 1.0 mg/mL of NRL nanoparticles in cell culture did not kill tested MC3T3-E1 cells. The NRL nanoparticles exposure to biological tissue is expected when one applies NRL for anticancer drug delivery.
In order for NRL nanoparticles to be employed in regenerative medicine, they need to have designed biological interaction with cells as well as favorable mechanical properties.Citation5 Cells can delicately sense and respond to external nanoscale features in intricate living systems. With this in mind, an in-depth understanding of cell differentiation may bring a new perspective to regenerative medicine including new bone regeneration and cartilage therapy. To the best of our knowledge, no attention has been paid to differentiation activity of NRL nanoparticles.
In this study, we focused on the surface modification of NRL nanoparticles through the biomineralization of hydroxyapatite (HA) in order to create a better cytocompatibility with controlled cell adhesion and mineralization properties in osteogenic culture to determine the effect of bone outcomes. Using MC3T3-E1 osteoblastic-like cells, we examined the osteogenic differentiation and expressions of multiple proteins and characteristic genes of mature osteoblast.
Materials and methods
NRL and biomineralization
The NRL solution sample (solid content of 35.4 wt.%) was provided by Sumitomo Riko Co. Ltd., Japan and was not further purified for use. It was stabilized (pH 11.3) using an ammonia solution (0.5 wt.% in 100 mL of latex). We fabricated NRL nanoparticles covered with HA by using simulated body fluid (SBF) having ion concentrations nearly equal to those of human blood plasma.Citation6
Milli-Q ultrapure water (18 MΩcm, total organic carbon (TOC) < 20 ppb) was added into plastic beaker under magnetic stirring. The water in the beaker was heated up to 36.5 °C. All reagents for mineralization were purchased from Nacalai Tesque, Kyoto. The mineralization solution, designated as SBF, (NaCl, 136.8 mM; NaHCO3, 4.2 mM; KCl, 3.0 mM; K2HPO4·3H2O, 1.0 mM; MgCl2·6H2O, 1.5 mM; CaCl2, 2.5 mM; Na2SO4, 0.5 mM; Tris base, 50 mM) was adjusted to the pH 7.40 precisely using 1.0 M of HCl.
4.0 mL of NRL solution (0.01 wt.%) and 36 mL of SBF were mixed at ambient temperature. The solution was incubated at 36.5 °C. After 24 h, a white precipitate (designated as NRL-HA) was yielded in the solution. The precipitate was collected after centrifuge at a speed of 5000 rpm for 5 min and subjected to the dialysis (MWCO 12000–14000, Fisher scientific) against deionized water for 24 h to remove free ions in dispersion. Subsequently, freeze-drying (FDU-2200, Eyela Ltd.) was performed under 20 Pa at –80 °C.
Characterization
The surface charge characteristics of NRL and NRL-HA nanoparticles in water (0.01 wt.%) were determined by electrophoresis (Zetasizer Nano ZS, Malvern Instruments, UK) by the technique of laser Doppler anemometry adjusting the pH of the suspension in the range of 2–12 using dilute HCl and NaOH (Nacalai-Tesque). The average diameter of the nanoparticles was measured by dynamic light scattering (DLS) using Zetasizer Nano ZS (wavelength = 532 nm). The dynamic information can be retrieved by examining the autocorrelation function g(t) of the time-dependent intensity.Citation7
The morphology was observed through field emission scanning electron microscope (FE-SEM: SU6600, Hitachi Ltd.). The operated accelerating voltage was 15 kV and the specimens were coated with a thin layer of gold and palladium (Au/Pd 6:4) with a thickness of ~20 nm on copper grids with 200 mesh size.
Fourier transform infrared (FTIR) spectroscopic imaging measurements were performed using a Perkin-Elmer Spectrum Spotlight 400 Microscope System. This system is equipped with a liquid N2 cooled Mercury-Cadmium-Telluride MCT detector. To construct FTIR maps, spectra were collected in continuous scan mode for sample area of 200 × 200 μm2 with a resolution of 1.65 μm/pixel by one scan for each spectrum of the specimens under the Ge attenuated total reflectance (ATR) crystal. Spectra were collected from 4000 to 680 cm−1 with a resolution of 8 cm−1 and integrated by taking the areas under the curve between the limits of the peaks of interest. Data acquisition was carried out by means of the Spotlight software package.
The thermal behavior was determined by thermo-gravimetric/differential thermal analysis (TG8120, Rigaku Inc.) in the temperature range of 20–900 °C at a heating rate of 5 °C/min under dry air. Sample weight of NRL-HA was normalized at 2.8 mg. The NRL nanoparticles before biomineralization was used for a control.
In vitro cell culture and cell viability
MC3T3-E1 mouse calvaria preosteoblastic cells obtained from ATCC were cultured in Alpha Minimum Essential Medium (α-MEM) (Gibco®, Life Technologies) at pH 7.4 supplemented with 10% (v/v) fetal bovine serum (FBS) (Gibco®, Life Technologies) and 1% antibiotic-antimycotic mixture (Nacalai-Tesque). Upon reaching confluence, cells were cultured on 10 cm dishes in an atmosphere of 5% CO2 and 95% relative humidity at 37 °C. MC3T3-E1 cells at 2–4 passages were used in the experiment.
Human lung carcinoma A549 cells (ATCC) was used as a reference cell and cultured in RPMI-1640 (Wako Pure Chemical Industries) supplemented with 10% FBS including 1% antibiotic-antimycotic mixture. A549 cells at 2–5 passages were used in the experiment.
The cells were seeded in 96-well plates at a cell concentration of 2 × 104 cells/cm2 in 100 μL of medium and cultured for 24 h. The NRL-HA and/or NRL suspensions at different concentrations (from 12 μg/mL to 1.2 mg/mL) were diluted with complete culture medium and added to each well. After incubation for 24 h in an atmosphere of 5% CO2 and 95% relative humidity at 37 °C, the cell viability was assessed by WST-8 assay (Dojindo) according to the manufacturer’s instructions. The WST-8 colorimetric test is measuring the activity of intracellular dehydrogenase activity, which is proportional to living cells. The optical density was read using a Multiskan FC (Thermo Fisher Scientific) at 450 nm for the absorbance and at 650 nm for the subtract background absorbance, respectively.
Differentiation
For osteogenesis, MC3T3-E1were seeded at a density of 4 × 104 cells/cm2 in 200 μL of osteogenic medium and cultured with NRL-HA and/or NRL nanoparticles, as reference. The effect of NRL-HA on cell differentiation was determined for 20 days in osteogenic medium, consisting of α-MEM, 10% FBS, 1% (v/v) ascorbic acid (TaKaRa), 0.2% (v/v) Hydrocortisone (TaKaRa), 2% (v/v) β-Glycerophosphate (TaKaRa), and 1% antibiotic-antimycotic mixture. The medium was changed with a fresh one every 3 days, 6 times during the 20 days incubation.
For the calcium deposition, after 20 days, the osteoinduction of the MC3T3-E1 cells in osteogenic culture was analyzed by measuring the absorption of treated cells by Alizaline Red staining (PG Research) according to the manufacture’s instructions. The optical density at 450 and 650 nm, for the subtract background absorbance, were read using a microplate spectrophotometer (Multiskan FC, Thermo Fisher Scientific).
Gene expression
The total RNA was extracted from cells, induced to osteogenic differentiation for 3, 10 and 20 days, using RNeasy Mini Kit (Qiagen) with a residual genomic DNA Eraser following the protocol. Then the RNA was subjected to reverse transcription using a SuperScript® III (Invitrogen, Life Technologies) following the manufacture’s instructions. The resulting complementary DNA (cDNA) yield was then subjected to real-time polymerase chain reaction (RT-PCR) using an ABI PRISM 7000 Sequence Detention System (Applied Biosystems). The results were analyzed with Sequence Detector software (Applied Biosystems). Reaction solutions included SYBR Green Mastermix, 1 μM forward and reverse predesigned primers (Table ), and 10 μL cDNA template in a 30 μL volume. The cDNA samples were analyzed for expression of Runx2, Sp7/Osterix, alkaline phosphatase (Alp), and osteocalcin (Bglap), relative to the β-actin (ACTB) as an internal standard for sample normalization.
Table 1 Validated primer sequences for RT-PCR
Statistics
Statistical analysis was performed using Student’s t-test and one-way analysis of variance with Dunnett's post hoc testing, and significance was considered at a probability of p < 0.05.
Results and discussion
Characterization of NRL-HA nanoparticles
The main reason for the formation of NRL-HA nanoparticles is due to the electrostatic interaction between negatively charged layer along the NRL surface and Ca2+, which deposited calcium ions, in turn, interacted with phosphate ions (PO43–) in the SBF. The HA then grew spontaneously accompanied by consuming the calcium and phosphate ions to form apatite.Citation8,9 The presence of layers can be demonstrated by monitoring the zeta potential, which change from negative toward positive direction when HA is at the outside and NRL (~ –70 mV) is at the inside as shown in Figure (a). The zeta potentials of the NRL-HA nanoparticles at pH 7.4 exhibit the negative values (–40 mV), indicating somewhat dense HA layers including cations, (e.g. Ca2+ and CaOH+) on the NRL particle surfaces. The values increase continuously with decreasing pH to attain 0 mV at pH 2.0.
Figure 1 (a) Zeta potential vs. pH of NRL and NRL-HA nanoparticles in water (0.01 wt.%). For comparison, the precipitated HA without NRL particles is shown (0.01 wt.%). Results are expressed as mean ± S.D. (Standard deviation) (n = 5). (b) Particle size distribution with PDI of NRL and NRL-HA after biomineralization at concentration of 0.01 wt.% at pH 7.4
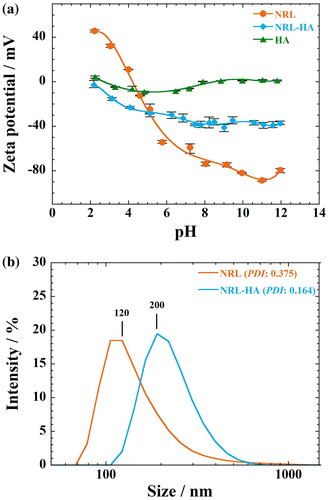
For comparison, the zeta potential of precipitated HA without NRL particles prepared at high pH 10.0, is also presented. The zeta potential values remain constant 0 to –10 mV, indicating both positively and negatively charged species are present on the surface of the HA particles.Citation10 At dispersion into water, the HA particles might have various ions on their surfaces (e.g. Ca2+, CaOH+, PO43–, HPO42–, H2PO4–, and CaH2PO4+). The positive surface charge is attributed to the increasing adsorption of H+ or the loss of OH–,Citation11 and vice versa in case of the negative surface charge. In over the entire pH range from 2 to 12, the HA particles are stabilized electrostatically due to the high surface charge.Citation12
The particle size of NRL-HA nanoparticles from DLS measurement is presented in Figure (b). At pH 7.4, the average particle size of pristine NRL is around 120 nm with polydispersity index (PDI) of 0.375 at concentration of 0.01 wt.%. The average size of the NRL-HA nanoparticles is 200 nm having narrower PDI of 0.164 in comparison with that of NRL nanoparticles before coating with HA layer.
Figure shows the morphological feature of NRL-HA nanoparticle as revealed by FE-SEM image. The nanoparticle exhibits rather coarse surface having a size of ~1 μm (Figure (a)). In addition, small discrete particle after biomineralization is also observed in which the particle size is ~200 nm (Figure (b)). We can postulate that the negatively charged layer on the NRL surface act as nucleating sites and HA crystal growth front as well. However, the top surface covered with HA layer may show less-ordered crystal structure (low crystallinity) as reported by our previous study of DNA mineralization in SBF.Citation8
Figure 2 FE-SEM images showing NRL-HA nanoparticle at concentration of 10−4 wt.%: (a) discrete particle and (b) small discrete particle with coarse surface
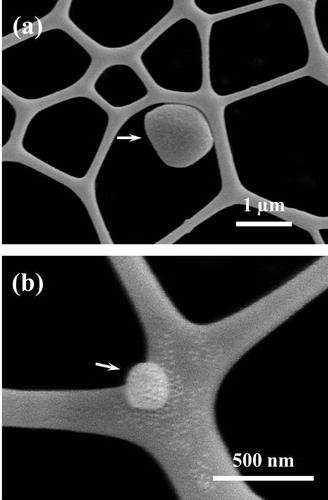
The TGA curve, derivative analysis (DTG) and DTA of the NRL-HA nanoparticles and the pristine NRL are shown in Figure . The NRL nanoparticles lost ~3% mass in the temperature range 120–200 °C (Figure (a)). A significant mass loss (~93%) from 200 to 510 °C is observed, accompanied by the four corresponding exothermic peaks at 240, 301, 404, and 453 °C, which are due to the pyrolysis and/or decomposition of NRL (Figure (b)).
Figure 3 TGA curves, derivative analysis (DTG) and DTA of (a), (b) pristine NRL nanoparticles, and (c), (d) NRL-HA nanoparticles
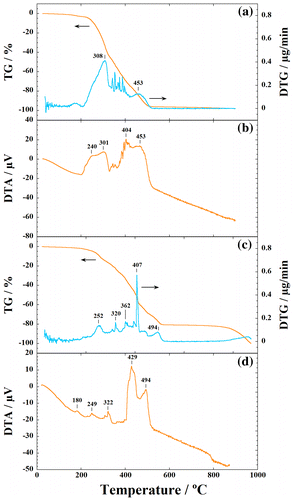
In contrast, NRL-HA nanoparticles indicate the weight loss of ~80% after freeze-drying in the temperature range from 200 to 510 °C (Figure (c)). The NRL in the NRL-HA decomposes in two stages, with a weight loss of 45% between 200 and 400 °C and a weight loss of 35% from 400 up to 510 °C, accompanied with the several peaks in DTG (252, 320, 362, 407, and 494 °C). At the same time, we can find that the two exothermic peak temperatures (i.e. 429 and 494 °C) of the NRL degradation in the NRL-HA nanoparticles are shifted to a higher temperature as compared with that of the pristine NRL (i.e. 404 and 453 °C) (cf. DTA curves: Figure (b), (d)).
The excellent thermal stability of HA beyond the temperature 1000 °C is well known in the literature.Citation13 The thermal stability of the incorporated NRL in NRL-HA nanoparticles is much better than that of the pristine NRL, despite the low crystallinity of HA. The calculated weight ratio of NRL/HA in the NRL-HA nanoparticles is ca.84/16 by taking the combustion residue of the pristine NRL into account.
FTIR image is generated by plotting the principal components analysis (PCA) to illustrate the relative distribution of the components (Figure ). The NRL-HA nanoparticles are divided into five components as revealed by PCA. In all components, original peaks of both NRL and HA are assigned. The sensitive bands at 2944 (asymmetric stretching: νas(CH2)), 2852 (symmetric stretching: νs(CH2)), 836 cm−1 (cis[CH=CH]) are attributed to cis-1,4-polyisoprene. The sensitive bands at 1628 (peptide: ν(C=O)), 1552, 1238 (peptide: ν(C=O/N–H)), and 1296 cm−1 (asymmetric stretching mode: νas(PO2–)) are attributed to the surface protein of NRL. The sensitive band at 1036 cm−1 (ν3(PO43–)) is attributed to HA.Citation14 The bands at 1460 cm−1 (ν3(CO32–)) and 1388 cm−1 (ν3(CO32–)) are presented.Citation15,16 That is, the phosphate groups or hydroxyl groups in HA crystallites have replaced the carbonate acid in SBF accompanied with the formation of carbonate hydroxyapatite, which indicate low crystallinity by incorporation of carbonate acid into HA via an anion exchange reaction. A band located at around 3200 cm−1 was assigned to the structural OH stretching mode (ν(OH)) from both NRL and HA. In the panels (2) and (5) in Figure , HA-rich compositions are detected in NRL particles.
Figure 4 FTIR image of NRL-HA nanoparticles after biomineralization obtained from the selected 200 × 200 μm2 area (left panel). FTIR spectra of corresponding five constituents (a)–(e) by plotting PCA (right panel) in the region of 4000–680 cm−1
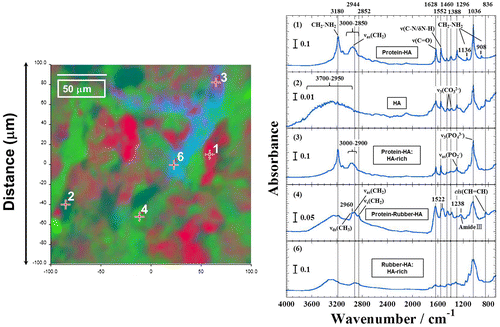
Although the information of them should be considered in more detail, the NRL-HA nanoparticles with different compositions are successfully prepared in the SBF. The constituents of the NRL-HA nanoparticles are mixture at present as seen in PCA. However, the cytocompatibility analysis has not been reported. The cytotoxicity of the NRL-HA nanoparticles were measured against MC3T3-E1 and cancer cells.
Cytotoxicity of NRL-HA nanoparticles
The viability of MC3T3-E1 cells is very sensitive to the NRL nanoparticle concentration. Beyond concentration of 10.0 μg/mL, NRL nanoparticles enhance MC3T3-E1 cells and viability in the range of 130–150% in comparison with that of control (Figure (a)). On the other hand, MC3T3-E1 cells exhibit no toxic effects and are not sensitive to NRL-HA nanoparticles, accompanied with high metabolic and dehydrogenase activity of the cells at concentration up to 1.2 mg/mL (Figure (a)).
Figure 5 Cell viability as measured by WST-8 assay using (a) MC3T3-E1 and (b) A549 cells after 24 h of incubation with NRL and/or NRL-HA nanoparticles of different concentrations. Data were expressed as mean ± S.D. (n = 5)
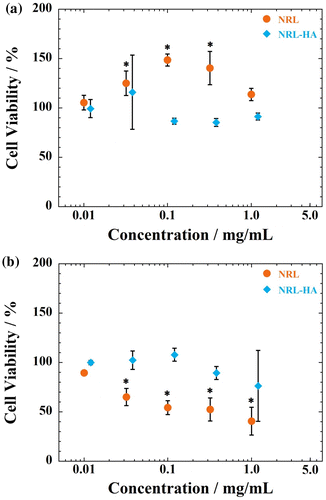
As seen in Figure S1 (Supplementary information), the administration of NRL particles could effectively inhibit the proliferation of A549 cells within 1 day. The estimated IC50 values are 330 ± 20 μg/mL for A549 cells in the range of 10 μg/mL to 10 mg/mL. In contrast, the NRL-HA nanoparticles are found to be no toxic to the A549 cells (cell viabilities remain constant up to 1.2 mg/mL) (Figure (b)). For HA layer on the surface of NRL particle, it is possible to enhance the cell viability even at high concentration dose of up to 1.2 mg/mL. These results indicate that the HA coating on NRL nanoparticles causes significant changes in the cytotoxicity of both cells.
Gene expression and matrix mineralization of MC3T3-E1 cells
The gene expression of Runx2, Sp7/Osterix, alkaline phosphatase (Alp), and osteocalcin (Bglap) relative to housekeeping gene (ACTB) were determined by RT-PCR at day 3, day 10, and day 20. Both NRL and NRL-HA nanoparticles show relatively stable expression of Runx2 from 3 days to 20 days (Figure (a)). The MC3T3-E1cells in mineralized matrices exhibit higher expression of Runx2 for the late stage of osteogenic differentiation (at day 20). But there are no statistical difference between any of samples and control throughout 20 days of culture. The Sp7/Osterix is also considered as a marker for the early stage osteogenic differentiation.Citation17 The expression of Sp7/Osterix shows same trend with Runx2 (Figure (b)). The expression of Alp gene is elevated up to day 20. Since Alp is generally considered as an early marker of osteoblast phenotype and calcified matrix production.Citation17 The NRL-HA nanoparticles promote the Alp gene expression than control at day 20 (Figure (c)), indicating the up-regulation leads to matrix maturation.
Figure 6 RT-PCR analysis of the osteogenic gene expression of (a) Runx2, (b) Sp7/Osterix, (c) Alp, and (d) Bglap for MC3T3-E1 cells cultured with NRL nanoparticles of 1.0 mg/mL and/or NRL-HA nanoparticles of 1.0 mg/mL at different time intervals (3, 10, and 20 days). Data were expressed as mean ± S.D. (n = 3)
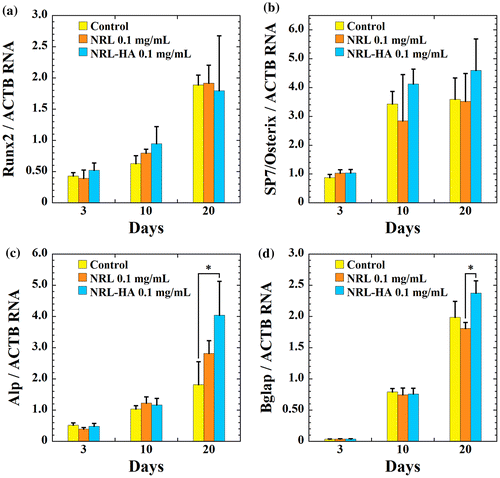
This promotion effect is further confirmed by the expression of the Bglap gene, which is a typical marker for the late stage of osteogenic differentiation. The cells cultured with NRL-HA nanoparticles exhibit significantly higher expression of Bglap in 10 days in comparison with that of NRL nanoparticles (Figure (d)).
Interestingly, for osteogenic differentiation of MC3T3-E1 cells, there are no statistical difference in osteogenic differentiation between control and loading of NRL nanoparticles of 0.1 mg/mL throughout 20 days of culture, as shown in Figure .
Figure 7 (a) Alizaline staining and (b) qualitative evaluation of calcium deposition by MC3T3-E1 cells cultured with different NRL nanoparticle concentrations (0.10, 0.32, and 1.0 mg/mL) after 20 days. (c) Alizaline staining and (d) qualitative evaluation of calcium deposition by MC3T3-E1 cells cultured with different NRL-HA nanoparticle concentrations (0.10, 0.38, and 1.2 mg/mL) after 20 days. Data were expressed as mean ± S.D. (n = 4)
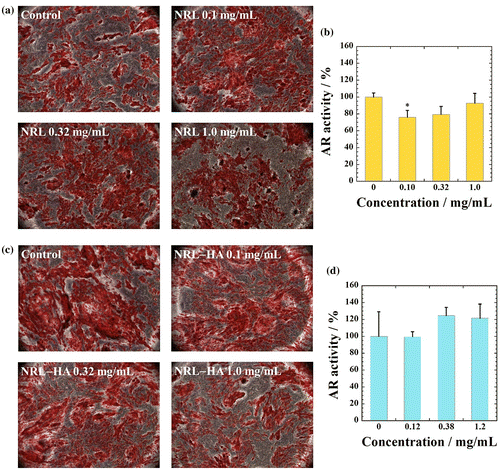
To examine matrix mineralization, 20 days cultures were fixed and stain by Alizaline solution, which stains calcium deposits as another marker of osteogenic differentiation. Interestingly, for osteogenic differentiation of MC3T3-E1 cells, there are no statistical difference in absorbance between any of samples except for NRL particles with 0.1 mg/mL, in which a slightly lower than the control, as shown in Figure . These results indicate that the HA coating on NRL nanoparticles did not cause significant changes in osteogenic differentiation in different concentrations of NRL-HA nanoparticles in the range of 120 μg/mL to 1.2 mg/mL. In addition, we have successfully prepared the biocomposites composed of NRL and bone tissue. Taking together the calcium deposition and gene expression, the results show promise of the NRL nanoparticle for application in bone engineering.
Conclusions
We have demonstrated the surface modification of NRL nanoparticles through the biomineralization of HA layer using SBF at 36.5 °C. The formation of NRL-HA nanoparticles were characterized by monitoring the zeta potential with different pH environment, morphological observation, DLS measurement, and FTIR imaging technique. The HA coating on NRL nanoparticles caused significant changes in the cytotoxicity of both MC3T3-E1 and A549 cells. The NRL-HA nanoparticles promoted the Alp gene expression than control at day 20. After 20 days of incubation with osteogenic supplement, both rather stable gene expression and calcium deposition were observed from MC3T3-E1 cells cultured with both NRL and NRL-HA nanoparticles. We have successfully prepared the biocomposites composed of NRL-HA and bone tissue. The results showed promise of the NRL nanoparticles for application in bone tissue engineering. Further efforts are needed to explore the mechanical properties of the biocomposites and long-term toxicity in pre-clinical setting.
Author contributions
The manuscript was written through contributions of all authors. All authors have given approval to the final version of the manuscript.
Disclosure statement
No potential conflict of interest was reported by the authors.
Funding
This work was supported by the Grant in TTI as a Special Research Project (2016).
Supplementary data
Supplementary material related to this article can be found, in the online version, at https://doi.org/10.1080/20550324.2017.1352111.
YNAN_1352111_Supplementary_Material.docx
Download MS Word (323.2 KB)References
- C. Ereno, S. A. Catanzaro Guimaraes, S. Pasetto, R. D. Herculano, C. P. Silva, C. F. O. Graeff, O. Tavano, O. Baffa and A. Kinoshita: ‘Latex use as an occlusive membrane for guided bone regeneration’, J. Biomed. Mater. Res. A., 2010, 95A, 932–939, DOI: 10.1002/jbm.a.32919.
- R. D. Herculano, C. P. Silva, C. Ereno, S. A. Catanzaro Guimaraes, A. Kinoshita and C.F. de Oliveira Graeff: ‘Natural rubber latex used as drug delivery system in guided bone regeneration (GBR)’, Mater. Res., 2009, 12, (2), 253–256, DOI: 10.1590/S1516-14392009000200023.
- R.B. Sampaio, R.J. Mendonca, A.R. Simioni, R.A. Costa, R.C. Siqueira, V.M. Correa, A.C. Tedesco, A. Haddad, J. Coutinho Netto and R. Jorge: ‘Rabbit retinal neovascularization induced by latex angiogenic-derived fraction: an experimental model’, Curr. Eye Res., 2010, 35, (1), 56–62, DOI: 10.3109/02713680903374216.
- C. E. Pedraza, D. C. Bassett, M. D. McKee, V. Nelea, U. Gbureck and J. E. Barralet: ‘The importance of particle size and DNA condensation salt for calcium phosphate nanoparticle transfection’, Biomaterials, 2008, 29, 3384–3392, DOI: 10.1016/j.biomaterials.2008.04.043.
- T.R. Cox and J.T. Erler: ‘Remodeling and homeostasis of the extracellular matrix: implications for fibrotic diseases and cancer’, Dis. Models Mechan., 2011, 4, 165–178, DOI: 10.1242/dmm.004077.
- T. Kokubo and H. Takadama: ‘How useful is SBF in predicting in vivo bone bioactivity?’, Biomaterials, 2006, 27, 2907–2915, DOI: 10.1016/j.biomaterials.2006.01.017.
- Y. Nishida, R. Domura, R. Sakai, M. Okamoto, S. Arakawa, R. Ishiki, M. R. Salick and L. Turng: ‘Fabrication of PLLA/HA Composite Scaffolds modified by DNA’, Polymer, 2015, 56, 73–81, DOI: 10.1016/j.polymer.2014.09.063.
- T. Takeshita, Y. Matsuura, S. Arakawa and M. Okamoto: ‘Biomineralization of hydroxyapatite on DNA Molecules in SBF: morphological features and computer simulation’, Langmuir, 2013, 29, 11975–11981, DOI: 10.1021/la402589j.
- Q. Zhang, V. N. Mochalin, I. Neitzel, K. Hazeli, J. Niu, A. Kontsos, J. G. Zhou, P. I. Lelkes and Y. Gogotsi: ‘Mechanical properties and biomineralization of multifunctional nanodiamond-PLLA composites for bone tissue engineering’, Biomaterials, 2012, 33, 5067–5075, DOI: 10.1016/j.biomaterials.2012.03.063.
- V. Sokolova and M. Epple: ‘Inorganic nanoparticles as carriers of nucleic acids into cells’, Angew. Chem. Int. Ed., 2008, 47, 1382–1395, DOI: 10.1002/anie.200703039.
- K. Skartsila and N. Spanos: ‘Surface characterization of hydroxyapatite: Potentimetric titrations coupled with solubility measurements’, J. Colloid Interface Sci., 2007, 308, 405–412, DOI: 10.1016/j.jcis.2006.12.049.
- ‘Zeta potential of colloids in water and waste water’, Philadelphia, ASTM D4187–82, 1985.
- D. M. Liu, T. Troczynski and W. J. Tseng: ‘Water-based sol-gel synthesis of hydroxyapatite: process development’. Biomaterials, 2001, 22, 1721–1730, DOI: 10.1016/S0142-9612(00)00332-X.
- W. L. Murphy, D. H. Kohn and D. J. Mooney: ‘Growth of continuous bonelike mineral within porous poly(lactide-co-glycolide) scaffolds in vitro’, J. Biomed. Mater. Res., 2000, 50, 50–58, DOI: 10.1002/(SICI)1097-4636(200004)50:1<50::AID-JBM8>3.0.CO;2-F.
- M. C. Chang and J. Tanaka: ‘FT-IR study for hydroxyapatite/collagen nanocomposite cross-linked by glutaraldehyde’, Biomaterials, 2002, 23, 4811–4818, DOI: 10.1016/S0142-9612(02)00232-6.
- G. Xu, I. A. Aksay and J. T. Groves: ‘Continuous crystalline carbonate apatite thin films’, A Biomimetic approach JACS, 2001, 123, 2196–2203, DOI: 10.1021/ja002537i.
- J. Y. Choi, B. H. Lee, K. B. Song, R. W. Park, I. S. Kim, K. Y. Sohn, J. S. Jo and H. M. Ryoo: ‘Expression patterns of bone-related proteins during osteoblastic differentiation in MC3T3-E1 cells’, J. Cell Biochem., 1996, 61, (4), 609–618, DOI: 10.1002/(SICI)1097-4644(19960616)61:4<609::AID-JCB15>3.0.CO;2-A.