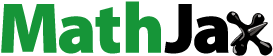
Abstract
Antibacterial foam dressing has been developed in recent years based on polymeric membranes and silver ions or silver nanoparticles as antibacterial components. However, silver ions or silver nanoparticles have cytotoxicity to humans. Herein, we used biocompatible multi-branched gold nanoparticles as an antimicrobial agent in polyurethane foam wound dressings. This study described a rapid and surfactant-free method for the preparation of multi-branched gold nanoparticles using hydroquinone as a reducing agent and chitosan as a stabilizer as well as assisted irradiation. The optimal procedure of polyurethane foam fabricated with high water absorption and small average pore size, achieved nearly 500% of absorptivity and 98 nm of pore size. The antibacterial effects of the multi-branched gold nanoparticles containing polyurethane foam against both Escherichia coli and Staphylococcus aureus were investigated. The results demonstrated that multi-branched gold nanoparticles can be used as a viable alternative to conventional antibiotics in wound dressings.
Graphical Abstract
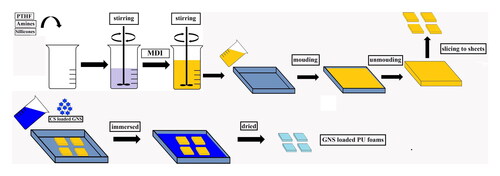
1. Introduction
In recent times, anisotropic gold nanoparticles have gained a great interest from the scientific community, envisaging their use new application areas including optical sensing, biomedicine, and chemical catalysis [Citation1–4]. Many research groups have developed the synthesis of anisotropic gold nanoparticles via two synthetic routes: seed [Citation5–8] and seedless [Citation9, Citation10] based methods. It is thought that both methods involve the reduction of Au3+ ions. In seed-based methods, small spherical gold nanoparticles (AuNPs) are used as seeds [Citation9]. These seeds serve as nucleation centres for the reduction of Au3+ promoting anisotropic growth. In contrast, seedless-based methods involve a growth mechanism controlled by reaction kinetics and key chemical factors (e.g. stabilizing, reducing, and capping agents) [Citation11]. In this report, a modification of the seedless–based method is introduced to synthesize multi-branched nanoparticles (GNS), three-dimensional assembling of anisotropic gold nanoparticles. Ultrasound assisted irradiation, is considered as an effective solution for the green synthesis of anisotropic gold nanoparticles due to several advantages including improvement of reaction conditions and selectivity, low pollution, and safe operation [Citation12].
Wound healing is a complex process and the mechanism of tissue repair processes includes many complex steps: inflammation, proliferation, and maturation [Citation13]. Wounds can be classified into two main groups, acute or chronic [Citation14]. Therein, chronic wounds do not heal as the normal processes. Non-healing wounds usually appear after some type of contamination has occurred. Therefore, it is necessary to develop wound dressings excluding contaminations and preventing growth of bacterial infections [Citation15]. There were many types of material developed for wound dressing including gauze, calcium/sodium alginate, hydrocolloids, hydrogel and silicone/polyurethane foam [Citation16–22]. Among them, polyurethane (PU) foam for wound dressing has many advantages compare with others materials, especially for moderate wound and drainage [Citation23]. Due to their good mechanical and physical properties and biocompatibility [Citation24–26], PU is used in a variety of biomedical applications. These include surgical tubing and drapes, catheters, wound dressing, and heart valve [Citation27–30]. Choosing a suitable dressing could creates optimal environment to accelerate wound healing and prevent the wound infection [Citation31]. Wound dressings based on PU have great water absorption capability and create a humid environment due to their opened-cell structure. These enhance wound healing and protect the wound from infection [Citation32]. Moreover, their physical properties such as thickness, mechanical stability, water absorption play a positive role in wound management [Citation33]. Besides, PU can be combined with various fillers to produce antibacterial PU composite foam. Among many types of filler, noble metallic nanoparticles such as gold nanoparticles (AuNPs) and silver nanoparticles (AgNPs) are attracted because of their great antimicrobial activity [Citation34, Citation35]. Especially, anisotropic gold nanoparticles have been used as antibacterial material against a broad range of bacteria in recent years [Citation36]. To the best of our knowledge, there have been only limited on the antibacterial activities of gold nanoparticles loaded polyurethane (PU) foam [Citation37]. Additionally, there has been no research on the antibacterial effect of GNS containing PU foam. Naik et al. reported [Citation38] the preparation of PU sheets embedded with methylene blue (MB), toluidine blue (TB), and AuNPs (2 nm) for the antibacterial activity. In this study the synthesis of GNS was conducted using ultrasound assisted irradiation seedless method. The antibacterial action of multi-branched gold nanoparticles containing polyurethane foam against Escherichia coli and Staphylococcus aureus was investigated.
2. Materials and methods
2.1. Materials
Chloroauric acid (HAuCl4.H2O, 52%), chitosan (CS) (deacetylated chitin, low molecular weight), hydroquinone (HQ, 99%) and acetic acid (glacial, 99%) were obtained from Sigma-Aldrich. Deionized water (18MΩ) was used throughout experiments. All chemical materials were GR grade. Polytetrahydrofuran (PTHF, average Mn 1,000), 4,4′-Methylenebis(phenyl isocyanate) (MDI), hexamethyldisiloxane (HDMSO, 98%) and poly(dimethylsiloxane) (PDMS), triethylenediamine (TEDA) were purchased of Sigma-Aldrich. Escherichia coli ATCC 25922 (E. coli) and Staphylococcus aureus ATCC 25923 (S. aureus) were obtained from ATCC. Muller Hinton Agar (MHA) and Luria-Bertani broth (LB) were purchased from Himedia.
2.2. Methods
2.2.1. Seedless, surfactant-free preparation of GNS combined ultrasound
The procedure for the preparation of GNS was carried according to the method reported previously [Citation39]. Firstly, a CS 2% solution was made by dissolving low molecular CS powder into 1% acid acetic solution. Next, 1 mL of HAuCl4 (2 × 10−2 M) solution was added to 9 mL CS 2% solution under stirring. Acetic acid was used to adjust the pH to 1.5. Finally, 1.0 ml of 0.1 M HQ solution was dropped into the mixture. The reaction mixture was then sonicated using Q2000 sonicator – Qsonica (power 1.375 watts, frequency 20 kHz. The color of solution changed from colorless to cobalt blue indicating multi-branched particles had formed. CS coated GNS were synthesized at a constant frequency of 20 kHz, level of amplitude 75 µm for 5 min at room temperature ().
The surface plasmon resonance (SPR) of GNS was carried by UV–Vis spectrophotometer Dynamica Halo RB-10 (Dynamica, Livingston, UK) in the wavelength range of 400–1100 nm, at a scanning rate of 200 nm/min. Transmission electron microscope (TEM) analysis was performed using a JEM1010-JEOL (Jeol, Tokyo, Japan). Thirty particles of each three sample from TEM photographs were used to calculate the average length of branches as well as diameter of cores of GNS using the J-Image software (NIH Image) [Citation40]. XRD pattern was recorded using Bruker diffractometer D5005.
2.2.2. Preparation of GNS loaded flexible PU foam
PU foam was produced by a one-step reaction of polyols with diisocyanate. All materials including PTHF 20 g, DI water, GNS, PDMS, HDMSO (parts per hundred polyol by weight) were stirred to form a homogenized blend. MDI was added into mixture and stirred for 15 s (). The mixture was poured into a mould for free foaming and curing at room temperature. Post PU foam as sliced into sheets with obtained length x width dimension of 50 × 50 mm as well as thickness of 6 ± 0.5 mm. Sliced PU sheets were immersed in GNS solution at ambient temperature for 24 h. Finally, PU sheets were evaporated at 40 °C to completely dried ().
Table 1. The compositions of multi-branched gold nanoparticles loaded flexible PU foam samples.
Moulded GNS loaded PU foam was sliced to sheets obtaining a size of 20 mm x 20 mm as well as thickness of 5 mm.
2.2.3. Characterization of water absorption and pore sizes
Water absorption was determined by taking the initial and final weights of foam sample after dipping sample in distilled water at room temperature for 24 h [Citation41]:
(1)
(1)
The microstructure of GNS loaded PU foam was observed using a Hitachi S-4800 scanning electron microscope (SEM) at accelerating voltage 3 kV. SEM images were used to calculate the average pore sizes of GNS loaded PU foam samples using the Image-J software.
2.2.4. Antibacterial test of GNS loaded flexible PU foam
The antibacterial activity of GNS loaded flexible PU foam was investigated using minimum inhibitory concentration (MIC) method [Citation33]. E. coli and S. aureus were grown in LB overnight at 37 °C to obtain a bacterial suspension with optical density OD600 = 0.105 − 0.115 (around 2.8 × 108 colony-forming unit, CFU/mL) for E. coli and OD600 = 0.100 − 0.110 (around 3.7 × 108 CFU/mL). Next, the bacterial cultures were diluted to 104 CFU for E. coli and 105 for S. aureus. Different concentrations of GNS loaded PU foams in size of 0.5 × 0.5 cm sterilized by UV light were soaked in previous bacterial suspensions at room temperature for 60 min. Different concentrations of GNS loaded PU foams were investigated in range of 5.0, 7.5, 10.0, 12.5 and 15.0 µg/mL.
After exposed with GNS loaded PU foams, bacterial suspensions were vortexed for 1 min. Next, 100 µL of exposed microbial suspension above was spread on MHA petri dish and incubated at 37 °C for 24 h. GNS unloaded PU foam was used as negative control. The antibacterial efficiency (E%) was calculated by formula below:
(2)
(2)
where Ncontrol is the total counted bacteria in control dish, Nsample is the total counted bacteria in GNS exposed dish
3. Results and discussion
3.1. Characterization of multi-branched gold nanoparticles
The UV–Vis spectroscopy and TEM analysis were shown in . The UV-Vis spectrum exhibited two absorption bands: a wide (surface plasmon resonance) SPR absorption ranged from 500- 1100 nm and reached the highest SPR absorbance at 839 nm. The weak band at the shorter wavelengths is the transverse plasmon resonance of core, whereas the longitudinal plasmon resonance of branches locates at the longer wavelengths [Citation42]. Besides, the synthesized GNS were multi-branched, prolonged particles with 45 ± 6 nm of branches while the average size of core, 40 ± 4 nm. Average length of branches as well as diameter of cores of GNS were calculated using the J-Image software (NIH Image), based on thirty particles of each three sample from the TEM images.
which was the recorded XRD pattern of GNS, exhibited four diffraction peaks corresponded to (111), (200), (220), and (311) planes of face-centered-cubic (fcc) structural crystal of gold [Citation43, Citation44]. It included an intense peak located at 37.2°, a moderate peak observed at 44.9°, another appeared at 65.5° and very weak at 78.2° indexed for (111), (220), (220), (311) planes, respectively.
3.2. Pore size and water absorption test
The morphology observed using SEM showed several round shaped and interconnected pores within the foam (). The average pore sizes of all GNS loaded PU samples are shown in . According to the result, all foamed samples have an average pore size ranging from 98–287 µm, depended on formulated compositions. Among them, GNS loaded PU flexible foam prepared by 10 php HDMSO and 0.5 php PDMS obtained the smallest pore size. Additionally, it was noticeable that the porosities of GNS loaded PU samples were similar to commercial wound dressings [Citation45, Citation46]. Besides, the composition of Au attached on PU foam was confirmed by EDX analysis ().
Figure 5. The SEM images of various GNS loaded flexible PU foams (a→e) and EDX spectrum of GNS loaded PU foam (4f): PU-1, b) PU-2, c) PU-3, d) PU-4, e) PU-5.
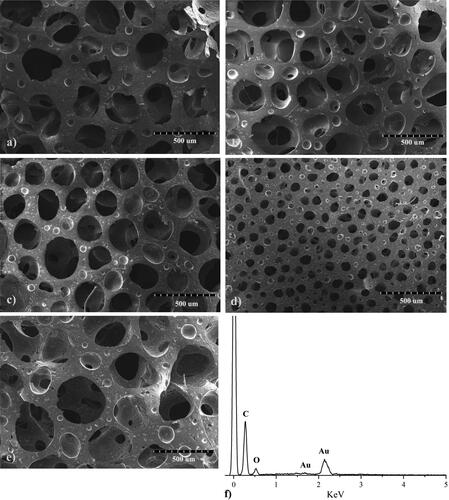
Table 2. The average pore sizes and water absorptions of multi-branched gold nanoparticles loaded flexible PU foam samples.
The calculated water absorption of GNS loaded PU foam samples are also shown in . According to results, the smallest pore size absorbs the highest volume of water. The GNS loaded PU foam which pore size was 98 µm, reached 497% of highest water absorption. While lowest water absorption was 102% related to GNS loaded PU foam had 287 µm of pore size. It was clear that the optimal PU foam for wound dressing was PU-4 sample because of high water absorption capacity and porosity. This sample was selected for bacterial testing.
3.3. Antibacterial effect of GNS loaded flexible PU foam
The antibacterial effect of various concentrations of GNS loaded PU foam samples against S. aureus and E. coli ranging from 0 µg/mL to 15.0 µg/mL are shown in . It is clear that control PU foam samples (0 µg/mL GNS) has no controlling affect, as expected. It indicated that pure PU had not effect on both E. coli and S. aureus. Furthermore, GNS loaded PU foam exhibited antibacterial acitivity against S. aureus better than E. coli at each similar concentration. The result showed that over 95% of S. aureus bacteria exposed to GNS loaded PU foam ranging from 5.0 − 15.0 µg/mL were destroyed. Meanwhile, antimicrobialactivity of GNS loaded PU foams against E. coli was approximately 85%, even at highest evaluated concentration of 15 µg/mL. This results could be explained by antibacterial mechanism according to some previous reports: gold nanoparticles can attach to the bacterial membrane and damage its integrity, leading to the leakage of bacterial components outside; or they pass into the cytoplasm where they can inhibit protein process, resulting in bacterial death [Citation47, Citation48]. Because of strict attachment to the surface of PU foam, GNS only destroys bacteria by interacting and disrupting the bacterial membrane. Beside peptidoglycan and plasma membrane, negative E. coli bacteria have an outer (lipopolysaccharide and protein) in cell wall which relates to poor killing efficiency. While positive S. aureus do not have this layer [Citation49].
4. Conclusions
In this study, we introduced a rapid and “green method” to synthesize GNS using a surfactant free combining ultrasound assisted irradiation method. At optimal conditions, produced GNS obtained an average core diameter of 40 ± 4 nm, and 45 ± 6 nm of branches. GNS loaded flexible PU foams could be manufactured by a one-step (in-situ) of polytetrahydrofuran with 4,4′-Methylenebis(phenyl isocyanate). These foams were characterized as having high water absorption and small pore size of nearly 500% and 98 nm respectively. The GNS loaded PU foams exhibited great antimicrobial activity against both E. coli and S. aureus, especially at low GNS loaded concentration 5.0 µg/mL. All procedures for preparing the GNS and composite foams were simple and rapid. GNS loaded flexible polyurethane foam is an interesting biomedical material for applications such as antibacterial dressings for wound treatment.
Disclosure statement
No potential conflict of interest was reported by the authors.
Data availability statement
The data used to support the findings of this study have been deposited at https://doi.org/10.6084/m9.figshare.19688580.v1.
Additional information
Funding
Notes on contributors
Phat Trong Huynh
Phat Trong Huynh is current researcher of chemical and bio-sensors group, Research Laboratories of Saigon Hi-tech Park. His research interests are anisotropic plamonic nano-structures and their use in sensors and medical applications.
Giang Dang Nguyen
Giang Dang Nguyen is member of chemical and bio-sensors group, Research Laboratories of Saigon Hi-tech Park. His research focusing on polymers and nanocomposites.
Khanh Thi Le Tran
Khanh Thi Le Tran is current researcher of chemical and bio-sensors group, Research Laboratories of Saigon Hi-tech Park. Her current research is organic and inorganic nanomaterials preparation.
Thu Minh Ho
Thu Minh Ho is current researcher of chemical and bio-sensors group, Research Laboratories of Saigon Hi-tech Park. Her research interests are focused on microbiology and biochemistry.
Vinh Quang Lam
Vinh Quang Lam is the Head of Science and Technology, Vietnam National University of Ho Chi Minh City (VNU-HCM). Besides, he currently working as an associate professor in the Faculty of Physics and Engineering Physics, University of Science, VNU-HCM. He received his PhD at Université Lille 1, France in 2006. His researches are optical spectrum and photonics, solar cells, quantum dots, etc.
Mark Bown
Mark Bown current roles Facilty Manager of Biomedical Materials Translational Facility (CSIRO). He received his MSc at Imperial College of Science & Technology in 1984 and PhD at University of Leeds in 1987. His research is in the field of Polyurethane, Organometallic Synthesis, Organic Synthesis, Nuclear Magnetic Resonance, etc.
Thanh Vo Ke Ngo
Thanh Vo Ke Ngo received PhD from Faculty of Physics and Engineering Physics, University of Science, VNU-HCM in 2019. He is current the Director of Research Laboratories of Saigon Hi-tech Park. His research is focusing on nanomaterials and sensors.
References
- Ou Y-C, Webb JA, O'Brien CM, et al. Diagnosis of immunomarkers in vivo via multiplexed surface enhanced raman spectroscopy with gold nanostars. Nanoscale. 2018;10(27):13092–13105.
- Chen S, Fan J, Qiu W, et al. A cellular/intranuclear dual-targeting nanoplatform based on gold nanostar for accurate tumor photothermal therapy. J Mater Chem B. 2018;6(10):1543–1551.
- Priecel P, Adekunle Salami H, Padilla RH, et al. Anisotropic gold nanoparticles: Preparation and applications in catalysis. Chin J Catal. 2016;37(10):1619–1650.
- Austin LA, Kang B, El-Sayed MA. Probing molecular cell event dynamics at the single-cell level with targeted plasmonic gold nanoparticles: a review. Nano Today. 2015;10(5):542–558.
- Huynh PT, Nguyen GD, Tran KTL, et al. One-pot, surfactant-free synthesis of gold nanostars and evaluation of their antibacterial effects against propionibacterium acnes. J Nanomater. 2021;2021:1–10.
- Chhatre A, Thaokar R, Mehra A. Formation of gold nanorods by seeded growth: Mechanisms and modeling. Crystal Growth & Design. 2018;18(6):3269–3282.
- Hatakeyama Y, Sasaki K, Judai K, et al. Growth behavior of gold nanorods synthesized by the seed-mediated method: Tracking of reaction progress by Time-Resolved X-ray absorption near-edge structure, Small-Angle X-ray scattering, and ultraviolet–visible spectroscopy. J Phys Chem. C. 2018;122(14):7982–7991.
- Nafisah S, Morsin M, Sanudin R, et al. Effect of additive acid on seeded growth of gold nanobipyramids. J Phys Chem Solids. 2021;148:109764.
- Chandra K, Rugg BK, Ratner MA, et al. Detecting and visualizing reaction intermediates of anisotropic nanoparticle growth. J Am Chem Soc. 2018;140(9):3219–3222.
- Chandra K, Culver KSB, Werner SE, et al. Manipulating the anisotropic structure of gold nanostars using good’s buffers. Chem Mater. 2016;28(18):6763–6769.
- Zhu B, Gong S, Cheng W. Softening gold for elastronics. Chem Soc Rev. 2019;48(6):1668–1711.
- Savun-Hekimoğlu B. A review on sonochemistry and its environmental applications. Acoustics. 2020;2(4):766–775.
- Rezvani Ghomi E, Khalili S, Nouri Khorasani S, et al. Wound dressings: Current advances and future directions. J Appl Polym Sci. 2019;136(27):47738.
- Bell D, Hyam D. Choosing an appropriate dressing for chronic wounds. Prescriber. 2007;18(11):65–70.
- Simões D, Miguel SP, Ribeiro MP, et al. Recent advances on antimicrobial wound dressing: a review. Eur J Pharm Biopharm. 2018;127:130–141.
- Dhivya S, Padma VV, Santhini E. Wound dressings - a review. Biomedicine (Taipei). 2015;5(4):22–22.
- Aderibigbe BA, Buyana B. Alginate in wound dressings. Pharmaceutics. 2018;10(2):42.
- Andrews KL, Derby KM, Jacobson TM, et al. Prevention and management of chronic wounds. In: Cifu DX, editors. Braddom's physical medicine and rehabilitation. 6th ed. Philadelphia: Elsevier; 2021. p. 469–484.
- Tavakoli S, Klar AS. Advanced hydrogels as wound dressings. Biomolecules. 2020;10(8):1169.
- Fogh K, Nielsen J. Clinical utility of foam dressings in wound management: a review. CWCMR. 2015;2:31–38.
- Zhang X, Shi X, Gautrot JE, et al. Nanoengineered electrospun fibers and their biomedical applications: a review. Nanocomposites. 2021;7(1):1–34.
- Sudarsan S, Selvi MS, Chitra G, et al. Nontoxic pH-sensitive silver nanocomposite hydrogels for potential wound healing applications. Polymer-Plastics Technol Mater. 2021;60(1):84–104.
- Jin Y, Li J, Wu S, et al. Comparison of polyurethane foam dressing and hydrocolloid dressing in patients with pressure ulcers: a randomized controlled trial protocol. Medicine (Baltimore). 2021;100(2):e24165.
- Zhou B, Hu Y, Li J, et al. Chitosan/phosvitin antibacterial films fabricated via layer-by-layer deposition. Int J Biol Macromol. 2014;64:402–408.
- Zhou X, Zhang T, Guo D, et al. A facile preparation of poly(ethylene oxide)-modified medical polyurethane to improve hemocompatibility. Colloids Surf, A. 2014;441:34–42.
- Greenwood JE, Damkat-Thomas L, Schmitt B, et al. Successful proof of the ‘two-stage strategy’ for major burn wound repair. Burns Open. 2020;4(3):121–131.
- Eskandarinia A, Kefayat A, Agheb M, et al. A novel bilayer wound dressing composed of a dense polyurethane/propolis membrane and a biodegradable polycaprolactone/gelatin nanofibrous scaffold. Sci Rep. 2020;10(1):3063.
- Wheatley DJ, Raco L, Bernacca GM, et al. Polyurethane: material for the next generation of heart valve prostheses? Eur J Cardio-Thoracic Surg. 2000;17(4):440–448.
- Wildgruber M, Lueg C, Borgmeyer S, et al. Polyurethane versus silicone catheters for Central venous port devices implanted at the forearm. Eur J Cancer. 2016;59:113–124.
- Poelaert J, Depuydt P, De Wolf A, et al. Polyurethane cuffed endotracheal tubes to prevent early postoperative pneumonia after cardiac surgery: a pilot study. J Thoracic and Cardiovasc Surg. 2008;135(4):771–776.
- Mir M, Ali MN, Barakullah A, et al. Synthetic polymeric biomaterials for wound healing: a review. Prog Biomater. 2018;7(1):1–21.
- Pahlevanneshan Z, Deypour M, Kefayat A, et al. Polyurethane-Nanolignin composite foam coated with propolis as a platform for wound dressing: Synthesis and characterization. Polymers. 2021;13(18):3191.
- Morena AG, Stefanov I, Ivanova K, et al. Antibacterial polyurethane foams with incorporated lignin-capped silver nanoparticles for chronic wound treatment. Ind Eng Chem Res. 2020;59(10):4504–4514.
- Gu X, Xu Z, Gu L, et al. Preparation and antibacterial properties of gold nanoparticles: a review. Environ Chem Lett. 2021;19(1):167–187.
- Anees Ahmad S, Sachi Das S, Khatoon A, et al. Bactericidal activity of silver nanoparticles: a mechanistic review. Mater Sci Energy Technol. 2020;3:756–769.
- Mobed A, Hasanzadeh M, Seidi F. Anti-bacterial activity of gold nanocomposites as a new nanomaterial weapon to combat photogenic agents: recent advances and challenges. RSC Adv. 2021;11(55):34688–34698.
- Farrokhi Z, Ayati A, Kanvisi M, et al. Recent advance in antibacterial activity of nanoparticles contained polyurethane. J Appl Polym Sci. 2019;136(4):46997.
- Naik AJT, Ismail S, Kay C, et al. Antimicrobial activity of polyurethane embedded with methylene blue, toluidene blue and gold nanoparticles against staphylococcus aureus; illuminated with white light. Mater Chem Phys. 2011;129(1-2):446–450.
- Huynh PT, Nguyen GD, Le Tran KT, et al. Rapid and green preparation of multi-branched gold nanoparticles using Surfactant-Free, combined ultrasound-assisted method. Processes. 2021;9(1):112.
- Borse V, Konwar AN. Synthesis and characterization of gold nanoparticles as a sensing tool for the lateral flow immunoassay development. Sensors Int. 2020;1:100051.
- Namviriyachote N, Lipipun V, Akkhawattanangkul Y, et al. Development of polyurethane foam dressing containing silver and asiaticoside for healing of dermal wound. Asian J Pharm Sci. 2019;14(1):63–77.
- Chirico G, Borzenkov M, Pallavicini P. Gold nanostars: Synthesis, properties and biomedical application., XV, Vol. 80; 2015. Springer International Publishing.
- Oliveira MJ, de Almeida MP, Nunes D, et al. Design and simple assembly of gold nanostar bioconjugates for surface-enhanced raman spectroscopy immunoassays. Nanomaterials. 2019;9(11):1561.
- Vo T-T, Nguyen TT-N, Huynh TT-T, et al. Biosynthesis of silver and gold nanoparticles using aqueous extract from crinum latifolium leaf and their applications forward antibacterial effect and wastewater treatment. J Nanomater. 2019;2019:1–14.
- Lee SM, Park IK, Kim YS, et al. Physical, morphological, and wound healing properties of a polyurethane foam-film dressing. Biomater Res. 2016;20(1):15.
- Lee SM, Park IK, Kim YS, et al. Superior absorption and retention properties of foam-film silver dressing versus other commercially available silver dressing. Biomater Res. 2016;20(1):22.
- Piktel E, Suprewicz Ł, Depciuch J, et al. Varied-shaped gold nanoparticles with nanogram killing efficiency as potential antimicrobial surface coatings for the medical devices. Sci Rep. 2021;11(1):12546.
- Zhao Y, Jiang X. Multiple strategies to activate gold nanoparticles as antibiotics. Nanoscale. 2013;5(18):8340–8350.
- Liu TY, Chen Y, Wang HH, et al. Differentiation of bacteria cell wall using raman scattering enhanced by nanoparticle array. J Nanosci Nanotechnol. 2012;12(6):5004–5008.