Abstract
A new concept, Re-Fib, was developed within an EU project, REFORM, to recycle carbon and glass fibres from polymeric composite structures, aiming to reduce energy consumption and degradation of fibre properties during recycling. The optimized thermolysis treatment, 24 h at 380 °C, was verified able to recover clean fibres from most tested composite structures containing different thermoset resins (epoxy, vinyl ester, and polyester) and various core materials such as polyvinyl chloride (PVC), polyurethane (PU), and wood. Single-fibre test was performed in dynamic mechanical analysis (DMA). The reduction of strength was found around 26% for carbon fibres and 34–40% for glass fibres. Thermally recycled glass fibres were melt-compounded with recycled polypropylene (rPP); the resultant composites showed promising mechanical properties.
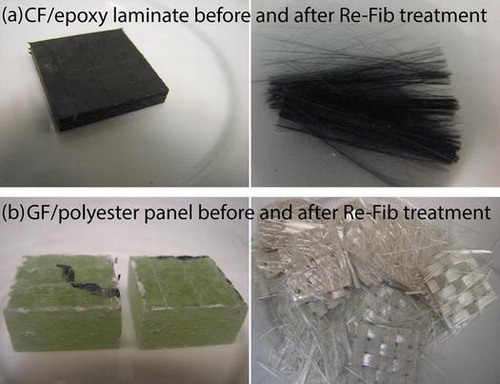
Introduction
Fibre-reinforced polymer (FRP) composite materials have been used in a wide range of applications because of their light weight, high performance-to-weight ratio, and ability to be manufactured into complex shapes. The disposal of end-of-life FRP composites, however, has been generally recognized as a serious problem. The E.U. Directive on end-of-life vehicle demands that the target for 2015 is to reuse or recycle at least 85 wt% of the waste from vehicles, with a total of 95 wt% reuse and recovery.Citation1 There are two main types of resin used for FRPs: thermoplastics and thermosets. Thermoplastics can be recycled by re-melting and re-molding. However, this is not the case for thermosets which dominate the construction, transportation and aerospace FRP market. One option for thermoset FRPs is grinding, with the resultant recyclate being used as filler in new composite materials.Citation2 Another option is to return FRP back to its original constituents, especially to reclaim the fibre reinforcement. This option may be more economically cost-effective because fibre-reinforcement has potentially the most recoverable value in a composite, particularly high-grade carbon (CF) and glass fibres (GF). The Lux report predicted that the global market for CFRPs will be more than double from $14.6 billion in 2012 to $36 billion in 2020. During the same period, demand for CF is expected to rise fourfold from the current value.Citation3 It is hence of great importance from both environmental and economical point of view to recover fibre reinforcement with minimum degradation of properties for industrial production.
Means to recover fibres from composites include dissolution of the plastic fraction using various organic and inorganic solvents,Citation4,5 and thermal processes in an atmosphere with or without oxygen.Citation6–8 Polymer matrix can be thermally decomposed to produce oil and gas products, leaving solid residues consisting of fibre, filler and char. The derived hydrocarbon liquid has properties that make it a viable fuel.Citation9 The gas could potentially provide the energy requirements for thermolysis process thus lead to lower energy consumption.Citation10 Relatively low temperature of thermolysis should minimize thermal degradation of fibres.Citation6,11 Both CFs and GFs have been thermally recycled, using pyrolysis processes in the absence of oxygen such as in a fixed-bed reactor using nitrogenCitation12 (led by the University of Leeds, UK), or a fluidized bed thermal process using hot airCitation6 (led by the University of Nottingham, UK). In a fixed-bed reactor, the pyrolysis temperature varies between 350 and 800 °C.Citation12 The tensile strength of recycled GF was found as 52% of virgin fibre at 400 °C and only 18% at 800 °C.Citation12 In a fluidized bed process,Citation8,13 the temperature can vary between 450 and 650 °C. For instance, 450 °C was used for GF/polyester composite while 550 °C for epoxy resin-based composites in order to volatilize the polymers and release the fibres. GFs typically suffer a 50% reduction in tensile strength but retain the same stiffness as the virgin fibres when processed at 450 °C, and can have a 90% reduction in strength at 650 °C; CFs show a lower strength degradation of typically 20% with retention of the original stiffness when processed at 550 °C.Citation8,13 It is found from previous studies that the thermal treatment conditions, especially temperature has a dominant effect on the recycling efficiency, which in turn varies depending on the resins and fibre reinforcement that were recycled. Too low temperature is not sufficient to decompose certain polymers, while too high temperature is detrimental to the mechanical properties especially strength of fibres. Methodology that can be a generally effective solution for disassembly of resins and core materials, and recovery of high-quality fibres from most FRP, thus needs to be developed.
In this study we report a new recycling concept, Re-Fib, a low-temperature thermolysis process that has been developed within an EU project, REFORM, by Swerea SICOMP, Sweden. FRP composite laminate and sandwich structures containing different thermoset resins and core materials were recycled using this methodology. The thermal decomposition efficiency was measured by the weight loss of composite materials. The properties of CF and GF recycled by optimal Re-Fib process were studied using single-fibre tensile test, and compared with those being recycled at higher temperature but shorter time. Recycled GFs (rGF) were compounded with recycled polypropylene (rPP); the mechanical properties of the resultant composites were studied.
Concept of Re-Fib thermolysis process
The Re-Fib concept was proposed on the basis of the idea that the use of relatively low temperatures (<400 °C) and long time should make it possible to find a steady-state situation for general composite materials where they are subjected to the same temperature and atmosphere, and hence obtain a low remaining resin content (RRC, <5 wt%). The chosen concept importantly limits the heating temperature to 380 °C to minimize the risk of decomposition of fibres and effectively remove the matrix. The used oven air atmosphere simplifies the equipment and enables lower temperature to be used than if a fully inert atmosphere is used. Due to fibre constituent the composite materials tend to become more porous with heat degradation. Our previous study has shown that the resin matrix in FRP composites degrades faster than neat resin at the same thermolysis conditions.Citation14 Furthermore, this process can make the disassembly easier for composite structures which have multi-material components, such as sandwich materials and those with metal inserts, etc., by thoroughly degrading resin and core material fractions. The recovered fibres are aimed for use in thermoset- and/or thermoplastic-composites processing. The Re-Fib process is schematically outlined in Figure .
Experimental
Materials
The used materials for recycling include epoxy, polyester, and vinyl ester resins, the CF- and GF-laminates based on these resins, as well as sandwich structures with these laminates and PVC, PU, or wood cores, provided by project partners. The commercial materials that were used in various composite laminates and sandwich structures include such as: ProBalsa and Divinycell H (Diab), Okoimè Marine Plywood (Bellotti), Atlac 580 AC 300 vinyl ester and Synolite 8488-G-2 polyester resin (DSM), Dion®9100 bisphenol-epoxy vinyl ester and Polylite 720-782 polyester (Reichhold), LY 1564 SP/Hardener XB 3486 epoxy system (Huntsman), multi-axial GF fabric 42031/M225–127-65 (Ahlstrom), etc.
For single-fibre tensile test, the virgin CF is HTS45 from TENAX® filament yarn and virgin GF is Owens Corning type 30® R25H-LS2400. Recycled CF and GF were obtained from laminates processed in SICOMP, in which the virgin fibres were used as reinforcement. CF was recycled using the optimal Re-Fib procedure, at 380 °C for 24 h. Two batches of GF were recycled, one was prepared using the Re-Fib procedure; the other one was thermally treated at 565 °C for 4 h, which is a practical protocol usually used in industry to burn out all polymeric fractions in composites to calculate the fibre fraction.
rPP (Axjo AB) with a melt flow index (MFI) of 3 g 10 min−1 was used as matrix for composites processing. The GFs recycled at 565 °C/4 h were used as reinforcement in the trial experiment due to the limited amount of rGF obtained using the optimized Re-Fib method within the project time frame. Maleic anhydride grafted PP (MAPP G-3216, Eastman) was used as a compatibilizer to improve the interfacial adhesion between rPP and the rGFs.
Re-Fib thermolysis process
For concept verification samples were placed separately in ceramic cups without cover, and then heated in an air atmosphere in a convection oven at a fixed temperature for 24 h. The samples include: (1) specimens with dimensions 20 × 20 mm (L × W) cut from cured resins and prepared/received composite structures. The thickness of samples is therefore the same as that of cured resins or the structures, ranging from 5 to 50 mm; (2) CFs in a fabric form with similar planar dimension, and (3) GFs in bundles.
Processing of recycled GF/rPP composites
Melt compounding was carried out using a twin-screw extruder (Coperion ZSK 26 K) with a screw speed of 230 rpm, polymer mass flow rate of 6.4 kg h−1 and fibre mass flow rate of 1.6 kg h−1. The rGFs (565 °C/4 h) were weighed and placed in plastic bags then manually fed into the side feed screw of the extruder. The weight of the plastic bags with rGFs was 53.3 g each for a 2-min extruder feeding procedure to ensure homogeneous fibre content in the resultant composites. The rPP was hand-mixed with MAPP, respectively, and then fed into the extruder. Three compositions were processed with varied configurations of extruder screws, and compounding temperature range along the extruder. The goal was to find a process setup that preserves the fibre length as well as provides good fibre dispersion. The composites compositions and processing details are specified in Table . Neat rPP was also processed through the extruder for property comparison. The extrudates were cut into pellets and processed into dog-bone shaped specimens using injection molding.
Table 1 Details of compositions and processing conditions of rGF/rPP composites
Characterization
Measurement of weight loss
The weight of samples before and after thermolysis was measured to calculate the weight loss of resins, core materials, sizing agent and binders as well as the possible weight loss of fibres. At least two samples were tested for each material or structure.
Tensile test of single fibre
Tensile properties of single CF and GF before and after thermolysis were measured in DMA (Q800, TA) at 25 °C. Fibres diameter was measured in a microscope Nikon LV-150 equipped with Nikon MQA11020 Digital Color Camera DS-Fi2, before being mounted in DMA. The length of fibres between the tension clamps of DMA was measured in situ by DMA, after being mounted. The length was in the range of 10–13 mm. Fibres were tested under a stress/strain mode up to break at a force ramp rate of 0.1 N min−1. The elastic modulus of the fibres was measured from the stress–strain curves in the strain range of 0.05–0.25%. The strength was recorded as the stress at break. About 10–15 fibres for each category were measured, and the average values were reported.
Mechanical characterization of rGF/rPP composites
Uniaxial tensile tests were carried out on rGF/rPP composites according to ISO527 in an MTS 20/M tensile tester. The Young’s modulus was calculated as the secant modulus between strains of 0.05 and 0.25%. At least five specimens were tested for each material.
Fibre length measurement
Pieces from injection-molded rGF/rPP composites specimens were placed separately between two sheets of a Mylar film in an oven at 200 °C. After melting the materials were pressed into thin films. The fibre length of rGF in the composites was analyzed from the thin films using a microscope Nikon LV-150 equipped with Nikon MQA11020 Digital Color Camera DS-Fi2.
Results and discussion
Evaluation of thermolysis efficiency of the Re-Fib process
First, three oven temperatures, 340, 360, and 380 °C, were used for 24-h Re-Fib treatment of two fully cured epoxy resins, RTM6 and LY556, and PU core materials for screening the optimal temperature. PU or PVC core are probably more difficult to decompose than thermoset resins, thus usually hinders the recycling of sandwich structures. The values of the residual solid content (RSC) at the three temperatures are recorded in Table . It was found that the RSC of LY556 is smaller than that of RTM6, indicating that neat LY556 resin is more prone to decompose than neat RTM6 resin. Around 15–20 and 5–10 wt% of epoxy resins still remain when heated for 24 h at 340 and 360 °C, respectively; but can almost completely decompose when heated at 380 °C for 24 h. The RSC of PU foam is around 20 and 10 wt% when heated for 24 h at 340 and 360 °C, respectively, which is reduced to around 5 wt% at 380 °C. Thermolysis at 380 °C for 24 h in air atmosphere was therefore selected as the optimal condition and used for subsequent Re-Fib treatment of other materials and composite structures.
Table 2 Residual solid content (RSC) of 24-h Re-Fib thermolysis at three temperatures
Table lists the weight loss of different cured thermoset resins and core materials as the constituents of prepared/supplied composite structures, and of neat CF and GF (brand name is confidential due to customers’ requirement). The results indicate that all the tested constituents can be sufficiently decomposed when heated at 380 °C for 24 h. The weight loss of neat CF and GF also coincides with the content values of sizing agents and thermoplastic binders provided by the suppliers.
Table 3 Weight loss of different constituents of composite structures and of neat CF and GF
Figure exemplifies the appearance of CF/epoxy and GF/polyester laminates before and after the optimal Re-Fib treatment, which also reveals that almost all the resin fractions were thermally decomposed and clean fibres were obtained.
Figure 2 Appearance of (a) CF/epoxy laminates processed in SICOMP (Sweden) and (b) GF/polyester panel provided by Azimut Benetti (Italy) before (left) and after (right) Re-Fib treatment at 380 °C for 24 h
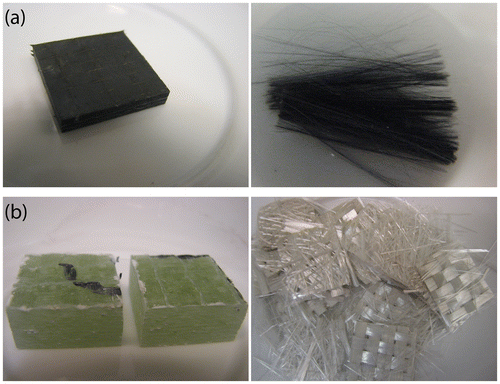
(a) CF/epoxy laminates processed in SICOMP (Sweden); (b) GF/polyester panel provided by Azimut Benetti (Italy).
Figure exemplifies the appearance of sandwich structure composed of GF/polyester laminates and PU core before and after the thermolysis for 24 h at 340, 360, and 380 °C, respectively. The images reveal that the sandwich structure still keeps integrated when heated for 24 h at the two lower temperatures, only the surface of laminates start to decompose. At the optimal Re-Fib condition; 380 °C/24 h, the PU core and polyester resin in the laminates are sufficiently decomposed, leading to a successful disassembly of the sandwich structure and recovery of clean GFs.
Figure 3 Appearance of sandwich structure composted of GF/polyester laminates and PU core: (a) before thermolysis, (b) after thermolysis at 340 °C for 24 h, (c) after thermolysis at 360 °C for 24 h, and (d) after thermolysis at 380 °C for 24 h.
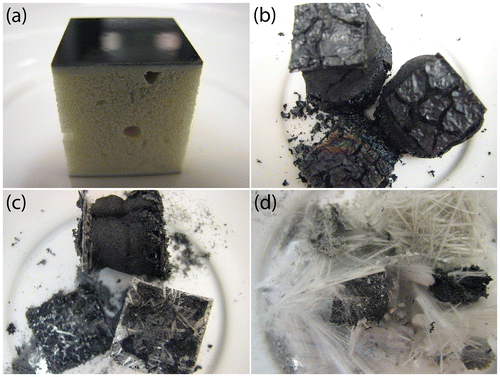
(a) before thermolysis; (b) after thermolysis at 340 °C for 24 h; (c) after thermolysis at 360 °C for 24 h; (d) after thermolysis at 380 °C for 24 h.
Single-fibre tensile properties
Figure represents the typical stress–strain curves of virgin and recycled CF and GF via optimal Re-Fib treatment (380 °C/24 h), where “V” refers to virgin fibres, and “R” recycled fibres.
Figure 4 Stress–strain curves of single-fibre tensile test in DMA at a force ramp rate of 0.1 N min−1 for CF and GF before and after optimal Re-Fib thermolysis (380 °C/24 h)
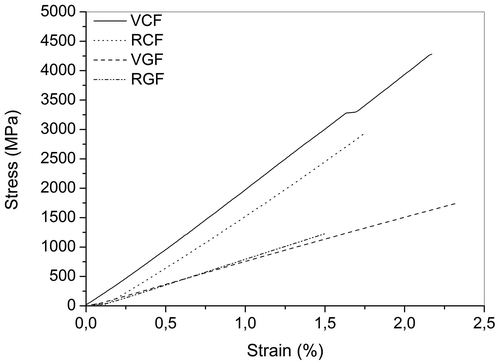
Table lists the values of modulus and strength of the fibres measured in the DMA single-fibre test. These values of both virgin CF and GF are close to those provided by suppliers. The difference can be due to different measurement methods. The modulus of CF and GF were found almost retained after the optimal Re-Fib heat treatment, only around 1–3% of reduction was observed. On the other hand, the decrease in strength cannot be neglected, however, more reduction was observed in GF. The decrement of strength is around 26% for CF and 34–40% for GF. These values are found very close to the ones reported in previous studies.Citation15,16 In those studies, other recycling means with much shorter cycle time were used, such as pyrolysis using fixed-bed reactor in which samples are usually held at the prescribed temperature for 60 min,Citation12 and microwave heating which can be processed at 3 kW for 8 s.Citation16
Table 4 Modulus and strength of single CF and GF measured in DMA
The tensile properties of GFs thermally recycled at 565 °C for 4 h were also measured at the same force ramp rate. Their modulus was found very close to that of GFs recycled by the optimal Re-Fib process, while the strength was around 50% lower, i.e. only around 10–20% of that of virgin GFs. The comparison of these results indicates that the temperature instead of time in thermolysis has a dominant effect not only on decomposition efficiency, but also on the properties of the recycled fibres. Higher temperature may benefit the acceleration of decomposition but impair more the fibre properties.
Fibre length measurement and mechanical properties of rGF/rPP composites
Fibre length distribution in injection molded test-bars from the studied rGF/rPP composites materials are shown in Figure . It can be seen that in Composite 1 and 2 the percentage of fibres shorter than 300 μm is higher than in Composite 3. Above 300 μm the percentage of fibres is higher in Composite 3. Given the same injection molding parameters were used for all composites, the results therefore show that screw configuration in the extrusion step affects the fibre length; a configuration with many dispersing and kneading elements leads to a more extensive fibre breakage, while a screw configuration without these types of elements preserves better the fibre length.
Figure 5 Fibre length distribution in injection molded test-bars from the rGF/rPP composite materials processed with three different screw configurations in the extrusion step
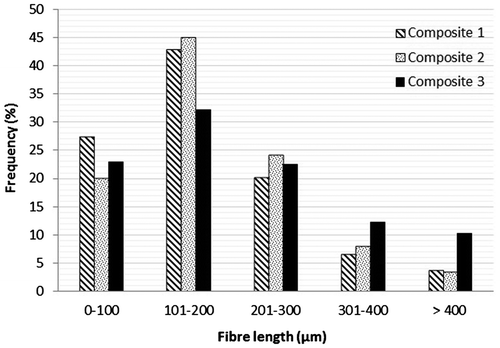
The results of uniaxial tensile test of the rGF/rPP composites are listed in Table . It is shown that the modulus and strength of the matrix was enhanced around 180 and 53%, respectively, with the addition of rGF.
Table 5 Mechanical properties of neat rPP and rGF/rPP composites. The GFs were recycled at 565 °C for 4 h
Critical fibre length, lc for efficient stress transfer can be expressed as:
(1)
where τ is the shear strength of either the matrix or the interface, df is the fibre diameter and σfu is the strength of the fibre. The composite will break due to the weakest link, either the interfacial shear strength, the shear strength of the matrix or the fibre strength. The lc of the recycled GF in the studied composite system can be estimated according to the following: (1) the ratio between shear strength and tensile strength of PP is assumed to be 0.8, as found in an earlier study,Citation17 the upper limit for the interfacial shear strength of this system should be around 22 MPa; (2) the measured fibre diameter ~22 μm; and (3) the strength of the recycled fibre is 50% (deducted from literature) of the virgin fibre. The lc should accordingly be 800 μm or longer for the recycled fibres. Thus most of the fibres found in these composites are shorter than lc. However, Composite 3 has the highest percentage of fibres longer than 400 μm, closest to the predicted lc, which could explain that it has the highest strength (Figure and Table ).
In addition, the quality of fibre reinforcement itself has an important impact on the physical properties of the resultant polymeric composites. The fibres recycled via the optimal Re-Fib process have better properties, especially strength, than those via common “burning” treatment (565 °C/4 h). Higher performance of composites using Re-Fib recycled fibres as reinforcement is therefore expected, which can be more promising for being used in industrial applications.
Conclusions
The low-temperature thermolysis, Re-Fib, has been applied to recycle clean carbon and glass fibres with promising mechanical properties from polymer composites that have been used in construction and automotive industries. The optimized conditions, heating at 380 °C for 24 h, work for most tested composite materials. Different common thermoset resin such as epoxy, polyester, and vinyl ester as well as core materials such as PU, PVC, and wood, can be decomposed sufficiently. The weight loss of these materials is more than 97%.
The modulus of virgin fibres can almost be retained after Re-Fib process, only around 1–3% reduction was observed for both carbon and glass fibres. The reduction of strength is about 26% for carbon fibres and 34–40% for glass fibres. These values are similar with those reported in other studies using fixed- or fluidized-bed reactor and microwave heat treatment. However, Re-Fib process uses a relatively low temperature for composite materials containing different resin matrices and core materials. This process therefore demands less handling and sorting of composite materials for oven input, and simplifies the parameter setting.
Fibres recycled using optimal Re-Fib process show better quality, especially strength, than those fibre recycled by thermolysis at higher temperature but shorter time. Although the latter were used for composites compounding, promising mechanical properties were achieved. The Re-Fib recycled fibres are hence expected to achieve better reinforcing effect in composites that can be used for industrial applications.
Reaction kinetic modeling in combination with the decomposition mechanisms and life cycle analysis are the focus of ongoing work as a continuation of the project, which will be specified in a coming paper.
Disclosure statement
No potential conflict of interest was reported by the authors.
Acknowledgement
Hard work from Runar Långström and Erwan Juin at Swerea SICOMP, Sweden, is appreciated.
Funding
This work was supported by the European Union’s Seventh Framework Programme for research, technological development and demonstration [grant number 283336]. Part of the work was done in the project Swerea Industrial Recycling funded by RISE, Sweden.
References
- Official J. Eur. Communities, 2000, 34, L269.
- G. Hobbs and S. Halliwell: ‘Recycling of plastics and polymer composites’, Composites and plastics in construction conference, Watford, UK, September 1999.
- Cutting costs of carbon composites, SAE International, 27 February 2013. http://articles.sae.org/11618/
- M. Buggy, L. Farragher and W. Madden: ‘Recycling of composite materials’, J. Mater. Proc. Technol., 1995, 55, 448–456.10.1016/0924-0136(95)02037-3
- S. H. Patel, K. E. Gonsalves, S. S. Stivala, L. Reich and D. H. Trivedi: ‘Alternative procedures for the recycling of sheet molding compounds’, Adv. Polym. Technol., 1993, 12, 35–45.10.1002/adv.1993.060120104
- S. J. Pickering: ‘Recycling technologies for thermoset composite materials-current status’, Composites A, 2006, 37, 1206–1215.10.1016/j.compositesa.2005.05.030
- J. R. Kennerley, R. M. Kelly, N. J. Fenwick, S. J. Pickering and C. D. Rudd: ‘The characterisation and reuse of glass fibres recycled from scrap composites by the action of a fluidised bed process’, Composites A, 1998, 29, 839–845.10.1016/S1359-835X(98)00008-6
- S. J. Pickering, R. M. Kelly, J. R. Kennerley, C. D. Rudd and N. J. Fenwick: ‘A fluidised bed process for the recovery of glass fibres from scrap thermoset composites’, Compos. Sci. Technol., 2000, 60, 509–523.10.1016/S0266-3538(99)00154-2
- M. Day, J. D. Cooney and Z. Shen: ‘Pyrolysis of automobile shredder residue: an analysis of the products of a commercial screw kiln process’, J. Anal Appl. Pyrolysis, 1996, 37, 49–67.10.1016/0165-2370(96)00938-2
- C. Roy and P. Mallette: ‘Vacuum pyrolysis of automotive shredder waste’, Symposium Papers-energy from Biomass and Wastes, Inst. Gas Technol., Chicago, 1991, 827–839.
- W. F. Thomas: ‘An investigation into the factors likely to affect the strength and properties of glass fibres’, Phys. Chem. Glasses, 1960, 1, 4–18.
- A. M. Cunliffe, N. Jones and P. T. Williams: ‘Pyrolysis of composite plastic waste’, Environ. Technol., 2003, 24, 653–663.10.1080/09593330309385599
- H. L. H. Yip, S. J. Pickering and C. D. Rudd: ‘Characterisation of carbon fibres recycled from scrap composites using fluidised bed process’, Plast. Rubber Compos., 2002, 31, 278–282.10.1179/146580102225003047
- K. Olofsson, G. Gong, B. Nyström, A. H. Praturlon and S. Durante: ‘Recycling fibres from composites using Re-Fib method’, ICMAC Conference Bristol Science Centre, Britain, 25 June 2015.
- P. Jenkins, J. Thomason and R. Meier: ‘Separation of mechanical and thermal degradation of thermally conditioned sized glass fibre’, ECCM 15–15th European Conference on Composite Materials, Venice, Italy, June 2012, 24–28.
- E. Lester, S. Kingman, K. H. Wong, C. Rudd, S. Pickering and N. Hilal: ‘Microwave heating as a means for carbon fibre recovery from polymer composites: a technical feasibility study’, Mater. Res. Bull., 2004, 39, 1549–1556.10.1016/j.materresbull.2004.04.031
- B. Nyström, R. Joffe and R. Långström: ‘Microstructure and strength of injection molded natural fibre composites’, J. Reinf. Plast. Comp., 2007, 26, 579–599.10.1177/0731684407075536