ABSTRACT
Introduction
In spite of the substantial advances in clinical practice, Gastric cancer (GC) remains the third leading cause of cancer death worldwide. The incidence of drug resistance remains a hindrance to effective treatment for GC. Although the molecular mechanisms of chemoresistance have broadly studied, the gene regulation and expression mechanisms of miRNA have not entirely understood.
Methods
Online databases of PubMed, Scopus, Google Scholar, and Embase databases were searched to retrieve relevant publications. The following keywords were used: MicroRNA, Non-coding RNA, miRNA, Gastric cancer, drug resistance, and chemoresistance.
Results
miRNAs play a pivotal role in the initiation, progression of tumor and metastasis, as well as in the development of pathways mediating resistance to chemotherapy in GC. Unluckily, to date, there is no consistent, reliable biomarker available to predict the response of chemotherapy before the start of the treatment.
Discussion
In this review, we would like to provide an overview of the miRNAs and miRNA facilitated chemoresistance machinery in GC to develop a personalized treatment to overcome GC drug resistance.
1. Introduction
Gastric cancer (GC) remains the fourth most common cancer worldwide. Recent data indicate that one million new cases of GC are diagnosed each year in globally [Citation1]. The average incidence of GC is extremely variable by region and is more commonly diagnosed in developed countries. The incidence rate is highest in Eastern (32.1/100,000 males and 13.2 females) and Central Asian populations [Citation2]. At present, radiotherapy, combinational chemotherapy, and surgery are the main therapeutic options for GC. However, in many patients these treatments are not successful due to the resistance of chemotherapeutic Drugs [Citation3]. The tumor intrinsic (preexistent) or acquired (drugs induced), mechanisms of resistance, makes a major obstacle to develop effective cancer therapies. In recent years, due to endoscopy and surgery in an early stage of GC, the overall 5-year mortality rate was expressively reduced up to 67%, while in advanced GC it remains only 50% [Citation4]. In advanced GC, lymph node metastasis, peritoneal dissemination, and rectal metastasis via hematogenous accelerate the cell proliferation, migration, invasion leading to recurrence of cancer cells and mortality with several molecular pathways [Citation5,Citation6].
MicroRNA (miRNAs) are 18–25 bp short noncoding RNAs that bind to the 3ʹ- untranslated region (UTR), specifically in the MRE sequence (miRNA recognition element) and inhibit the gene expression [Citation7]. Emerging evidence demonstrated that miRNAs play a pivotal role in the progression of tumor and metastasis, as well as chemoresistance in GC [Citation8–Citation12]. In this review, we will emphasized on the role of miRNA mediated chemoresistance mechanisms in GC and provide novel insights in the field of miRNA for GC diagnosis and prognosis.
2. The biological significance of miRNA
miRNAs are highly conserved endogenous small noncoding sequences which are involved in the normal functioning of eukaryotic cells. Lee et al. discovered the first miRNA lin-4 in the 3ʹ untranslated region (UTR) of C. elegans [Citation13]. miRNA genes are transcribed by RNA polymerase (II and III) from non-coding (introns), coding (exons) regions, generating initial precursors that undergo a series of cleavage events by the Drosha/DGCR8. pri-miRNA also regulated by several transcription factors (TFs), and finally, pri-miRNA transcribed as mature miRNA in the cytoplasm [Citation14,Citation15]. Further, it is predicted that single miRNAs can combine with many different mRNAs and may regulate the expression of > 60% protein-coding genes in human genome [Citation16]. Concerning the miRNA’s biological relevance, these small molecules are regulatory elements in various biological processes, including cell differentiation, proliferation, and apoptosis and The miRNA regulate the multiple genes involved in cancer progression and metastasis [Citation17,Citation18].
3. miRNA and drug resistance in GC
To date, more than 2000 miRNAs have been identified, and some of them are significantly associated with tumorigenesis, cancer invasion, metastasis and also apoptosis in GC [Citation19]. A recent study demonstrated that one single miRNA could act as an oncogenic miRNA as well as tumor suppressor miRNAs which seem to have a significant role in drug resistance [Citation20]. The relationship between miRNA expression and sensitivity of GC to chemotherapeutic drugs is studied extensively. A recent study reported that the miR-551b-3p directly binds to the intronic region of dysbindin mRNA and negatively regulates the expression and involved in Platinum resistance in GC cells [Citation21]. Further, decreased miR‐122 expression is directly involved in the induction of cisplatin (CDDP) resistance by increasing excision repair cross‐complementing 1 (ERCC1) expression [Citation22]. Xi et al. showed that metastasis-associated lung adenocarcinoma transcript 1 (MALAT1), which potentiates autophagy-associated CDDP resistance by regulating the miR-30b in GC [Citation23]. Furthermore, Wang et al. Showed that overexpression of miR-574-3p reduced the migratory and invasive properties of the GC cells and inhibit the EMT and enhanced cisplatin sensitivity in GC cells by suppressing in vitro and in vivo [Citation24]. Recentaly, Tao et al revealed that miR-92a contributes role in cell proliferation, apoptosis and doxorubicin chemosensitivity in GC cells, and suggests a potential therapeutic strategy for the treatment of GC [Citation25].
& documents the features of 39 miRNAs that regulate the chemoresistance mechanisms in GC. Among them, 29 miRNA were down-regulated and significantly decreased sensitivity to chemo drugs, and 10 are up-regulated in different resistant pathways.
Figure 1. miRNAs associated with chemoresistance in gastric cancer. Drug resistance related to the red and green boxes represents miRNA with expression level,5-FU(5-fluorouracil) MDR (multi-drug resistance), CDDP(cisplatin), and Others.
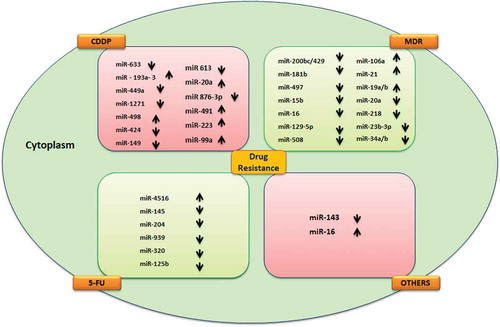
Table 1. Compendium of MicroRNAs involved in gastric cancer drug resistance.
4. Prognostic prediction and therapeutic response of GC
The prognosis of GC remains poor due to the slow progress in the development of diagnostic and therapeutic machinery. Tumor-node-metastasis staging (TNM staging) system is the most widely used independent predictor of survival for GC individuals [Citation63]. On the other hand, TNM staging is alone not enough to predict the prognosis of GC in diverse stages [Citation64]. Therefore, it is very crucial to identify key factors that predict the overall survival and response rate to the existing therapeutic options. However, GC linked to tumor extension and nodal involvement beyond the gastric wall; It may provide some vital information to predict GC [Citation65]. The overexpression of miR-93 is associated with reduced cellular response to transforming growth factor (TGF-β) by influencing the synthesis of p21, a well known apoptotic protein [Citation66]. Overexpression of miR-93 was strongly associated with GC to predict poor survival rate. Apart from this, altered expression of miR-125 showed as a significant prognostic factor for survival of GC patients [Citation67,Citation68]. Zhang et al. reported that high expression of miR-21-5p is associated with poor overall survival (OS) and disease-free survival (DFS) in GC. Further, they found that overexpression of miR-200c-3p, miR-222-3p might also influence the prognosis of GC [Citation69].
Down-regulation of miR-376a may contribute to the in tumorigenesis and progression of GC and act as a potential therapeutic candidate and help to predict prognosis of GC [Citation70]. Notably, the identification of miRNAs as a prognostic factor of GC will not only aid in predicting the prognosis, but also help to develop new therapeutic targets in the future. Therefore, further analysis of miRNAs is very crucial in GC therapy.
5. miRNAs in the identification of gastric cancer
Despite accumulating data on genetic and epigenetic events in GC, the lack of adequate noninvasive tools for early identification of GC remains the most significant challenge. Currently, serum tumor markers such as carcinoembryonic antigen (CEA), carbohydrate antigen 724 (CA724) and carbohydrate antigen 199 (CA199) are in use for detecting GC. However, serum tumor markers have poor sensitivity and less specificity [Citation71]. Thus, it is an urgent requirement to discover novel diagnostic agents to detect the GC. Since the last decade, miRNAs are gathering a lot of scientific attention and opening new windows to find out a centered way to detect GC in early stage. From this perspective, in silico research identified 14 miRNAs of which 3 miRNAs (miR-211, let-7b, and miR-708) were first time reported and could be used as a novel potential biomarker in GC after further clinical validation [Citation72]. An experimental data of Korean cohort distinguished miR-24 as stage 1 and stage 2 diagnostic markers in GC patients. Shin and colleagues showed that miR-627, miR-629 and miR-652 were significantly up-regulated in GC and play a role as a crucial noninvasive diagnostic marker [Citation73]. Hwang et al. showed that dysregulation of miR-107, miR-300, miR-26a, and miR-375 in an early stages of GC [Citation74]. The above evidences indicated that miRNAs are promising candidates serving as novel prognostic marker in the early stage of GC.
6. Invasion and miRNAs
Several studies demonstrated that dysregulation of miRNAs has been involved in various cancer progression mechanisms including invasion and metastasis. Recently, Kim et al. observed that overexpression of hsa-miR-421/hsa-miR-29b-1-5p in MKN-74 cells might play an essential role in the migration of GC and prevented the progression of GC [Citation75]. Mei et al. showed that miR-1275 overexpression could suppress the invasion and metastasis of GC through targeting of JAZF1 [Citation76]. Wang et al. noticed that up-regulation of Wnt7a suppressed the miR-127 expression and reversed the ability of GC cell migration and invasion [Citation77]. Xu et al. demonstrated that speckle-type POZ protein (SPOP) could inhibit cell migration and invasion by downregulation of miR-543 in GC cells [Citation78]. Qiu et al. found that miR-26a and miR-148a were down-regulated in GC tissues and overexpression of miR-26 inhibit the proliferation, migration, invasion, in GC cell line [Citation79]. Interestingly, miR-610 inhibits the migration and invasion of GC cells by suppressing the expression of vasodilator-stimulated phosphoprotein (VASP) [Citation80]. Similarly, miR-181a directly inhibits caprin-1 and promotes proliferation, apoptosis, migration, and invasion in GC [Citation81].
7. Histological behaviors of miRNAs in GC
On the basis of gene expression, GC cell lines and patients tissue can be classified into three broad categories, intestinal, diffuse and undifferentiated, which overlain with the histological Lauren’s classification [Citation82]. Jiang et al. found that miR-421 was overexpressed in GC tissues but had no significant association with the histological behaviors [Citation83]. The weighted gene correlation network analysis (WGCNA) showed that miR 99a, 100, let-7c and 125b associated with the diffuse histological subtype. Beside miR181 family and 21 miR were associated with tumoral phenotype [Citation84]. Recently, Pereira et al. observed that seven miRNAs were differentially expressed in both GC and adjacent GC tissue among them, hsa-miR-200a-3p and hsa-miR-873-5p have diagnostic value for detection of early and advanced disease.
Further, hsa-miR-196a-5p was differentially expressed between adjacent to cancer and GC tissues [Citation85]. The bioinformatics analyses revealed that miR-124 and miR-29b is involved in the tumor stage and serve as a potential biomarker in histological classification of GC [Citation11]. Also, miR-383 levels were down-regulated in intestinal-type GC as compared with non-tumor tissues; this phenomenon was maybe the promising factor to discriminate intestinal GC patients from healthy controls [Citation86].
8. miRNAs associated with GC stages
The TNM staging is an anatomically based system that primarily used in solid tumor staging to describe the extent and to spread of cancer in a patient. Serum concentrations of miR-21, miR-146a, and miR-148a were significantly associated with pN stage, but not in pT stage. This result suggests that miR may be potential candidates to predict the presence of Lymph Node metastasis in GC [Citation87]. Overexpression of miR-29, miR-196a/196b and miR-630 were found to be associated with GC invasion and metastasis and TNM staging system [Citation88–Citation90]. Wu et al. found that miR-21 Level in serum and peripheral blood mononuclear cells (PBMCs) was significantly associated with early (stage I) and late GC (stage IV) [Citation91]. Recently it was identified that the overexpression of hsa-miR-15b, hsa-miR-18a, hsa-miR-19a, hsa-miR-20a, hsa-miR-22, hsa-let-7i, hsa-miR-93, hsa-miR-106b hsa-miR-196a, hsa-miR-196b, hsa-miR-221, hsa-miR-503, and hsa-miR-545 were significantly associated with GC stages I, II and III compared with normal controls [Citation92].
9. miRNAs and recurrence of GC
GC is clinically heterogeneous and recurrence is the major hindrance to achieve better outcomes. The Cancer Genome Atlas (TCGA) genome-wide data Identified that 312 differentially expressed miR were differentially expressed in 446 GC tissues and signature to predict recurrence in patients with GC [Citation93]. Yan et al. demonstrated that the up-regulation of hsa-miR-335 has the potential responsible for the recurrence risk and relates to the prognosis of GC patients [Citation94]. Imaoka et al. showed that downregulation of serum miR-203 significantly involved in the prediction of metastases, early recurrence, and poor prognosis in human GC [Citation95].
Similarly down-regulated serum miR206 level might not only serve as a diagnostic biomarker but also predict cancer recurrence for GC [Citation96]. A frozen and formalin-fixed paraffin-embedded tissue microarray-based study showed that the combination of hsa-miR-375 and hsa-miR-142-5p may act as a positive indicator for recurrence and poor survival of GC [Citation97]. Xue et al. found overexpression of miR-328 in early GC tissues and recurrence-free rate was significantly longer in those with relatively high expression of miR-328. This data indicate that miR-328 can function as a predictive biomarker of recurrence [Citation98].
10. Clinical diagnostic of miRNA in GC
Due to the poor diagnosis, lack of specific treatment options and resistance to chemotherapeutic drugs, the detection of biomarkers for GC remains a major challenge. Hence, there is an urgent need to establish a reliable method for early detection and diagnosis of GC stages as well as novel treatments to overcome chemoresistance. Until now, there is no prominent biomarker to diagnose GC and its stages efficiently. Over the past years, researchers are considering circulating miRNAs as dynamic budding biomarkers since they work as a tumor suppressor gene as well as oncogene and involved in cell proliferation, differentiation, chemoresistance and metastasis [Citation99]. As miRNAs can prognosticate response to chemotherapy, they can be used as a promising clinical candidate to target GC. Further, the proficiency of response to chemoresistance implies that the expression pattern of various circulating miRNAs carrying significant differences between patients who are chemoresistance and chemosensitive. Different expression patterns of circulating miRNAs in laboratory specimens might help us to discriminate between responder patients and non-responders to a specific treatment.
In some in vitro studies, demonstrated variations in the expression of different miRNAs between chemotherapy resistance GC cell lines and sensitive control CG cell lines. For instance, upregulation of miR-19a and miRNA-19b was observed in a vincristine (VCR) and doxorubicin (DOX) resistant GC cell lines [Citation100]. Significantly upregulated miRNA-21 and miRNA-106a was found in cisplatin-resistant GC cell lines as compared to controls [Citation101,Citation102]. Another study showed that the level of miRNA-195 and miR-378 were upregulated in GC cell lines which are resistant to 5-azacytidine [Citation103]. Downregulation of miRNAs namely miR-15b and miRNA-16 was found in a VCR resistant GC [Citation104]. Moreover, downregulation of miR-508-5p was found in GC cell lines which are resistant toward DOX and VCR [Citation105]. Downregulation of miR-181b and miR-497 was found in VCR resistant GC cell [Citation106,Citation107]. Further, downregulation of miR-567 in GC tissue as compared to healthy tissues and enhanced chemotherapeutic sensitization to 5-FU and oxaliplatin [Citation108].]. A meta-analysis conducted using the expression patterns of 35 circulating miRNAs in GC patients versus healthy controls reported that the miR-21 is most consistently upregulated in GC cells [Citation109].
11. Therapeutic potential
Conventional therapies are too toxic or ineffective due to chemoresistance. Further, many targeted therapies alone are inadequately effective and will have to be used in combination with other therapeutic targets. In the recent era, application of diverse miRNAs as a modulator of chemotherapy sensitivity is an appealing approach as a significant therapeutic aide with traditional chemotherapeutics. Artificial manipulation of specific miRNAs of different concentrations can also help to accelerate sensitivity or decrease resistance toward various chemotherapy drugs. In addition, alteration of miRNA expression leads to resistance of the different malignant tumors to already established chemotherapy treatment was observed. Some recent findings suggest that circulating miRNA based therapy may be an aggressive and challenging way to fight GC.
On the one hand, miRNA based therapy has the potential to significantly inhibit the oncomiRNA by overexpressing the suppressed miRNA and suppressing oncomiRNA by targeting respective signaling pathways, eventually which may result in the decreased neoplastic progression [Citation110,Citation111]. For instance, some studies revealed that miR-34 suppresses oncomiRNA in a few cancer cells, including liver, breast, lung and Gastric cancer [Citation112–Citation114]. Ji et al. found that restoration of miR-34 was significantly associated with inhibition of GC tumorspheres by facilitating the expression of mutated TP53 gene [Citation115]. Besides, Wang et al. reported that the miR-34 specifically targeting the three prime 3ʹUTR and inhibits the expression of Yin Yang1 (YY1) which was results in reduced tumor growth and proliferation in SC-M1 and AZ521 GC cells [Citation116]. In addition, miRNAs can significantly influence drug-metabolizing enzymes, drug transporters, nuclear receptors, and transcription factors. The knockdown of miR-21 can substantially promote the anti-proliferative effects and apoptosis by CDDP [Citation102]. Furthermore, time to progress (TPP) associated chemoresistance miRNA expression profile for gastric cancer patients getting CDDP and FU [Citation117]. Till date it is reported a total of 58 miRNAs linked to the TTP of gastric cancer patients and able to modulate apoptosis in vitro [Citation118]. This study identified miRNA-517, miR-518f, miR-519e, miR- 520a, miR-520d, miR-363 that correlated with chemoresistance which, can be used as an aggressive candidate to predict the responsiveness of GC patients to FU chemotherapy. In addition to this, several studies have identified various miRNA expression profiles during drug induced MDR in GC cell lines, which might be useful to predict the chemotherapy responses in GC cells.
12. MiRNAs modulating GC chemoresistance pathways
Various signaling pathways involved in the GC chemoresistance and expression of miRNA during chemoresistance were summarized in .
Table 2. Summary of Molecular signaling pathways involved in miRNA induced chemoresistance in Gastric cancer.
13. PTEN/PI3K/AKT/miRNAs signaling
Several studies demonstrated that the phosphatase and tensin homolog (PTEN)/phosphatidylinositol 3-kinase (PI3K)/protein kinase B (Akt) is most frequently mutated pathways in all cancers including GC. Dysregulated expression of various miRNAs are correlated with different cancerous phenotypes, including neoplasm growth, angiogenesis, and metastasis by regulating relative signaling pathways in GC. The modulation of PTEN expression is directly associated with the aberrant expression of the miRNA-221/222 cluster in GC cells [Citation121]. PTEN is also an appealing target for miRNA-21 and miRNA-214 that promote rapid proliferation and invasion of GC cells [Citation122,Citation123]. In addition to this, expression of miRNA-375 directly linked to suppression of PI3K/Akt signaling through direct targeting 3-phosphoinositide dependent kinase-1 (PDK1), an activator of Akt eventually that was resulting in reduced viable cells through induction of the apoptotic pathway [Citation124]. Microarray data has also supported that the miRNA-375 is downregulated in GC. It is also noted that the upregulation of miRNA-28 helps in GC cell proliferation and invasion by downregulating PTEN [Citation125]. Expression of macrophage migration inhibitory factor (MIF) was found to promote GC cell proliferation via the PI3K/Akt signaling [Citation126]. Downregulated miRNA-451 expression was found in GC,restoration of miRNA-451 leads to the downregulation of MIF that results in decreased GC cell proliferation in reaction to irradiation [Citation127].
14. RAS/RAF/MEK/ERK/miRNAs signaling
The rat sarcoma 2 viral oncogene homolog (RAS)/rapidly accelerated fibrosarcoma (RAF)/MAPK/ERK kinase (MEK)/extracellular signal-regulated kinase (ERK) is a central signaling transduction component that contributes in certain cellular functions in normal homeostasis. It is also termed as mitogen-activated protein kinases (MAPK) that known for its unique ability to convey signals from cell surface receptors to transcription factors in the nucleus [Citation128]. Overexpression of KRAS protein in GC is associated with the expression of miRNA-181c [Citation129]. The miRNA-29s family significantly influencing the Ras/Raf/MEK/ERK signaling pathway by decreasing the expression of Cdc42 and the level of phosphorylation of its downstream molecule PAK1, thereby resulting in reduced GC cell proliferation [Citation130]. The miRNA-107 as a tumor suppressor and potentially reduced the expression of cyclin-dependent protein kinase 6 (CDK6) that leads to a decline in GC cell proliferation [Citation131]. One recent study demonstrated that the increased expression of miRNA-939 reduces the proliferation, migration, and invasion in GC and contributes to chemosensitivity via inhibiting SLC34A2/Raf/MEK/ERK cascade [Citation132].
15. Slit/Robo/miRNAs signaling
The Roundabout (ROBO) is a transmembrane receptor that binds to SLIT, a secretory glycoprotein ligand that modulate the function of neurons and glia, leukocytes, and endothelial cell migration [Citation133]. Slit-Robo is plays a crucial role in tumor progression by taking part in the cell cycle, cell adhesion, motility, angiogenesis, apoptosis, and invasion [Citation134]. The dysregulation of the Slit-Robo pathway modulates several oncogenic signaling pathways that are coupled with the growth and progression of cancer. The miRNA-218 genes transcribe together with Slit genes, which binds to the Robo1 receptor, thus creating a negative feedback loop that negatively regulates Slit/Robo1 signaling [Citation135]. A recent study showed that the expression of miRNA-218 increases the methylation of SLIT2 or SLIT3 [Citation136].
16. JAK/STAT/miRNAs signaling
The JAK/STAT signaling cascade is one of the principal molecular signaling mechanisms such as cell growth, proliferation, differentiation, cell migration, apoptosis, inflammation, and survival of immune cells [Citation137]. The JAK/STAT interaction is specifically concerned for a wide array of growth factors and cytokines such as IFNα, IFN-γ and IL-6 mediated signaling [Citation138]. Janus kinase family consists of four members coding as JAK1, JAK2, JAK3, and TYK2. JAK1, JAK2, and TYK2 are ubiquitously expressed cytoplasmic non-receptor. The expression of JAK3 is only restricted to the hematopoietic cells and responsible for hematopoietic differentiation. The miRNA-375 reduced GC cell proliferation and metastasis by targeting the JAK2 gene [Citation139]. The miRNA-135a represses p-STAT3 by targeting JAK2 thus resulting in reduced expression of cyclin D1 and Bcl-xL and suppressed GC metastasis [Citation140]. Further, overexpression of miRNA-18a in GC which negatively regulates the expression of PIAS3, thereby resulting in increased STAT3 signaling [Citation141]. A recent study demonstrated that the miRNA-340 affects GC cell proliferation, cell cycle blocking, and promoting apoptosis through SOCS3/JAK-STAT signaling pathway [Citation142].
17. WNT/beta-Catenin/TCF/miRNAs signaling
The canonically known WNT/beta-catenin signaling cascade is activated by the binding of WNT glycoproteins as a ligand to membrane-bound Frizzled receptors and co-receptor namely low-density lipoprotein receptor-related protein (LRP5/6) eventually phosphorylate Disheveled (Dsh) by requiting it in the membrane. Dsh inhibits the phosphorylation of b-catenin by disrupting the Wnt-Fz-LRP5/6 complex consisting of the adenomatous polyposis coli, Axin and glycogen synthase kinase-3b proteins which resulting in the stabilization of b-catenin. Further, this accumulated unphosphorylated beta-catenin travels to the nucleus and forms complex with transcription factors of the T-cell factor/lymphoid-enhancer factor (TCF/LEF). This complex activates WNT target for transcription specific genes i.e. c-Myc, cyclin D1, gastrin and ITF-2 [Citation143,Citation144]. The miRNA-27a inhibits the expression of Sp-1 repressor mRNA (ZBTB10) that contribute to GC cell survival, cell growth and angiogenesis [Citation145]. Increased expression of miRNA-10b caused a decrease in MAPRE1 expression which may serve as a direct and appealing target of GC [Citation146]. Besides this, another study showed that MAPRE1 might also function as an oncogene through activating the beta-catenin/TCF signaling to promote cell growth and inhibit cell death via apoptosis in GC [Citation147]. The miR-27 by modulating the WNT signal transduction pathway induce GC growth and metastasis [Citation148]. Further, miR-144, the‐miR-148a, and miR-193b, were significantly downregulated in GC patients through WNT/beta-catenin pathway [Citation149,Citation150].
18. Concluding remarks
Standard tumor markers, such as serum levels of CA 72–4, carcinoembryonic antigen (CEA), CA 19–9, and alpha-fetoprotein (AFP) are commonly used for diagnosing advanced disease but not in the early stage of GC patients due to its poor sensitivity and specificity. miRNA regulates expression of proteins that modulate chemosensitivity and chemoresistance to a cancer drug. Many studies found that alterations in miRNA expression differ for specific phases and types of GC, suggesting miRNAs may play a functional as well as novel and potential targets for the treatment of GC.
The expression of specific miRNA might moderately improve chemosensitivity and potentially enhance the antitumor properties of certain drugs. Another rising strategy that can modify gene specific limitations is the machinery of genome editing by clustered regulatory interspaced short palindromic repeats-associated endonuclease 9 (CRISPR-Cas9), which is a quick and efficient ways to down or upregulate the genes, including miRNAs. At present, the comprehensive understanding of the emerging role of miRNA in chemoresistance is unknown. However, further research still needed for the investigation of the sensitivity and specificity of miRNAs that can control GC progression. Overcoming the above challenges will help us to use the miRNAs as biomarkers for the precise management of GC. Many researchers agree that, in near future miRNA will be used as the most important diagnostic and prognostic tool.
Disclosure statement
No potential conflict of interest was reported by the authors.
Additional information
Funding
Notes on contributors
Henu Kumar Verma
Henu Kumar Verma has completed his M.Sc in Medical Biotechnology from Pt. J.N.M. Medical College, Raipur, India. Mr. Verma is International Marie Curie Ph.D fellow at Institute of Endocrinology and oncology CNR, Naples, Italy. He has published more than 25 peer-reviewed international papers and a book chapter in reputed journals. His field of specialization is Molecular Biology, Genetics, Immunology, Cancer Diagnostics and Cell Biology.
Yashwant Kumar Ratre
Yashwant kumar Ratre has completed his M.Sc in Medical Biotechnology from Pt. J.N.M. Medical College, Raipur, India. Currently Mr. Ratre is Ph.D fellow at Guru Ghasidas University, Bilaspur, India. He has published 5 peer-reviewed international papers in reputed journals. His field of specialization is Molecular Biology and Genetics.
Pellegrino Mazzone
Dr. Pellegrino Mazzone has completed his Ph.D. in Bioinformatics and Life Science from the University of Studies of sannio, Benevento. Mazzone has joined Biogem scientific research lab, Italy as a postdoctoral fellow in 2014. He has published more than 12 peer-reviewed international papers in reputed journals. His field of specialization is Cell Biology, Immunology, Cancer Diagnostics and Molecular Biology
Simona Laurino
Dr. Simona Laurino has completed her Ph.D. in Molecular Life Science and Medicine. Laurino has joined IRCCS Centro di Riferimento Oncologico della Basilicata (CROB) as a postdoctoral fellow in 2017. She has published more than 15 peer-reviewed international papers in reputed journals. His field of specialization is Molecular Biology, Cancer Biology, Cancer Diagnostics, and Cell Biology.
L. V. K. S. Bhaskar
Dr. L.V.K.S. Bhaskar is a Professor of Zoology and Dean of the School of Life Sciences at Guru Ghasidas Central University, Chhattisgarh. Prior to this appointment, Dr. Bhaskar was the Senior Scientist at Sickle Cell Institute, Chhattisgarh. Dr. Bhaskar was awarded the Prof. Pampapathi Rao memorial Gold Medal in 2003. Dr. Bhaskar is an active member of several genetics and biotechnology-related professional and academic bodies. He has conducted research to explore molecular basis of alcoholism, genetics of complex human diseases, population genetics, and cancer biology. Dr. Bhaskar has published more than 150 research papers in reputed national and international journals. Dr. Bhaskar has been a regular reviewer for several national and international journals and also serves on the editorial board of various journals.
References
- Bray F, Ferlay J, Soerjomataram I, et al. Global cancer statistics 2018: GLOBOCAN estimates of incidence and mortality worldwide for 36 cancers in 185 countries. CA Cancer J Clin. 2018;68(6):394–424.
- Balakrishnan M, George R, Sharma A, et al. Changing trends in stomach cancer throughout the world. Curr Gastroenterol Rep. 2017;19(8):36.
- Holohan C, Van Schaeybroeck S, Longley DB, et al. Cancer drug resistance: an evolving paradigm. Nat Rev Cancer. 2013;13(10):714–726.
- Hamashima C, Shabana M, Okada K, et al. Mortality reduction from gastric cancer by endoscopic and radiographic screening. Cancer Sci. 2015;106(12):1744–1749.
- Deng JY, Liang H. Clinical significance of lymph node metastasis in gastric cancer. World J Gastroenterol. 2014;20(14):3967–3975.
- Verma HK, Falco G, Bhaskar LVKS, Molecular Signaling Pathways Involved in Gastric Cancer Chemoresistance. In Theranostics Approaches to Gastric and Colon Cancer, Raju GSR, Bhaskar LVKS (eds.); Springer Singapore: Singapore, 2020; 117-134.
- Qi M, Liu D, Zhang S. MicroRNA-21 contributes to the discrimination of chemoresistance in metastatic gastric cancer. Cancer Biomark. 2017;18(4):451–458.
- Zeng J-F, Ma X-Q, Wang L-P, et al. MicroRNA-145 exerts tumor-suppressive and chemo-resistance lowering effects by targeting CD44 in gastric cancer. World J Gastroenterol. 2017;23(13):2337–2345.
- Russi S, Verma HK, Laurino S, et al. Adapting and surviving: intra and extra-cellular remodeling in drug-resistant gastric cancer cells. Int J Mol Sci. 2019;20(15):3736.
- da Silva Oliveira KC, Thomaz Araújo TM, Albuquerque CI, et al. Role of miRNAs and their potential to be useful as diagnostic and prognostic biomarkers in gastric cancer. World J Gastroenterol. 2016;22(35):7951–7962.
- Cheng J, Zhuo H, Xu M, et al. Regulatory network of circRNA–miRNA–mRNA contributes to the histological classification and disease progression in gastric cancer. J Transl Med. 2018;16(1):216.
- Hao N-B, He Y-F, Li X-Q, et al. The role of miRNA and lncRNA in gastric cancer. Oncotarget. 2017;8(46):81572–81582.
- Lee RC, Feinbaum RL, Ambros V. The C. elegans heterochronic gene lin-4 encodes small RNAs with antisense complementarity to lin-14. Cell. 1993;75(5):843–854.
- Lee Y, Kim M, Han J, et al. MicroRNA genes are transcribed by RNA polymerase II. Embo J. 2004;23(20):4051–4060.
- Schanen BC, Li X. Transcriptional regulation of mammalian miRNA genes. Genomics. 2011;97(1):1–6.
- Catalanotto C, Cogoni C, Zardo G. MicroRNA in control of gene expression: an overview of nuclear functions. Int J Mol Sci. 2016;17(10):1712.
- Hwang HW, Mendell JT. MicroRNAs in cell proliferation, cell death, and tumorigenesis. Br J Cancer. 2007;96 Suppl:R40–R44.
- Feng Y, Bai F, You Y, et al. Dysregulated microRNA expression profiles in gastric cancer cells with high peritoneal metastatic potential. Exp Ther Med. 2018;16(6):4602–4608.
- Li W, Ng JM-K, Wong CC, et al. Molecular alterations of cancer cell and tumour microenvironment in metastatic gastric cancer. Oncogene. 2018;37(36):4903–4920.
- Li Y, Zhang Q, Tang X. Long non-coding RNA XIST contributes into drug resistance of gastric cancer cell. Minerva Med. 2019;110(3):270–272.
- Guo X, Wang X, Li S, et al. 27PLncRNA-GC1 contributes to gastric cancer chemo-resistance through inhibition of miR-551b-3p and the overexpression of dysbindin. Ann Oncol. 2019;30(Supplement_5):v8.
- Song A-L, Zhao L, Wang Y-W, et al. Chemoresistance in gastric cancer is attributed to the overexpression of excision repair cross-complementing 1 (ERCC1) caused by microRNA-122 dysregulation. J Cell Physiol. 2019;234(12):22485–22492.
- Xi Z, Si J, Nan J. LncRNA MALAT1 potentiates autophagyassociated cisplatin resistance by regulating the microRNA30b/autophagyrelated gene 5 axis in gastric cancer. Int J Oncol. 2019;54(1):239–248.
- Wang M, Zhang R, Zhang S, et al. MicroRNA-574-3p regulates epithelial mesenchymal transition and cisplatin resistance via targeting ZEB1 in human gastric carcinoma cells. Gene. 2019;700:110–119.
- Tao XC, Zhang X-Y, Sun S-B, et al. miR‑92a contributes to cell proliferation, apoptosis and doxorubicin chemosensitivity in gastric carcinoma cells. Oncol Rep. 2019;42(1):313–320.
- Liu YP, Sun X-H, Cao X-L, et al. MicroRNA-217 suppressed epithelial-to-mesenchymal transition in gastric cancer metastasis through targeting PTPN14. Eur Rev Med Pharmacol Sci. 2017;21(8):1759–1767.
- Zhu W, Xu H, Zhu D, et al. miR-200bc/429 cluster modulates multidrug resistance of human cancer cell lines by targeting BCL2 and XIAP. Cancer Chemother Pharmacol. 2012;69(3):723–731.
- Wang T, Ge G, Ding Y, et al. MiR-503 regulates cisplatin resistance of human gastric cancer cell lines by targeting IGF1R and BCL2. Chin Med J (Engl). 2014;127(12):2357–2362.
- Zhuang M, Shi Q, Zhang X, et al. Involvement of miR-143 in cisplatin resistance of gastric cancer cells via targeting IGF1R and BCL2. Tumour Biol. 2015;36(4):2737–2745.
- Hu J, Fang Y, Cao Y, et al. miR-449a Regulates proliferation and chemosensitivity to cisplatin by targeting cyclin D1 and BCL2 in SGC7901 cells. Dig Dis Sci. 2014;59(2):336–345.
- Wu H, Huang M, Lu M, et al. Regulation of microtubule-associated protein tau (MAPT) by miR-34c-5p determines the chemosensitivity of gastric cancer to paclitaxel. Cancer Chemother Pharmacol. 2013;71(5):1159–1171.
- Sacconi A, Biagioni F, Canu V, et al. miR-204 targets Bcl-2 expression and enhances responsiveness of gastric cancer. Cell Death Dis. 2012;3(11):e423.
- Li L-Q, Pan D, Chen Q, et al. Sensitization of gastric cancer cells to 5-FU by microRNA-204 through targeting the TGFBR2-mediated epithelial to mesenchymal transition. Cell Physiol Biochem. 2018;47(4):1533–1545.
- Li Y, Lv S, Ning H, et al. Down-regulation of CASC2 contributes to cisplatin resistance in gastric cancer by sponging miR-19a. Biomed Pharmacother. 2018;108:1775–1782.
- Yang M, Shan X, Zhou X, et al. miR-1271 regulates cisplatin resistance of human gastric cancer cell lines by targeting IGF1R, IRS1, mTOR, and BCL2. Anticancer Agents Med Chem. 2014;14(6):884–891.
- Zhang Y, Qu X, Li C, et al. miR-103/107 modulates multidrug resistance in human gastric carcinoma by downregulating Cav-1. Tumour Biol. 2015;36(4):2277–2285.
- Li X, Zhang Z, Yu M, et al. Involvement of miR-20a in promoting gastric cancer progression by targeting early growth response 2 (EGR2). Int J Mol Sci. 2013;14(8):16226–16239.
- Du Y, Zhu M, Zhou X, et al. miR-20a enhances cisplatin resistance of human gastric cancer cell line by targeting NFKBIB. Tumour Biol. 2016;37(1):1261–1269.
- Takagi T, Iio A, Nakagawa Y, et al. Decreased expression of microRNA-143 and −145 in human gastric cancers. Oncology. 2009;77(1):12–21.
- Wu Q, Yang Z, Xia L, et al. Methylation of miR-129-5p CpG island modulates multi-drug resistance in gastric cancer by targeting ABC transporters. Oncotarget. 2014;5(22):11552–11563.
- Zhang XL, Shi H-J, Wang J-P, et al. MiR-218 inhibits multidrug resistance (MDR) of gastric cancer cells by targeting Hedgehog/smoothened. Int J Clin Exp Pathol. 2015;8(6):6397–6406.
- Cheng Y, Lin C, Xin C, et al. [Expression profiling and functional analysis of hsa-miR-125b and its target genes in drug-resistant cell line of human gastric cancer]. Yi Chuan. 2014;36(2):119–126.
- An Y, Zhang Z, Shang Y, et al. miR-23b-3p regulates the chemoresistance of gastric cancer cells by targeting ATG12 and HMGB2. Cell Death Dis. 2015;6(5):e1766.
- Li X, Liang J, Liu Y-X, et al. miR-149 reverses cisplatin resistance of gastric cancer SGC7901/DDP cells by targeting FoxM1.. Die Pharmazie. 2016;71(11):640–643.
- Xu Y-C, Liu X, Li M, et al. A novel mechanism of doxorubicin resistance and tumorigenesis mediated by microRNA-501-5p-suppressed BLID. Mol Ther Nucleic Acids. 2018;12:578–590.
- Liu X, Lu Y, Xu Y, et al. Exosomal transfer of miR-501 confers doxorubicin resistance and tumorigenesis via targeting of BLID in gastric cancer. Cancer Lett. 2019;459:122–134.
- Peng C, Huang K, Liu G, et al. MiR-876-3p regulates cisplatin resistance and stem cell-like properties of gastric cancer cells by targeting TMED3. J Gastroenterol Hepatol. 2019;34(10):1711–1719.
- Xue M, Li G, Sun P, et al. MicroRNA-613 induces the sensitivity of gastric cancer cells to cisplatin through targeting SOX9 expression. Am J Transl Res. 2019;11(2):885–894.
- Zhao Y, Dong Q, Wang E. MicroRNA-320 inhibits invasion and induces apoptosis by targeting CRKL and inhibiting ERK and AKT signaling in gastric cancer cells. Onco Targets Ther. 2017;10:1049–1058.
- Pang X, Zhou Z, Yu Z, et al. Foxo3a-dependent miR-633 regulates chemotherapeutic sensitivity in gastric cancer by targeting Fas-associated death domain. RNA Biol. 2019;16(2):233–248.
- Wang F, Song X, Li X, et al. Noninvasive visualization of microRNA-16 in the chemoresistance of gastric cancer using a dual reporter gene imaging system. PLoS One. 2013;8(4):e61792.
- Zhang Y, Lu Q, Cai X. MicroRNA-106a induces multidrug resistance in gastric cancer by targeting RUNX3. FEBS Lett. 2013;587(18):3069–3075.
- Jingyue S, Xiao W, Juanmin Z, et al. TFAP2E methylation promotes 5fluorouracil resistance via exosomal miR106a5p and miR421 in gastric cancer MGC803 cells. Mol Med Rep. 2019;20(1):323–331.
- Eto K, Iwatsuki M, Watanabe M, et al. The microRNA-21/PTEN pathway regulates the sensitivity of HER2-positive gastric cancer cells to trastuzumab. Ann Surg Oncol. 2014;21(1):343–350.
- Jin B, Liu Y, Wang H. Antagonism of miRNA-21 sensitizes human gastric cancer cells to paclitaxel. Cell Biochem Biophys. 2015;72(1):275–282.
- Zhu M, Zhou X, Du Y, et al. miR-20a induces cisplatin resistance of a human gastric cancer cell line via targeting CYLD. Mol Med Rep. 2016;14(2):1742–1750.
- Zhou X, Jin W, Jia H, et al. MiR-223 promotes the cisplatin resistance of human gastric cancer cells via regulating cell cycle by targeting FBXW7. J Exp Clin Cancer Res. 2015;34(1):28.
- Liu X, Ru J, Zhang J, et al. miR-23a targets interferon regulatory factor 1 and modulates cellular proliferation and paclitaxel-induced apoptosis in gastric adenocarcinoma cells. PLoS One. 2013;8(6):e64707.
- Hu Y, Song J, Liu L, et al. microRNA-4516 contributes to different functions of epithelial permeability barrier by targeting poliovirus receptor related protein 1 in Enterovirus 71 and Coxsackievirus A16 infections. Front Cell Infect Microbiol. 2018;8:110.
- Lee SD, Yu D, Lee DY, et al. Upregulated microRNA-193a-3p is responsible for cisplatin resistance in CD44(+) gastric cancer cells. Cancer Sci. 2019;110(2):662-673.
- Lee SD, Yu D, Lee DY, et al. Upregulated microRNA-193a-3p is responsible for cisplatin resistance in CD44(+) gastric cancer cells. Cancer Sci. 2019;110(2):662–673.
- Zhao T, Chen Y, Sheng S, et al. Upregulating microRNA-498 inhibits gastric cancer proliferation invasion and chemoresistance through inverse interaction of Bmi1. Cancer Gene Ther. 2019;26(11-12):366-373.
- Siewert JR, Böttcher K, Stein HJ, et al. Relevant prognostic factors in gastric cancer: ten-year results of the German gastric cancer study. Ann Surg. 1998;228(4):449–461.
- Seevaratnam R, Cardoso R, Mcgregor C, et al. How useful is preoperative imaging for tumor, node, metastasis (TNM) staging of gastric cancer? A meta-analysis. Gastric Cancer. 2012;15(Suppl S1):S3–S18.
- Zubarayev M, Min E-K, Son T. Clinical and molecular prognostic markers of survival after surgery for gastric cancer: tumor-node-metastasis staging system and beyond. Transl Gastroenterol Hepatol. 2019;4:59.
- Petrocca F, Visone R, Onelli MR, et al. E2F1-regulated microRNAs impair TGFbeta-dependent cell-cycle arrest and apoptosis in gastric cancer. Cancer Cell. 2008;13(3):272–286.
- Yu BQ, Su LP, Li JF, et al. microRNA expression signature of gastric cancer cells relative to normal gastric mucosa. Mol Med Rep. 2012;6(4):821–826.
- Chen L, Jiang M, Yuan W, et al. Prognostic value of miR-93 overexpression in resectable gastric adenocarcinomas. Acta Gastro-enterologica Belgica. 2012;75(1):22–27.
- Zhang L, Huang Z, Zhang H, et al. Prognostic value of candidate microRNAs in gastric cancer: A validation study. Cancer Biomark. 2017;18(3):221–230.
- Zhang C, Liang Y, Ma MH, et al. Downregulation of microRNA-376a in gastric cancer and association with poor prognosis. Cell Physiol Biochem. 2018;51(5):2010–2018.
- Shimada H, Noie T, Ohashi M, et al. Clinical significance of serum tumor markers for gastric cancer: a systematic review of literature by the task force of the Japanese gastric cancer association. Gastric Cancer. 2014;17(1):26–33.
- Yan W, Wang S, Sun Z, et al. Identification of microRNAs as potential biomarker for gastric cancer by system biological analysis. Biomed Res Int. 2014;2014:901428.
- Shin VY, Ng EKO, Chan VW, et al. A three-miRNA signature as promising non-invasive diagnostic marker for gastric cancer. Mol Cancer. 2015;14(1):202.
- Huang Y, Zhu J, Li W, et al. Serum microRNA panel excavated by machine learning as a potential biomarker for the detection of gastric cancer. Oncol Rep. 2018;39(3):1338–1346.
- Kim YJ, Jeong S, Jung WY, et al. miRNAs as potential biomarkers for the progression of gastric cancer inhibit CREBZF and regulate migration of gastric adenocarcinoma cells. Int J Med Sci. 2020;17(6):693–701.
- Mei J-W, Yang Z-Y, Xiang H-G, et al. MicroRNA-1275 inhibits cell migration and invasion in gastric cancer by regulating vimentin and E-cadherin via JAZF1. BMC Cancer. 2019;19(1):740.
- Wang L, Wang X, Jiang X. miR-127 suppresses gastric cancer cell migration and invasion via targeting Wnt7a. Oncol Lett. 2019;17(3):3219–3226.
- Xu J, Wang F, Wang X, et al. miRNA-543 promotes cell migration and invasion by targeting SPOP in gastric cancer. Onco Targets Ther. 2018;11:5075–5082.
- Qiu X, Zhu H, Liu S, et al. Expression and prognostic value of microRNA-26a and microRNA-148a in gastric cancer. J Gastroenterol Hepatol. 2017;32(4):819–827.
- Wang J, Zhang J, Wu J, et al. MicroRNA-610 inhibits the migration and invasion of gastric cancer cells by suppressing the expression of vasodilator-stimulated phosphoprotein. Eur J Cancer. 2012;48(12):1904–1913.
- Lu Q, Chen Y, Sun D, et al. MicroRNA-181a functions as an oncogene in gastric cancer by targeting Caprin-1. Front Pharmacol. 2019;9(1565). DOI:10.3389/fphar.2018.01565.
- Alessandrini L, Manchi M, De Re V, et al. Proposed molecular and miRNA classification of gastric cancer. Int J Mol Sci. 2018;19(6):1683.
- Jiang Z, Guo J, Xiao B, et al. Increased expression of miR-421 in human gastric carcinoma and its clinical association. J Gastroenterol. 2010;45(1):17–23.
- Yepes S, López R, Andrade RE, et al. Co-expressed miRNAs in gastric adenocarcinoma. Genomics. 2016;108(2):93–101.
- Pereira A, Moreira F, Vinasco-Sandoval T, et al. miRNome reveals new insights into the molecular biology of field cancerization in gastric cancer. Front Genet. 2019;10(592). DOI:10.3389/fgene.2019.00592.
- Azarbarzin S, Safaralizadeh R, Kazemzadeh M, et al. The value of miR-383, an intronic miRNA, as a diagnostic and prognostic biomarker in intestinal-type gastric cancer. Biochem Genet. 2017;55(3):244–252.
- Kim SY, Jeon TY, Choi CI, et al. Validation of circulating miRNA biomarkers for predicting lymph node metastasis in gastric cancer. J Mol Diagn. 2013;15(5):661–669.
- Chu D, Zhao Z, Li Y, et al. Increased microRNA-630 expression in gastric cancer is associated with poor overall survival. PLoS One. 2014;9(3):e90526.
- Tsai -M-M, Wang C-S, Tsai C-Y, et al. MicroRNA-196a/-196b promote cell metastasis via negative regulation of radixin in human gastric cancer. Cancer Lett. 2014;351(2):222–231.
- Gong J, Li J, Wang Y, et al. Characterization of microRNA-29 family expression and investigation of their mechanistic roles in gastric cancer. Carcinogenesis. 2014;35(2):497–506.
- Wu J, Li G, Wang Z, et al. Circulating microRNA-21 is a potential diagnostic biomarker in gastric cancer. Dis Markers. 2015;2015:435656.
- Yuan C, Zhang Y, Tu W, et al. Integrated miRNA profiling and bioinformatics analyses reveal upregulated miRNAs in gastric cancer. Oncol Lett. 2019;18(2):1979–1988.
- Yang Y, Qu A, Zhao R, et al. Genome-wide dentification of a novel miRNA-based signature to predict recurrence in patients with gastric cancer. Mol Oncol. 2018;12(12):2072–2084.
- Yan Z, Xiong Y, Xu W, et al. Identification of hsa-miR-335 as a prognostic signature in gastric cancer. PLoS One. 2012;7(7):e40037.
- Imaoka H, Toiyama Y, Okigami M, et al. Circulating microRNA-203 predicts metastases, early recurrence, and poor prognosis in human gastric cancer. Gastric Cancer. 2016;19(3):744–753.
- Hou CG, Luo XY, Li G. Diagnostic and prognostic value of serum microRNA-206 in patients with gastric cancer. Cell Physiol Biochem. 2016;39(4):1512–1520.
- Zhang X, Yan Z, Zhang J, et al. Combination of hsa-miR-375 and hsa-miR-142-5p as a predictor for recurrence risk in gastric cancer patients following surgical resection. Ann Oncol. 2011;22(10):2257–2266.
- Xue H-G, Yang AH, Sun XG, et al. Expression of microRNA-328 functions as a biomarker for recurrence of early gastric cancer (EGC) after endoscopic submucosal dissection (ESD) by modulating CD44. Med Sci Monit. 2016;22:4779–4785.
- Bras-Rosario L, Matsuda A, Pinheiro AI, et al. Expression profile of microRNAs regulating proliferation and differentiation in mouse adult cardiac stem cells. PLoS One. 2013;8(5):e63041.
- Wang F, Li T, Zhang B, et al. MicroRNA-19a/b regulates multidrug resistance in human gastric cancer cells by targeting PTEN. Biochem Biophys Res Commun. 2013;434(3):688–694.
- Fang Y, Shen H, Li H, et al. miR-106a confers cisplatin resistance by regulating PTEN/Akt pathway in gastric cancer cells. Acta Biochim Biophys Sin (Shanghai). 2013;45(11):963–972.
- Yang S-M, Huang C, Li X-F, et al. miR-21 confers cisplatin resistance in gastric cancer cells by regulating PTEN. Toxicology. 2013;306:162–168.
- Deng H, Guo Y, Song H, et al. MicroRNA-195 and microRNA-378 mediate tumor growth suppression by epigenetical regulation in gastric cancer. Gene. 2013;518(2):351–359.
- Xia L, Zhang D, Du R, et al. miR-15b and miR-16 modulate multidrug resistance by targeting BCL2 in human gastric cancer cells. Int J Cancer. 2008;123(2):372–379.
- Shang Y, Zhang Z, Liu Z, et al. miR-508-5p regulates multidrug resistance of gastric cancer by targeting ABCB1 and ZNRD1. Oncogene. 2014;33(25):3267–3276.
- Zhu W, Shan X, Wang T, et al. miR-181b modulates multidrug resistance by targeting BCL2 in human cancer cell lines. Int J Cancer. 2010;127(11):2520–2529.
- Zhu W, Zhu D, Lu S, et al. miR-497 modulates multidrug resistance of human cancer cell lines by targeting BCL2. Med Oncol. 2012;29(1):384–391.
- Zhang F, Li K, Yao X, et al. A miR-567-PIK3AP1-PI3K/AKT-c-Myc feedback loop regulates tumour growth and chemoresistance in gastric cancer. EBioMedicine. 2019;44:311–321.
- Zhu X, Lv M, Wang H, et al. Identification of circulating microRNAs as novel potential biomarkers for gastric cancer detection: a systematic review and meta-analysis. Dig Dis Sci. 2014;59(5):911–919.
- Pichler M, Calin GA. MicroRNAs in cancer: from developmental genes in worms to their clinical application in patients. Br J Cancer. 2015;113(4):569–573.
- Riquelme I, Letelier P, Riffo-Campos A, et al. Emerging role of miRNAs in the drug resistance of gastric cancer. Int J Mol Sci. 2016;17(3):424.
- Wang R, Ma J, Wu Q, et al. Functional role of miR-34 family in human cancer. Curr Drug Targets. 2013;14(10):1185–1191.
- Misso G, Di Martino MT, De Rosa G, et al. Mir-34: a new weapon against cancer? Mol Ther Nucleic Acids. 2014;3:e194.
- Zhang DG, Zheng JN, Pei DS. P53/microRNA-34-induced metabolic regulation: new opportunities in anticancer therapy. Mol Cancer. 2014;13(1):115.
- Ji Q, Hao X, Meng Y, et al. Restoration of tumor suppressor miR-34 inhibits human p53-mutant gastric cancer tumorspheres. BMC Cancer. 2008;8(1):266.
- Wang A-M, Huang -T-T, Hsu K-W, et al. Yin Yang 1 is a target of microRNA-34 family and contributes to gastric carcinogenesis. Oncotarget. 2014;5(13):5002–5016.
- Kim CH, Kim HK, Rettig RL, et al. miRNA signature associated with outcome of gastric cancer patients following chemotherapy. BMC Med Genomics. 2011;4(1):79.
- Smid D, KULDA V, SRBECKA K, et al. Tissue microRNAs as predictive markers for gastric cancer patients undergoing palliative chemotherapy. Int J Oncol. 2016;48(6):2693–2703.
- Lu C, Shan Z, Li C, et al. MiR-129 regulates cisplatin-resistance in human gastric cancer cells by targeting P-gp. Biomed Pharmacother. 2017;86:450–456.
- Li X, Lv Y, Hao J, et al. Role of microRNA-4516 involved autophagy associated with exposure to fine particulate matter. Oncotarget. 2016;7(29):45385–45397.
- Chun-Zhi Z, Lei H, An-ling Z, et al. MicroRNA-221 and microRNA-222 regulate gastric carcinoma cell proliferation and radioresistance by targeting PTEN. BMC Cancer. 2010;10(1):367.
- Xiong X, Ren H-Z, Li M-H, et al. Down-regulated miRNA-214 induces a cell cycle G1 arrest in gastric cancer cells by up-regulating the PTEN protein. Pathol Oncol Res. 2011;17(4):931–937.
- Zhang BG, LI JF, YU BQ, et al. microRNA-21 promotes tumor proliferation and invasion in gastric cancer by targeting PTEN. Oncol Rep. 2012;27(4):1019–1026.
- Tsukamoto Y, Nakada C, Noguchi T, et al. MicroRNA-375 is downregulated in gastric carcinomas and regulates cell survival by targeting PDK1 and 14-3-3zeta. Cancer Res. 2010;70(6):2339–2349.
- Li L, Zhu X, Shou T, et al. MicroRNA-28 promotes cell proliferation and invasion in gastric cancer via the PTEN/PI3K/AKT signalling pathway. Mol Med Rep. 2018;17(3):4003–4010.
- Li GQ, Xie J, Lei X-Y, et al. Macrophage migration inhibitory factor regulates proliferation of gastric cancer cells via the PI3K/Akt pathway. World J Gastroenterol. 2009;15(44):5541–5548.
- Bandres E, Bitarte N, Arias F, et al. microRNA-451 regulates macrophage migration inhibitory factor production and proliferation of gastrointestinal cancer cells. Clin Cancer Res. 2009;15(7):2281–2290.
- Liu F, Yang X, Geng M, et al. Targeting ERK, an Achilles’ Heel of the MAPK pathway, in cancer therapy. Acta Pharm Sin B. 2018;8(4):552–562.
- Hashimoto Y, Akiyama Y, Otsubo T, et al. Involvement of epigenetically silenced microRNA-181c in gastric carcinogenesis. Carcinogenesis. 2010;31(5):777–784.
- Lang N, Liu M, Tang Q-L, et al. Effects of microRNA-29 family members on proliferation and invasion of gastric cancer cell lines. Chin J Cancer. 2010;29(6):603–610.
- Feng L, Xie Y, Zhang H, et al. miR-107 targets cyclin-dependent kinase 6 expression, induces cell cycle G1 arrest and inhibits invasion in gastric cancer cells. Med Oncol. 2012;29(2):856–863.
- Zhang J-X, Xu Y, Gao Y, et al. Decreased expression of miR-939 contributes to chemoresistance and metastasis of gastric cancer via dysregulation of SLC34A2 and Raf/MEK/ERK pathway. Mol Cancer. 2017;16(1):18.
- Dickinson RE, Dallol A, Bieche I, et al. Epigenetic inactivation of SLIT3 and SLIT1 genes in human cancers. Br J Cancer. 2004;91(12):2071–2078.
- Mehlen P, Delloye-Bourgeois C, Chédotal A. Novel roles for Slits and netrins: axon guidance cues as anticancer targets? Nature Reviews Cancer. 2011;11(3):188–197.
- Tie J, Pan Y, Zhao L, et al. MiR-218 inhibits invasion and metastasis of gastric cancer by targeting the Robo1 receptor. PLoS Genet. 2010;6(3):e1000879.
- Kim M, KIM J-H, BAEK S-J, et al. Specific expression and methylation of SLIT1, SLIT2, SLIT3, and miR-218 in gastric cancer subtypes. Int J Oncol. 2016;48(6):2497–2507.
- Bousoik E, Montazeri Aliabadi H. “Do We Know Jack” About JAK? A Closer Look at JAK/STAT Signaling Pathway. Front Oncol. 2018;8(287). DOI:10.3389/fonc.2018.00287
- Furqan M, Mukhi N, Lee B, et al. Dysregulation of JAK-STAT pathway in hematological malignancies and JAK inhibitors for clinical application. Biomark Res. 2013;1(1):5.
- Ding L, Xu Y, Zhang W, et al. MiR-375 frequently downregulated in gastric cancer inhibits cell proliferation by targeting JAK2. Cell Res. 2010;20(7):784–793.
- Wu H, Huang M, Cao P, et al. MiR-135a targets JAK2 and inhibits gastric cancer cell proliferation. Cancer Biol Ther. 2012;13(5):281–288.
- Wu W, Takanashi M, Borjigin N, et al. MicroRNA-18a modulates STAT3 activity through negative regulation of PIAS3 during gastric adenocarcinogenesis. Br J Cancer. 2013;108(3):653–661.
- Xiao C, Hong H, Yu H, et al. MiR-340 affects gastric cancer cell proliferation, cycle, and apoptosis through regulating SOCS3/JAK-STAT signaling pathway. Immunopharmacol Immunotoxicol. 2018;40(4):278–283.
- He T-C, Sparks AB, Rago C, et al. Identification of c-MYC as a target of the APC pathway. Science. 1998;281(5382):1509–1512.
- Shtutman M, Zhurinsky J, Simcha I, et al. The cyclin D1 gene is a target of the beta-catenin/LEF-1 pathway. Proc Natl Acad Sci U S A. 1999;96(10):5522–5527.
- Scott GK, Mattie MD, Berger CE, et al. Rapid alteration of microRNA levels by histone deacetylase inhibition. Cancer Res. 2006;66(3):1277–1281.
- Kim K, Lee H-C, Park J-L, et al. Epigenetic regulation of microRNA-10b and targeting of oncogenic MAPRE1 in gastric cancer. Epigenetics. 2011;6(6):740–751.
- Liu M, Yang S, Wang Y, et al. EB1 acts as an oncogene via activating beta-catenin/TCF pathway to promote cellular growth and inhibit apoptosis. Mol Carcinog. 2009;48(3):212–219.
- Zhang Z, Liu S, Shi R, et al. miR-27 promotes human gastric cancer cell metastasis by inducing epithelial-to-mesenchymal transition. Cancer Genet. 2011;204(9):486–491.
- Zhou H, Wang K, Hu Z, et al. TGF-beta1 alters microRNA profile in human gastric cancer cells. Chin J Cancer Res. 2013;25(1):102–111.
- Liu D, Hu X, Zhou H, et al. Identification of aberrantly expressed miRNAs in gastric cancer. Gastroenterol Res Pract. 2014;2014:473817.