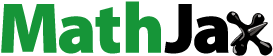
ABSTRACT
Introduction
The effects of chronic administration of phenytoin (PHT), levetiracetam (LEV), and PHT + LEV adjunctive treatments were examined on the cognitive functions of male rats.
Methods
Twenty-eight male Wistar rats (150–180 g) were randomized into 4 groups (N = 7). Groups I–IV received daily intraperitoneal administration of normal saline (0.2 ml), therapeutic doses of PHT (50 mg/kg), LEV (50 mg/kg) or sub-therapeutic dose of PHT (25 mg/kg) and LEV (25 mg/kg) combination, respectively, for 28 days. Thereafter, the animals were subjected to behavioral assessment and evaluation of the activities of acetylcholinesterase, lipid peroxidation, and lastly the morphological evaluation of the brain. Data were analyzed using descriptive and inferential statistics. The results were presented as mean ± SEM in graphs or tables, while the level of significance was taken at p < 0.05.
Results
Working & spatial memory, exploratory activities, and motor-coordination indices were significantly (p = 0.0099) impaired with a reduction in the frontal lobe and hippocampal weight following PHT and PHT + LEV adjunctive treatments. The frontal lobe and hippocampal activities of acetylcholinesterase increased significantly (p = 0.0437) following PHT and PHT + LEV adjunctive treatment. The concentrations of malondialdehyde increased significantly (p = 0.0473) in PHT, LEV, and PHT + LEV compared with the control. There was disorganization in the histoarchitectural profile with chromatolysis, hyalinization, and neural vacuolation in the pre-frontal cortex, hippocampus, and cerebellar tissue, especially in the PHT + LEV treated rats.
Conclusion
Impairment of cognitive functions following PHT and PHT + LEV adjunctive treatments may be attributable to the deregulation of cholinergic transmission and neurotoxicity.
1. Introduction
Unperturbed cognitive functions remain core components of maintaining a healthy, active, and independent lifestyle [Citation1]. In epilepsy, cognitive impairments are a potentially lifelong problem [Citation2]. This is reportedly attributable to seizure frequency, type/duration, and/or the type of antiepileptic drugs in use [Citation2,Citation3]. Older AEDs such as phenytoin, carbamazepine, valproic acid are some of the first line conventional AEDs, owing to some advantages such as lower cost, wide availability and long-term experience [Citation4], often time, they exhibit greater toxicity than newer antiepileptics such as gabapentin, vigabatrin, levetiracetam, etc. [Citation5,Citation6].
Phenytoin (Dilantin®) is an anticonvulsant medication that has been widely and effectively used in the treatment of epilepsy. This AED acts on the central nervous system by blocking the voltage-sensitive sodium ion channels, inhibiting neuronal firing with resultant modulatory effect on the bioelectric activity of the cell membrane in the brain [Citation7,Citation8]. However, it is reportedly implicated as a toxic agent with resultant nystagmus, diplopia, slurred speech, ataxia, incoordination, paraparesis, confusion, memory loss, dystonia, and altered mental status [Citation9,Citation10]. Levetiracetam is an AED that selectively inhibits high-voltage-activated calcium channels and reduces calcium release from intraneuronal stores. It also binds to a specific target in the brain, the synaptic vesicle protein 2A (SV2A), an integral membrane glycoprotein, which is involved in the control of vesicle fusion and exocytosis [Citation11]. This α-ethyl analog of the nootropic agent piracetam does not induce hepatic enzyme cytochrome p450 as part of its mechanism of action [Citation12,Citation13]; hence, it was approved as adjunctive therapy for primary generalized tonic-clonic seizures, myoclonic seizures of juvenile myoclonic epilepsy and partial-onset seizures with or without secondary generalization [Citation14].
Several pre-clinical experiments suggest synergistic efficacy between specific combinations of AEDs [Citation15–17], unfortunately, their consequential adverse effects are multi-systemic, e.g. pituitary-testicular dysfunction and ataxic movement among others [Citation5,Citation6]. Meanwhile, effective treatment of epilepsy depends on medication compliance across a lifetime [Citation7,Citation18]. The cognitive adverse events of AEDs usually abate after dose reduction or cessation [Citation19]. However, there is a paucity of experimental literature on the potential adverse effects of phenytoin – levetiracetam adjunctive treatment on the markers of cognitive functions, hence this study.
2. Methodology
2.1. Animals handling/care
The approval to carry out this experimental research was obtained from the Health Research Ethical Committee (HREC) of the Osun State University, Osogbo, Nigeria. Phenytoin and levetiracetam (Sigma, USA) were freshly prepared and dissolved in physiological solutions. A total of twenty-eight male Wistar rats (150–180 g; 12–14 weeks old) were randomized into 4 groups (N = 7). Female and any male rat whose weight and age did not fall within this range were excluded from the study. Group I (control) rat received normal saline (0.2 ml/day intraperitoneally [i.p]); group II received PHT (50 mg/kg/day i.p) [Citation19]; group III received LEV (50 mg/kg/day i.p) [Citation20]; while group IV received the adjunctive treatment of PHT (25 mg/kg/day i.p) with LEV (25 mg/kg/day i.p). The daily administration was done between 10 am and 11 am for 28 consecutive days. Thereafter, the rats were subjected to various behavioral tests of learning, memory, and motor coordination.
2.2. Behavioral studies
In this study, all the behavioral tests were carried out between 7th and 8th hour after drug administration under conditions of silence and illumination of 630 nm red LED vivarium light.
2.2.1. Step-down latency in inhibitory avoidance test
This was carried out on the 27th and 28th of the treatment. Each rat was placed on the shock-free zone and allowed to explore the chamber for 10 minutes. On stepping down on the grid floor, the animal received electric shock (20 V) through the grid floor. Two hours later, this procedure was repeated, the time taken for each rat to step down was measured in seconds. This is known as the step-down latency and this constitutes the training process. Following the training session, step-down latency by each rat was determined after 24 h of the training session. A prolongation or shortening of step-down latency was used as a parameter of learning and memory [Citation21].
2.2.2. Novel object recognition test
This was carried out on the 28th day of drug administration. This particular study was set to determine working and spatial memory in animals. Three days before the test sessions, the rats were exposed to two identical objects to acclimatize with for 5 min; thereafter, the rats were returned into their home cages. Thirty minutes later, the probe test was conducted, each rat was placed inside the box with one of the objects replaced by a novel one. The total time spent exploring the two objects was recorded by a blinded but experienced experimenter as a memory index (expressed as the ratio of total exploration time with both objects in the choice phase)[Citation22].
2.2.3. Open field test
The exploratory activity was investigated on the 28th day of drug administration. This was achieved by determining the total number of horizontal and vertical lines crossed by each rat in a wooden box measuring 1 m long × 1 m wide × 1 m high according to the method of Seibenhener et al. [Citation23]. In this study, exploratory activities of each rat were recorded over a period of 5 min by a video camera and scored by a blinded experimenter.
2.2.4. Beam balance transversal test
The beam balance test was carried out on the 28th day of drug administration to establish the effects of the treatment drugs on fine motor activities of the rat. Following a 10 minutes habituation period, animals were introduced to a beam transversal test according to the method of Hayashi et al. [Citation24], while the total time taken for each rat to reach the goal box was determined.
2.2.5. Accelerated rotarod test
This was carried out 21 hours after the last treatment, just before the sacrifice. The animal was initially habituated to the test room for 10 minutes before the commencement of the test. Motor-coordination of the rats was determined using accelerating Rota-rod (Ugo-Basile, Model No, 7750, Cosmerio, Italy, acceleration: 4–40 rpm in 5 minutes) according to a modified method of Hayashi et al. [Citation24].
2.3. Animal sacrifice, weight determination, and homogenization
Animals were decapitated 24 hours after the last treatment and the cranium was rapidly and carefully removed, while the brain was dissected. The frontal lobe, hippocampus and cerebellar weights were isolated, individually perfused in an isotonic solution, and then subjected to an electronic balance (Camry, China) for weight determination. The frontal lobe, hippocampus, and cerebellum of 5 rats from each group were homogenized in ice-cold medium containing 50 mM Tris/HCl and 300 mM sucrose at pH 7.4 to give a 10% (w/v) solution [Citation25]. The homogenate was spun in a cold centrifuge at 3000 rpm 10 min at 4°C. The supernatant was preserved at −20°C for the evaluation of markers of cholinergic neurotransmission, neuroinflammation, and lipid peroxidation
2.4. Biochemical studies
2.4.1. Estimation of Malonaldehyde concentration
The product of lipid peroxidation (malondialdehyde [MDA]) in the frontal lobe, hippocampus, and cerebellum was determined according to the method of Sally et al. [Citation26]. The principle is based on the spectrophotometric measurement of the color generated by the reaction of thiobarbituric acid (TBA) with MDA. Concentration of MDA: the absorbance coefficient of the MDA-TBA complex [absorbance coefficient E = 1.56 × 105 cm-1 M-1 (nmol/g wet tissue)].
2.4.2. Estimation of acetylcholinesterase activity
The supernatants from the tissue of the frontal lobe, hippocampus, and cerebellum were evaluated for the activities of acetylcholinesterase (AChE) according to the method of Habila et al. [Citation27]. Briefly, to 0.004 M buffered substrate, the tissue homogenate was added and incubated for 1 min at room temperature, followed by the addition of hydroxylamine hydrochloride, 4 N HCl, and 0.37 M FeCl3. After 1 min, the absorbance was recorded at 540 nm. Standards were prepared with acetylcholine chloride and the levels of AChE were expressed as μg/g tissue.
2.4.3. Histomorphology
Two rats from each group were perfused intracardially with 4% paraformaldehyde. Each brain was dissected and processed according to the conventional method as earlier described by Onaolapo et al. [Citation28]. The pre-frontal cortex, hippocampus, and cerebellum were sectioned, stained with hematoxylin and eosin (H and E). Digital photomicrographs of stained sections of sub-region cornu ammonis (CA 1) were taken at 400 magnifications.
2.4.4. Statistical analysis
Data were analyzed using descriptive and inferential statistics with the use of graph pad prism software. The control and test groups were compared using paired t-test, one-way analysis of variance (ANOVA), and Student-Newman-Keuls post hoc analysis where appropriate. The results were presented as mean ± SEM in graphs or tables and the level of significance was taken at p-value <0.05.
3. Result
3.1. Effects of phenytoin–levetiracetam combination on object recognition
In this study, there was significant decrease (p = 0.0366) in short term/working memory of animals treated with PHT + LEV adjunctive treatment (28.6 ± 3.41) compared with control (41.8 ± 4.29), while PHT (46.2 ± 4.84) and LEV treatment groups (41.0 ± 3.11) had no significant (p = 0.6444) difference ()
3.2. Effects of phenytoin–levetiracetam combination on step down latency
There was significant (p = 0.0071) decrease in the step-down latency of PHT + LEV adjunctive treated rats (167 ± 20.2) compared with the control (253.2 ± 16.13). However, neither PHT (243 ± 17.4) nor LEV (263 ± 18.6) posed a significant (p = 0.7154) effect on the long-term memory of the treated rats ().
3.3. Effects of phenytoin–levetiracetam combination on traversal period on the beambalance
In this study, chronic PHT treatment, and PHT + LEV adjunctive treatment increased (p = 0.0001) the traversal period (33.0 ± 2.74) and (40.0 ± 4.00), respectively, of male rats compared with the control (13.4 ± 2.01), while LEV treatment had no significant (p = 0.1263) effect ().
3.4. Effects of phenytoin–levetiracetam combination on exploratory activities
There was a significant (p = 0.0469) decrease in the exploratory activities (23.4 ± 3.33) of PHT + LEV treated rat compared with the control, while none of PHT, and LEV treatment altered the exploratory activity significantly (p = 0.0613) ().
3.5. Effects of phenytoin–levetiracetam combination on motor coordination in rotarod test
In this study, latency on rota rod decreased significantly (p = 0.0001) after long-term administration of PHT (45.0 ± 4.74), and PHT + LEV (15.0 ± 4.23) treatments compared with the control (69.0 ± 5.33), while LEV alone treatment had no significant (p = 0.9192) effect ().
3.6. Effects of phenytoin–levetiracetam combination on the weight of the brain, frontal lobe, hippocampus, and cerebellum of male Wistar rats
Neither chronic PHT (1.61 ± 0.08) nor LEV (1.69 ± 0.10) treatment had significant (p = 0.4898) effect on the bran weight relative to control (1.75 ± 0.04). However, there was a significant (p = 0.0006) decrease in the brain weight of rats treated with PHT + LEV ().
Table 1. Effects of phenytoin + levetiracetam adjunctive treatment on the weight of the frontal lobe, hippocampus, and cerebellum in male Wistar rat
In this study, PHT (0.203 ± 0.0145 g) treatment and PHT + LEV combination treatment (0.20 ± 0.01 g) decreased the weight of the frontal lobe significantly (p = 0.0150) compared with the control (0.27 ± 0.01 g) ().
The hippocampal weight decreased significantly (p = 0.0006) following PHT (0.150 ± 0.015 g) and PHT + LEV (0.103 ± 0.032 g) treatments compared with the control (0.400 ± 0.027 g). However, the hippocampal weight of LEV treatment group had no significant difference (p = 0.005) ().
Concerning the cerebellar weight, none of the treatment groups PHT (0.290 ± 0.023 g), LEV (0.340 ± 0.021 g) and PHT + LEV (0.340 ± 0.038 g) posed a significant (p = 0.4411) weight change compared with the control (0.340 ± 0.010) ().
3.7. Effects of phenytoin–levetiracetam combination treatment on the acetylcholinesterase activities of the frontal lobe, and hippocampus of male Wistar rat
In this study, significant (p = 0.0061) increase in the activities of AChE observed in the PHT (89.4 ± 10.10 U/l) and PHT + LEV (175 ± 3.96 U/l) treated groups compared with control (129 ± 7.55 U/l), while LEV treatment had no significant (p = 0.7076) effect ().
Table 2. Effects of phenytoin–levetiracetam combination on the acetylcholinesterase activities in the frontal lobe and hippocampus
There was a significant increase in the hippocampal activities of acetylcholinesterase following PHT and PHT + LEV adjunctive treatment (148 ± 1.48 U/l) and (175 ± 3.96 U/l), respectively, compared with the control (126 ± 5.16 U/l), while LEV posed no significant (p = 0.3423) effect ().
Treatment with PHT (81.82 ± 4.63 U/l) or LEV (74.09 ± 8.60 U/L) had no significant alteration in the activities of acetylcholinesterase. However, PHT + LEV adjunctive treatment increased AChE activities significantly (p = 0.0002) in the cerebellum of male Wistar rats ()
3.8. Effects of phenytoin-levetiracetam adjunctive treatment on the malondialdehyde concentration of frontal lobe, hippocampus, and cerebellum of male Wistar rats
The product of lipid peroxidation, malondialdehyde concentration increased significantly (p = 0.0001) in the frontal lobe of PHT (4.71 ± 0.11 mM) and PHT + LEV (4.84 ± 0.042 mM) treatment groups compared with control (3.89 ± 0.08 mM) ().
Table 3. Effects of phenytoin–levetiracetam combination treatment on the level of the frontal lobe, hippocampus and cerebellar malondialdehyde in male Wistar rats
In the hippocampal tissue, however, there was significant (p = 0.0007) increase in the concentration of MDA following PHT (7.44 ± 0.56 mM), LEV (6.08 ± 0.344 mM), and PHT + LEV (14.0 ± 1.98 mM) adjunctive treatment compared with the control ().
In this study, none of the PHT (3.35 ± 0.03 mM), LEV (3.32 ± 0.29 mM), and PHT + LEV treatment groups had a significant effect (p = 0.1571) on the cerebellar concentration of MDA compared with control (3.31 ± 0.18 mM) ().
3.9. The histomorphology of pre-frontal cortex following PHT, LEV and PHT+ LEV adjunctive treatment in male Wistar rats
The prefrontal cortex of the normal saline-treated rat showed normal laminae and the neuronal cells appear normal (blue arrow), the capillaries seen were normal and the stroma also appeared normal (slender arrow) ().
Figure 6. The histomorphology of pre-frontal cortex following PHT, LEV and PHT+ LEV adjunctive treatment in male Wistar rats
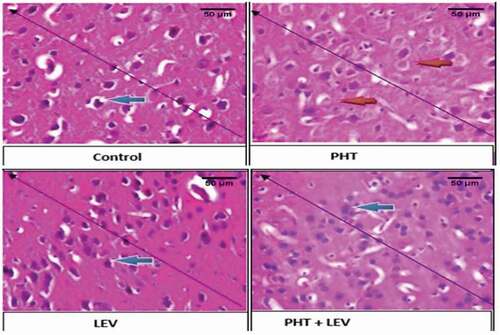
The PFC of the PHT treated rat showed moderately normal laminae, while some of the neuronal cells were presented with a less severe chromatolysis and hyalinization (red arrow), the capillaries are normal and the stroma also appears normal (slender arrow) ().
The PFC of the LEV treated rat showed normal laminae and the neuronal cells appear normal (blue arrow), the capillaries seen are normal and the stroma appears normal as well (slender arrow) ().
In the PHT + LEV treated rats, there were distorted laminae, the neuronal cells appear normal (blue arrow), while the capillaries are unaltered (slender arrow) ().
3.10. The histomorphology of precentral cortex following PHT, LEV, and PHT + LEV adjunctive treatment in male Wistar rats
The precentral cortex of the normal saline-treated rat showed normal laminae and the neuronal cells appear normal (blue arrow), the capillaries seen were normal and the stroma also appeared normal (slender arrow) ().
Figure 7. The histomorphology of hippocampus following PHT, LEV and PHT+ LEV adjunctive treatment in male Wistar rats
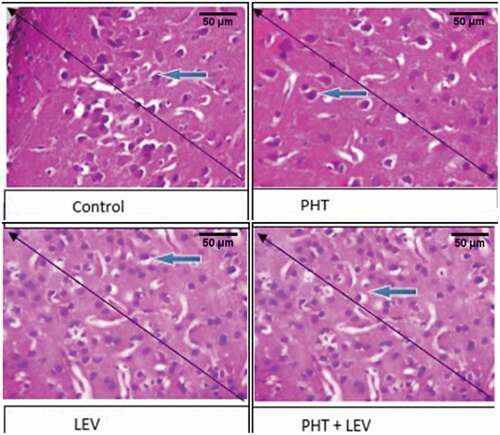
The PFC of the PHT treated rat showed moderately normal laminae, while some of the neuronal cells were presented with a less severe chromatolysis and hyalinization (red arrow), the capillaries are normal and the stroma also appears normal (slender arrow) ().
The PFC of the LEV treated rat showed normal laminae and the neuronal cells appear normal (blue arrow), the capillaries seen are normal and the stroma appears normal as well (slender arrow) ().
3.11. The histomorphology of hippocampus following PHT, LEV, and PHT + LEV adjunctive treatment in male Wistar rats
In this study, the CA 1 in the hippocampus of the PHT, LEV, and PHT + LEV adjunctive treatment showed neuronal vacuolation/atrophy (red arrow) relative to the control (white arrow). This degeneration was most severe in the PHT + LEV adjunctive treated representative rat but severe in the LEV treated group ().
Figure 8. The histomorphology of cerebellum following PHT, LEV and PHT+ LEV adjunctive treatment in male Wistar rats
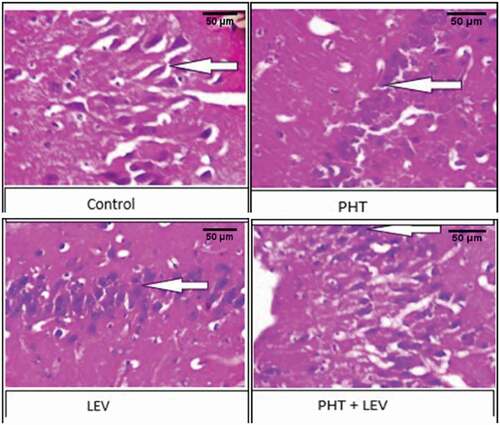
3.12. The histomorphology of cerebellum following PHT, LEV, and PHT + LEV adjunctive treatment in male Wistar rats
In the PHT treated rat, there was normal cerebellar formed folia, the molecular cell layer appears normal (gray arrow), the Purkinje cell layer showed normal Purkinje cells (gray arrow) and granular layer appear normal (red arrow) ().
Figure 9. The histomorphology of cerebellum following PHT, LEV and PHT+ LEV adjunctive treatment in male Wistar rats
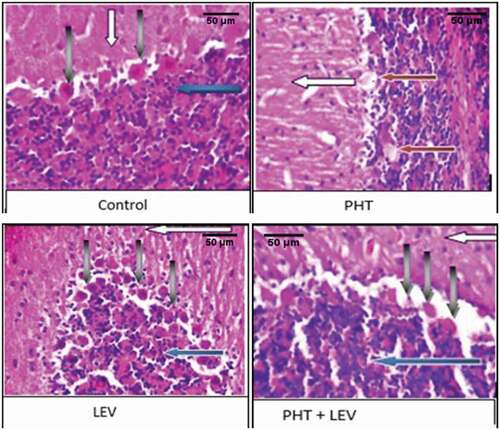
The cerebellar cortex of the LEV treated rat showed normally formed folia, the molecular cell layer appeared normal (white arrow), the Purkinje cell layer showed mild to moderate Purkinje cell hyperplasia (gray arrow), and granular layer appears normal (red arrow) ().
However, the section of the cerebellum in the PHT + LEV treated rat showed normal folia, the molecular cell layer appears normal (white arrow) the Purkinje cell layer showed eosinophilic and hyalinized Purkinje cells (gray arrow), and granular layer appears normal (red arrow) ().
4. Discussion
Chronic treatment with adjunctive AEDs with varying mechanisms of action has become a norm, especially in the management of refractory seizures. Although there are several reports on the effects of monotherapy of AED on cognitive functions [Citation29–31], there is a paucity of literature on the markers of cognitive functions following adjunctive treatment, which could have shed more light on the pathophysiology of its potential adverse effects. Findings from this study suggest that treatments with a conventional AED, PHT, and PHT + LEV favors smooth motor performance in male Wistar rats. Findings from both clinical and experimental investigations have revealed the choreoathetosis and ataxic movements in monotherapy and polypharmacy of AEDs Citation31,Citation32]. A significant attenuation in the exploratory activities in the PHT + LEV adjunctive treatment group suggests synergistic effects of the two drugs in the induction of the nerve endings of the following voltage – and ligand-gated ion channels: the α subunits of voltage-gated sodium channels, T-type and α2-δ subunits of voltage-gated calcium Ca2+ channels, A- or M-type voltage-gated K+ channel, the ϒ- aminobutyric acid (GABA) receptor channel complex and ionotropic glutamatergic receptors [Citation33,Citation34] with resultant sedative effects due to the binding and stimulation of striatal GABAA receptors [Citation34].
A significant decrease in absolute or relative organ weight could serve as a pointer to the toxicity of such agents [Citation35]. A significant decrease in the weight of the frontal lobe and hippocampus after a chronic PHT or PHT + LEV adjunctive treatment could be linked to the alteration in the cognitive functions observed in this study. It would be recalled that the frontal lobe, specifically the precentral gyrus is the brain region concerned with the initiation and control of movement activities (i.e. excito-motor- area 4 and suppressor area 4S) [Citation36], while the pre-frontal cortex forms the center for the higher functions like emotion, learning, short-term memory and social behavior [Citation37,Citation38]. Moreover, prefrontal cortex is the center for planned actions and seat of intelligence [Citation39]. Therefore, a reduction in the weight of the frontal lobe in this study is not unrelated to awkward movement and poor working memory recorded following the PHT and PHT + LEV adjunctive treatment.
Step-down latency and novel object recognition tests are strong markers of hippocampal-dependent memory [Citation38,Citation40]. Significant memory impairment observed in the PHT and PHT +LEV treated groups is traceable to an increase in the activity of hippocampal and frontal lobe acetylcholinesterase. Acetylcholine has multiple mechanisms by which it can modulate hippocampal memory [Citation41]. However, previous reports on the anticonvulsant effects of phenytoin have shown the attenuation of acetylcholine in various synapses [Citation42]. It is therefore inferred that an increase in the activity of acetylcholinesterase in this study is not unrelated to the degradation of acetylcholine with resultant impairment of central cholinergic transmission.
Significant increase in the accumulation of end product of lipid peroxidation, malondialdehyde following PHT, LEV, and PHT + LEV treatment is suggestive of the oxidative stress impact of the drugs either as a single or adjunctive treatment, but the LEV treatment was better tolerated. This present finding corroborates the previous report of Morimoto et al. [Citation43] and Mahmoud et al. [Citation44] that lipid membrane peroxidation in epilepsy treatment is not unrelated to the antiepileptic drugs. However, our finding is in contrast to the report of Menon et al. [Citation45] that oxidative stress in patients with epilepsy is independent of antiepileptic drugs. This difference may be due to the variation in study design. Findings from this study are consistent with the previous report of Milligan et al., [Citation46] that newer anticonvulsant drug poses lesser adverse effect compared with the conventional AEDs, while adjunctive treatment or polytherapy remains the last option when there is a refractory epileptic seizure.
The chromatolysis and hyalinization observed in the photomicrograph of the PFC and cerebellum suggest the potential toxicity of PHT and PHT + LEV treatment which is probably induced by lipid peroxidation of the tissue membrane. Besides, the disorganization of the histoarchitectural profile of CA 1 in the hippocampus following PHT, LEV, and PHT + LEV adjunctive treatment is an assertion that none of the AEDs is without cytotoxicity in the brain.
In conclusion, chronic administration of PHT, LEV, or PHT + LEV adjunctive treatment may induce alteration in the cognitive function through neurotoxicity and deregulation of cholinergic transmission with the most severe effects on PHT + LEV adjunctive treatment, while LEV alone treatment posed fewer adverse effects.
Grant information
The authors declared that this research was self-sponsored and conducted in the absence of any commercial or financial relationships that could be construed as a potential conflict of interest.
Disclosure statement
No potential conflict of interest was reported by the author(s).
References
- Bixter MT, Blocker KA, Rogers WA. Enhancing the social engagement of older adults through technology. Author links open overlay panels. Aging, Technology, and Health; 2018. p. 179–214.
- Mula M. Cognitive dysfunction in patients with epilepsy: focus on clinical variables. Future Neurol. 2015;10(1):41–48.
- Landi S, Petrucco L, Sicca F, et al. Transient cognitive impairment in epilepsy. Front Mol Neurosci. 2019;11:458.
- Eddy CM, Rickards HE, Cavanna AE. The cognitive impact of antiepileptic drugs. Ther Adv Neurol Disord. 2011;4(6):385–407.
- Akinsomisoye OS, Owolabi AR, Adeyeye OB, et al. Effects of vigabatrin, carbamazepine, or its combination on the pituitary-gonadal axis in male Wistar rats. Res J Health Sci. 2017;5(4):194–203.
- Osuntokun OS, Adedokun KI, Oladokun OO, et al. Carbamazepine alone induced more severe ataxic movement and cerebellar toxicity than gabapentin or carbamazepine-gabapentin. UNIOSUN J Sci. 2018;3(2):122–131.
- Prunetti P, Perucca E. New and forthcoming anti-epileptic drugs. Curr Opin Neurol. 2011;24(2):159–164.
- Bialer M. Why are antiepileptic drugs used for nonepileptic conditions? Epilepsia. 2012;53(7):26–33.
- Lowry JA, Vandover JC, DeGreeff J, et al. Unusual presentation of iatrogenic phenytoin toxicity in a newborn. J Med Toxicol. 2005;1(1):26–29.
- Gupta V, Yadav TP, Yadav A. Phenytoin toxicity presenting as acute meningoencephalitis in children. Neurol India. 2011;59(1):66–67.
- Lukyanetz EA, Shkryl VM, Kostyuk PG. Selective blockade of N-type calcium channels by levetiracetam. Epilepsia. 2002;43(1):9–18.
- Swaroop HS, Ananya C, Nithin K, et al. Levetiracetam: a Review of its use in the treatment of epilepsy. Int J Med Biomed Res. 2013;2(3):166–172.
- Rachamallu V, Song MM, Reed JM, et al. Levetiracetam-induced transaminitis in a young male with traumatic brain injury. Oxf Med Case Reports. 2017;2017(11):omx067.
- Brown C. Pharmacological management of epilepsy. Prog Neurol Psychiatry. 2018;20(2):27–34.
- Matagne AC, Baltes E, Coupez R. Levetiracetam enhances markedly the seizure suppression of other antiepileptic drugs in audiogenic susceptible mice. Epilepsia. 2001;42:82.
- Czubak A, Nowakowska E, Kus K, et al. Effect of gabapentin on cognitive processes in rats not exposed and exposed to tobacco smoke during fetal life. Hum Exp Toxicol. 2001;27(12):883–894.
- Olaibi OK, Osuntokun OS, Ijomone OM. Effects of chronic administration of gabapentin and carbamazepine on the histomorphology of the hippocampus and striatum. Ann Neurosci. 2014;21(2):57–61.
- Witt JA, Helmstaedter C. Monitoring the cognitive effects of antiepileptic pharmacotherapy – approaching the individual patient. Epilepsy Behav. 2013;26(3):450–456.
- Soysal H, Unur E, Duzler A, et al. Effects of intraperitoneal administration of the phenytoin on the skeletal system of rat fetus. Seizure. 2011;20(3):187–193.
- Talos D, Chang M, Kosaras B. Antiepileptic effects of levetiracetam in a rodent neonatal seizure model. Pediatr Res. 2013;73(1):24–30.
- Brioni JD, Hock FJ, Mcgaugh JL. Drug effects on learning and memory. In: Vogel HG, Vogel WH, editors. Drug discovery and evaluation. 2nd ed. Philadephia: Springer; 2002. p. 619–622.
- Ogundele OM, Adeniyi PA, Ajonijeb DC, et al. Motor and memory function in rat models of cyanide toxicity and vascular occlusion induced ischemic injury. Pathophysiology. 2014;21(3):191–198.
- Seibenhener ML, Wooten MC. Use of the open field maze to measure locomotor and anxiety-like behavior in mice. JoVE. 2015;6 (96):e52434.
- Hayashi K, Furuya A, Sakamaki Y, et al. The brain-specific RasGEF very-KIND is required for normal dendritic growth in cerebellar granule cells and proper motor coordination. PLoS One. 2017;12(3):e0173175. .
- Tsakiris S, Schulpis KH, Marinou K, et al. Protective effect of L-cysteine and glutathione on the modulated suckling rat brain Na+, K+-ATPase and Mg2+-ATPase activities induced by the in vitro galactosemia. Pharmacol Res. 2004;49(5):475–479.
- Sally AE, Gehad AAJ, Dalia OS. Alleviation of haloperidol-induced oxidative stress in rats: effects of sucrose vs grape seed extract. Bull Faculty Pharm Cairo Univ. 2015;53(1):29–35.
- Habila N, Inuwa HM, Aimola IA, et al. Correlation of acetylcholinesterase activity in the brain and blood of Wistar rats acutely infected with Trypanosoma Congolense. J Acute Dis. 2012;1(1):26–30.
- Onaolapo OJ, Onaolapo AY, Akanmu MA, et al. Evidence of alterations in brain structure and antioxidant status following ‘low dose’ monosodium glutamate ingestion. Pathophysiology. 2016;23(3):147–156.
- Czuczwar SJ, Borowicz KK. Polytherapy in epilepsy: the experimental evidence. Epilepsy Res. 2002;52(1):15–23.
- Forcelli PA, Kozlowski R, Snyder C, et al. Effects of neonatal antiepileptic drug exposure on cognitive, emotional, and motor function in adult rats. J Pharmacol Exp Ther. 2012;340(3):558–566.
- Zimcikova E, Simko J, Karesova I, et al. Behavioral effects of antiepileptic drugs in rats: are the effects on mood and behavior detectable in an open-field test? Seizure. 2017;52:35–40.
- Shanmugam S, Daniel L, Ahamed J. A meta-analysis of antiepileptic drugs induced choreoathetosis in pediatric patients. Int J Med Rev Case Rep. 2017;1(3):49–52.
- Chindo BA, Adzu B, Gamaniel KS. Antiepileptic drug targets: an update on ion channels, epileptology - the modern state of science, Vladimir V. Kalinin, Intech Open; 2016. DOI:https://doi.org/10.5772/64455.
- Schipper S, Aalbers MW, Rijkers K, et al. Tonic GABAA receptors as potential target for the treatment of temporal lobe epilepsy. Mol Neurobiol. 2016;53(8):5252–5265.
- Osuntokun OS, Olayiwola G, Oladele A, et al. Chronic administration of gabapentin and a gabapentin-carbamazepine combination reversibly suppress testicular function in male Wistar rats (Rattus norvegicus). Pathophysiology. 2017;24(2):63–69.
- Nachev P, Wydell H, O’Neill K, et al. The role of pre-supplementary motor areas in the control of the action. Neuroimage. 2007;36:3.
- Sakurai Y, Furukawa E, Kurihara M, et al. Frontal phonological agraphia and acalculia with impaired verbal short-term memory due to left inferior precentral gyrus lesion. Case Rep Neurol. 2018;10(1):72–82.
- Arora A, Mehta AK, Sharma KK, et al. Effects of carbamazepine and lamotrigine on cognitive function and oxidative stress in the brain during chemical epileptogenesis in rats. Basic Clin Pharmacol Toxicol. 2009;106(5):372–377.
- Sembulingam K, Sembulingam P. Essential of medical physiology. Jaypee Brothers Medical Publishers(P) Ltd. 2012;12:884–889.
- Kersten M, Rabbe T, Blome R, et al. Novel object recognition in rats with NMDAR dysfunction in CA1 after stereotactic injection of anti-NMDAR encephalitis cerebrospinal fluid. Front Neurol. 2019;10(586):1–11.
- Maurer SV, Williams CL. The cholinergic system modulates memory and hippocampal plasticity via its interactions with non-neuronal cells. Front Immunol. 2017;8:1489.
- Bookheimer SY. Precentral Gyrus. In: Volkmar FR, editor. Encyclopedia of autism spectrum disorders. New York, NY: Springer; 2013. DOI:https://doi.org/10.1007/978-1-4419-1698-3_203
- Morimoto M, Hashimoto T, Kitaoka T, et al. Impact of oxidative stress and newer antiepileptic drugs on the albumin and cortisol value in severe motor and intellectual disabilities with epilepsy. J Clin Med Res. 2018;10(2):137–145.
- Mahmoud AT, Tawfik MA, Abd-El-Aziz HAD. A study of DNZ damage in epileptic treated with valproic acid or carbamazepine. Menoufia Med J. 2019;32(3):1078–1082.
- Menon B, Ramalingam K, Kumar RV. Oxidative stress in patients with epilepsy is independent of antiepileptic drugs. Seizure. 2012;21(10):780–784.
- Milligan TA, Hurwitz S, Bromfield EB. Efficacy and tolerability of levetiracetam versus phenytoin after supratentorial neurosurgery. Neurology. 2008;71(9):665–669.