ABSTRACT
Cephalosporins are the first-line therapy antibiotics used in the treatment of gram-negative bacterial infections. However, high prevalence of cephalosporins resistance in Klebsiella pneumoniae and Escherichia coli has been reported worldwide. Studies conducted in Uganda reported high incidences of cephalosporin resistance (CR). Successive studies at Mulago National Referral Hospital indicated a decline in the resistance levels pointing to the need for regular antibiotic resistance surveillance. Therefore, this study carried out molecular characterization of CR determinants in E. coli and K. pneumoniae isolated from patients attending Kampala International University Teaching Hospital (KIU-TH). A retrospective study using E. coli and K. pnuemoniae samples previously obtained from surgical wounds and urinary tract infections among patients treated at KIU-TH between September 2016 and August 2018 was conducted. Biochemical assays were used to confirm the identity of the samples. Combined disc and boronic acid assays were used to determine the cephalosporine resistance profile of the isolates. Multiplex PCR amplification was used to characterize the extended spectrum beta-lactmase (ESBL) encoding genes. The study revealed that E. coli (130/81.2%) isolates were more predominant than K. pneumoniae (30/18.8%) among the archived samples. K. pneumoniae showed the highest phenotypic resistance with a mean prevalence of 90.6% but comparable to that of E. coli (89.3%). Of the 160 isolates screened, 105 (65.6%) were ESBL producers. Multiplex PCR revealed that the most predominant ESBL encoding gene was blaSHV at a prevalence of 42.0%, followed by blaTEM at 27.3%, blaCTX-M at 22.4% and blaCTX-M-15 at 8.4%. The incidence of phenotypic resistance and distribution of ESBL genes were significantly higher in patients of Ishaka division. Our study reports a high prevalence of cephalosporin-resistant E. coli and K. pnuemoniae isolated from patients attending KIU-TH and highlights the need for routine screening of antimicrobial resistance in health-care facilities so as to guide clinicians on the rational prescription of antibiotics.
List of abbreviations: ATCC: American Type Culture Collection; ESBL: extended spectrum beta-lactmases; AmpC: aminopenicillin cephalosporinase; CTX-M: cephotaxime (M-first detected in Munich) hydrolyzing capabilities; CTX-M-U: cephotaxime hydrolyzing capabilities gene Universal primer; SHV: sulfhydryl variables (variant-2); CMY-2: cephamycins (variant-2); TEM: temoneira; ACT-1: AmpC type (variant-1); Bla: Beta lactam; DNA: deoxyribonucleic acid; PCR: Polymerase chain reaction; MNRH: Mulago National Referral Hospital; MRRH: Mbarara Regional Referral Hospital; KRRH: Kabale Regional Referral Hospital (KRRH); KIU-TH: Kampala International University Teaching Hospital; CDL: Central Diagnostic Laboratory; CoVAB: College of Veterinary Medicine, Animal Resources and Biosecurity; CR: cephalosporin resistance
1. Introduction
Cephalosporins form a relatively large group of antibiotics with a β-lactam ring. This class of antibiotics is bactericidal against gram-negative bacteria. Cephalosporins are classified into first, second, third, fourth, and fifth generations [Citation1]. The third generation cephalosporins are the most widely used in the treatment of gram-negative bacterial infections. They include cefotaxime, ceftriaxone, ceftazidime, cefixime, ceftizoxime, and cefoperazone [Citation2]. Cephalosporin resistance (CR) in Enterobacteriaceae, most importantly, Escherichia coli and Klebsiella pneumoniae, which are the leading causes of hospital- and community-acquired bacterial infections, has been reported worldwide [Citation3–5]. Antibiotic resistance to beta-lactam antibiotics arises through a number of mechanisms, which include: (a) inactivation of antibiotics through hydrolyzing the beta-lactam ring by bacterial enzymes encoded by resistance genes located on chromosomes and plasmids, (b) modification and downregulation of porin expression that leads to the decrease in permeability of the outer membrane to cephalosporins; (c) flushing out of cephalosporins by efflux pumps; and (d) acquisition of a new penicillin-binding protein (PBP) or modification of the PBP, thereby reducing the affinity of PBP component to cephalosporins [Citation6,Citation7]. The mode of resistance exhibited by most gram-negative bacteria to cephalosporins is mainly attributed to bacterial enzymes called beta-lactamases, which are encoded by different genes that include TEM, SHV, CTX, ACT-1, CMY-2, CMY-4, and DHA-1 [Citation6–8].
A study in Uganda reported CR prevalence of 79.2% and 92.3% in E. coli and K. pneumoniae isolates, respectively, at Mulago National Referral Hospital (MNRH) [Citation9]. Another study at Mbarara Regional Referral Hospital (MRRH) revealed that the most common extended spectrum beta-lactmase (ESBL) producers were E. coli at 34% and K. pneumonia at 12%, where the ESBLs resistant determinants characterized included blaCTX-M (70%), blaSHV (34%), mixed blaCTX-M/blaSHV (7.4%), blaCTX-M/blaTEM (5.8%), blaSHV/blaTEM (0.8%), and blaCTX-M/blaSHV/blaTEM (3.8%) [Citation10].
This variation in the CR prevalence and resistance genes reported in Uganda point to the need for antibiotic resistance surveillance at several localities. This is because drug resistance within the pathogens is an evolving process that requires routine surveillance and monitoring in order to provide physicians with the current status of antibiotic resistance for rational prescription of drugs [Citation11]. Furthermore, hospital-based antibiotics resistance stewardship dictates antibiotics prescription in a given hospital and is determined by the prevailing resistance to a given class of antibiotics [Citation11,Citation12]. Thus, this study focused on prevalence and genotypic characterization of CR genes in E. coli and K. pneumoniae isolated from patients attending Kampala International University Teaching Hospital (KIU-TH) in Uganda.
2. Materials and methods
2.1. Study design and bacterial samples
We carried out a retrospective study using E. coli and K. pneumoniae samples previously obtained from surgical wounds and UTIs among patients of Ishaka, Bushenyi, and Nyakabirizi divisions treated at KIU-TH between September 2016 and August 2018. Archived resistant E. coli and K. pneumoniae isolates were collected and recovered from the Microbiology Laboratory, KIU-TH, and transferred to the Central Diagnostic Laboratory, Makerere University, for identity confirmation by biochemical assays. CR profile was determined using the disc diffusion method and, thereafter, the characterization of CR genes using multiplex PCR.
3. Inclusion and exclusion criteria
Archived samples previously isolated from urine and surgical wounds of in- and out-patients at KIU-TH were included. A total of 180 samples were included. Samples that were not isolated from patients of Bushenyi-Ishaka Municipality (Ishaka, Bushenyi, and Nyakabirizi divisions) and those that could not be confirmed as E. coli or K. pnuemoniae or those where no bacterial growth was observed were excluded from subsequent analysis.
4. Antimicrobial susceptibility test
E. coli and K. pneumoniae identity was confirmed using biochemical tests as previously described. Triple sugar iron test that detects glucose, lactose, and sucrose fermenters [Citation13,Citation14], indole test [Citation14] and citrate utilization test [Citation13]), urease test [Citation14]), and methyl red and Voges Proskaue tests [Citation14] were conducted. The susceptibility tests were performed using the disc diffusion method according to the standard guidelines [Citation15]. The isolates were subjected to antibiotic susceptibility test on Muller–Hinton agar using Ampicillin 25 µg; amoxicillin/clavulanic acid 20/10 µg; ceftazidime 30 µg; cefuroxime (CXM) 30 μg; temocillin (TEM) (30 μg); piperacillin-tazobactum (TPZ) 110 μg; cefoxitin (FOX) 30 μg; ceftizoxime (CTX) 30 μg; ceftazidime (CAZ) 30 μg; ceftriaxone (CRO) 30 μg; and cefixime (CFM) 30 μg. K. pneumoniae American Type Culture Collection (ATCC) 700603 and E. coli ATCC 25922 were used as positive and negative controls, respectively [Citation16,Citation17].
5. Detection of ESBLs and aminopenicillin cephalosporinases (AmpCs)
To distinguish between ESBLs and AmpCs producers, the phenotypic confirmatory disc diffusion test was performed as follows. The Mueller Hinton Agar (MHA) was inoculated with standard inoculums (0.5 McFarland) of the test isolate. Antibiotic discs containing a mixture of ceftazidime and clavulanic acid at a concentration of 30 µg/10ug and ceftazidime alone was used to taste for the level of resistance. A larger inhibitory zone diameter (>5 mm) in the presence of clavulanic acid than with ceftazidime alone indicated the presence of ESBLs [Citation18]. The phenotypic detection of AmpC β-lactamases was achieved by using boronic acid disc test. Discs containing boronic acid were prepared as follows. One hundred twenty milligrams of phenyl boronic acid (benzeneboronic acid) were dissolved in 3 mL of dimethyl sulfoxide. Then, 3 mL of sterile distilled water was added to this solution. Twenty microliters of this solution was dispensed onto the discs containing 30 µg of cefotetan discs and allowed to dry for 30 minutes and used immediately. The boronic acid test was performed by inoculating MHA with standard inoculums of E. coli and K. pneumoniae followed by placing a disc containing 30 µg of cefotetan and a disc containing 30 µg of cefotetan and 400 µg of boronic acid solution onto the agar. The inoculated plates were incubated overnight at 35°C. Isolates that demonstrated zones of inhibition around the disc containing cefotetan and boronic acid ≥5 mm were considered AmpC producers. Isolates that were positive for phenotypic confirmatory disc diffusion test and boronic acid test were considered to express both ESBLs and AmpCs [Citation18–20].
6. Molecular detection of ESBLs encoding genes
Genomic DNA was extracted using the Qiagen DNA extraction kit following the manufacturer’s protocol. Multiplex PCR was performed as previously described by Voets et al. [Citation21] and Dallenne et al. [Citation22], with modifications to enable the concurrent amplification of all the target gene variants using blaTEM, blaSHV, blaCTX-M, and blaCTX-M-15 general primers (). Briefly, a final PCR volume of 40 µL containing 5 µL of 0.5 µg DNA template, 1 µL of 1 U Taq polymerase, 1 µl of 200 µM dNTPs, 8 µL of all primer combinations (2 μl/primer pair), and 25 µL PCR buffer (2 mM MgCl2,100 mM Tris-HCl,500 mM KCl pH 8.3) was constituted. The PCR program consisted of an initial denaturation at 94°C for 5 minutes followed by 35 cycles at 94°C for 1 minute, 60°C for 30 seconds, 72°C for 1 minute, and a final extension at 72°C for 10 minutes. PCR products were run on a 1.5% agarose gel, stained using ethidium bromide, visualized and imaged under UV light using Image analyzer. The size of bands was used to identify the gene responsible for resistance () [Citation23]. K. pneumoniae 7881, K. pneumoniae ATCC 700603, and E. coli ATCC 35218 containing blaSHV, blaCTX-M, and blaTEM genes were used as positive controls, whereas E. coli ATCC 25922 was used as the negative control.
Table 1. Cephalosporin resistance gene, gene primer sequences, amplicon size, and their prevalence in E. coli and K. pneumoniae isolated from patients of Ishaka-Bushenyi Municipality
7. Data analysis
Data analysis was done using OriginPro version 8.5. Comparisons of CR between E. coli and K. pneumoniae, distribution of phenotypic resistance, and genetic determinants of resistance among communities (division of the patients) were performed using one-way analysis of variance (ANOVA) followed by Tukey’s multiple comparisons test. A P-value of ≤0.05 indicated significant statistical variance.
8. Results
8.1. CR profile
Of the 180 isolates obtained from the archives, 130 (81.2%) were confirmed to be E. coli, 30 (18.8%) K. pneumoniae, and 13 (7.2%) isolates were neither E. coli nor K. pneumoniae, while 7 (3.9%) isolates exhibited no growth. Bacterial isolates were predominantly obtained from urine (96/60%), . All the 160 isolates were at least resistant to one of the antibiotics. Resistance to Cefotaxime was the highest but not significantly different from the resistance registered by ceftriaxone, ceftazidime and cefixime. K. pneumoniae and E. coli exhibited statistically similar phenotypic resistance, .
Table 2. Resistance profiles of E. coli and K. pneumoniae isolated from Ishaka, Bushenyi, and Nyakabirizi
Table 3. Bacteria isolates obtained from different clinical specimens
8.2. Prevalence of ESBLs and AmpCs producers
Phenotypic confirmatory disc diffusion and boronic acid disc assays revealed that out of 160 isolates screened, 105 (65.6%) were ESBLs producers where 56.2% (69 E. coli and 21 K. pneumoniae) were positive for ESBLs, while 9.4% (12 E. coli and 3 K. pneumoniae) harbored both ESBLs and AmpCs. None of the isolates was positive for only AmpCs while 55 (34.4%) were negative for both ESBLs and AmpCs.
8.3. Distribution of ESBLs encoding genes
The 105 isolates that stood to be ESBL producers were typed by multiplex PCR and recorded a total of 143 genes (). The blaSHV-1-like gene recorded the highest prevalence (35%), followed by blaTEM (27.3%), blaCTX-M (22.4%), blaCTX-M-15 (8.4%), and then blaSHV-2-like (7.0%) (). Furthermore, multiple Tukey comparison revealed that the prevalence of CR genes in E. coli and K. pneumoniae was substantially dissimilar.
Figure 1. Multiplex PCR amplification of the ESBLs encoding genes. 1000 bp ladder (1Kb)-Eurofins genomics, Positive control 1 (+C1)-E. coli ATCC 35218, Positive control 2 (+C2)-K. pneumoniae 7881, Positive control 3 (+C3) K. pneumoniae ATCC 700603; Amplicon level 1 represents CTXM-15 gene (approx. 995bp), level 2: TEM gene (approx. 856bp), level 3:SHV-2 gene (approx. 713bp), level 4:CTXM-U gene (approx. 596bp, level 5:SHV-1 (approx. 230bp) and E1–E10 representative E. coli isolates
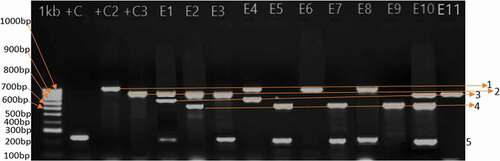
Of the 105 isolates, 35 (33.3%) contained more than one ESBL genes; eight (7.6%) E. coli and 3(2.8%) K. pneumoniae isolates coharboured blaSHV-1-like and blaTEM, blaSHV-1-like and blaCTX-M resided in 6 (5.7%) E.coli and 2 (1.9%) K. pneumoniae, blaCTX-M and blaTEM co-occurred in 2 (1.9%) E. coli and 3 (2.8%) K. pneumoniae, blaCTX-M-15 and blaTEM coexisted in 2 (1.9%) E. coli, blaSHV-1-like and blaCTX-M-15 co-occurred in 3 (2.8%) E. coli, blaCTX-M and blaCTX-M-15 3 (2.8%) were co-present in E. coli. Three (2.8%) E. coli isolates had blaSHV-like, blaCTX-M, and blaTEM genes coexisting. However, the mean occurrence of ESBL encoding genes was substantially higher in E. coli (; ).
Table 4. Combinations of ESBL genes detected in Extended Spectrum β-lactmases producing E. coli and K. pneumoniae isolates
Seventy isolates (66.7%) contained one ESBL gene. The incidence of blaSHV-1-like was greatest at 17.1% (18) and 6.6% (7) for E. coli and K. pneumoniae respectively, followed by blaTEM (13.3%/14) for E. coli and (3.8%/4) for K .pneumoniae, blaSHV-2-like at 9.5% (10) for E. coli, blaCTX-M at 7.6% (8) and 4.7% (5) for E. coli and K. pneumoniae respectively and then blaCTX-M-15 at 3.8% (4) for E. coli, ; .
Table 5. One ESBL gene per isolate detected in ESBLs producing E. coli and K. pneumoniae.
8.4. Comparison of phenotypic resistance prevalence among the divisions
One-way analysis of variance using Tukey’s multiple comparisons test package was conducted to compare the mean phenotypic resistance prevalence among the different divisions of Bushenyi-Ishaka Municipality. The multiple comparison analysis registered P values >0.05 indicating statistically similar levels of phenotypic resistance among the different divisions ().
8.5. Distribution of cephalosporin genetic resistance determinants among the divisions
ESBLs encoding genes were highly distributed among the Ishaka division population with a prevalence of 15.5% followed by Bushenyi division (7.3%) and Nyakabirizi division (2.3%). Comparison of the distribution of ESBLs encoding genes among the different divisions located in Bushenyi-Ishake Municipality was performed in one-way ANOVA. Tukey’s multiple comparison analysis computed a P value >0.05 between Bushenyi and Nyakabirizi divisions indicating no statistical difference in the prevalence of ESBLs encoding genes among the population, whereas P values <0.05 were obtained between Ishaka and Bushenyi divisions, Ishaka and Nyakabirizi division signifying statistically higher prevalence of the ESBL genes among the Ishaka population. The distribution of the ESBL genes is illustrated by .
9. Discussion
Phenotypic and molecular characterization revealed that 105 (65.6%) of the isolates expressed ESBLs. The phenotypic resistance in this study is comparable to that (62.0%) reported at MNRH in 2015 [Citation25] but lower than resistance frequencies of 81.4% and 89% reported at MNRH in 2013 [Citation9] and Kabale Regional Referral Hospital (KRRH) [Citation26], respectively. Contrary to this, lower ESBLs phenotypic (29.9% and 13.4%) and genotypic (51%) frequencies were reported by studies carried out at MRRH [Citation10] and MNRH in 2017 [Citation27].
Nosocomial outbreaks in hospital settings with limited therapeutic options have been attributed to AmpC β-lactamase producing bacteria [Citation28]. In this study, combined disc diffusion and boronic acid assays revealed that only 15 isolates co-expressed ESBLs and AmpCs. Thus, a low prevalence (9.4%) of AmpC β-lactamase producers was registered by this study. These findings corroborate well with other studies that reported relatively low prevalence (0.5%–30%) of coproduction of ESBLs and AmpCs [Citation29–32]
E. coli (81.2%) isolates were more predominant than K. pneumoniae (18.8%) among the archived samples. This is in agreement with other studies that attempted to establish the prevalence of ESBLs producing Enterobacteriaceae in Uganda [Citation9,Citation10,Citation25,Citation27]. The mean prevalence of CR was higher in K. pneumoniae (90.6% ± 9.07) but not significantly different from that registered by E. coli isolates (89.3% ± 5.51). This is in contrast with two studies where the prevalence of CR reported was 52% and 44% at KRRH [Citation26] and 72.7% and 58.1% at MNRH in 2015 [Citation25] but corroborates with other studies that reported CR incidence as 79.2% and 92.3% at MNRH in 2013 [Citation9] and 85% and 88% globally [Citation33] in E. coli and K. pneumoniae, respectively. However, studies by Moses et al. [Citation10] at MRRH in 2013 and Ampaire et al. [Citation27] at MNRH in 2017 reported E. coli as the most predominant ESBL producer. Antimicrobial resistance (AMR) stewardship strategies put forward to combat antibiotic resistance can only be assessed by routine quantification of AMR prevalence. Successive studies were carried out to determine the prevalence of ESBLs producers at MNRH. Indeed, the results are promising as the prevalence of ESBL producers reduced from >75% [Citation9] to 13.4% [Citation27] reported in 2013 and 2017, respectively.
For molecular characterization of ESBL genes, blaSHV primer that amplified two genes, probably blaSHV-1-like and blaSHV-2-like, with different amplicon sizes was used. The commonest ESBL gene identified was blaSHV (SHV-1 and SHV-2) (42%) followed by blaTEM (27.3%), blaCTX-M (22.4%), and blaCTX-M-15 (8.4%). However, these findings are not in agreement with the ESBL genes prevalence registered at MRRH where the most prevalent ESBL gene was CTX-M (70%), SHV (34%), and TEM (0.8%) [Citation10] but comparable to ESBL gene prevalence reported in other parts of the world. For example, studies conducted in Australia, Belgium, Turkey, South Africa, and USA reported blaSHV (67.1%) as the most prevalent gene, trailed by CTX-M-type(23.3%) and TEM–type (16.4%) [Citation34], and this incidence is similar in many other countries [Citation35–37]. Furthermore, 35 out of the 105 ESBLs positive isolates expressed more than one ESBL gene. This collaborates with Moses et al.’s [Citation10] study at MRRH where 87 of the 213 isolates harbored more than one ESBL gene and other studies done outside Uganda. For instance, in Turkey, 68 isolates had SHV/TEM coexisting, 61 had TEM/CTX-M, and 54 had SHV/CTX-M genes [Citation38]. Moreover, seven (21.87%) isolates containing TEM/SHV were reported in Iran [Citation39]. It is worth noting that this study registered a rare occurrence of three genes: blaSHV-1/blaCTX-M/blaTEM coexisting in three of the E. coli isolates. Existence of three ESBL genes in one isolate is in line with Mohsen et al.’s [Citation40] study who reported the co-occurrence of blaCTX-M/blaTEM/blaSHV in 14 isolates.
Furthermore, 55 out of 160 isolates contained neither ESBLs nor AmpCs but exhibited excellent CR. This resistance may be attributed to the presence of resistance intrinsic factors, such as downregulation and mutation of the outer membrane proteins, through which antibiotics diffuse [Citation41–44]. High prevalence of carbapenem resistance has been reported in Uganda [Citation45–47]. Acquisition of carbapenemase encoding genes by Enterobacteriaceae confer resistance to all beta lactam antibiotics [Citation41,Citation45]. Thus, the most likely source of resistance in the 55 isolates is probably the presence of carbapenem resistance genes.
Among the three divisions, Ishaka has the highest population density (891.0/km2), followed by Bushenyi division also known as Central division (490.4/km2), and Nyakabirizi division (248.1/km2) [Citation48]. Statistical comparison of phenotypic resistance prevalence and distribution of cephalosporin-resistance determinants within the divisions of Bushenyi-Ishaka Municipality revealed higher phenotypic resistance and distribution of ESBLs encoding genes in the population of Ishaka division. The results corroborate well with Bruinsma et al.’s [Citation49] findings. While studying the impact of population density on antibiotic resistance, Bruinsma et al. [Citation49] observed that population density is a vital aspect that influences the development of antibiotic resistance. Similar findings were reported by Honda et al. [Citation50].
10. Conclusions
High prevalence of CR in E. coli and K. pneumoniae isolated from patients treated at KIU-TH was revealed by this study. K. pneumoniae was encountered as the most prevalent resistant isolate and blaSHV as the most predominant gene. Thus, the third-generation cephalosporin endorsed for treatment of Enterobacteriaceae infections were not effective in vitro. Indeed, this study accentuates routine screening of AMR in different health-care facilities. This will guide clinicians on the rational prescription of antibiotics so as to control the emergence and transmission of resistant strains. This study has provided an insight into the possible presence of determinants of CR other than ESBL and AmpCs; thus, further investigations are required to determine the other factors responsible for CR among Enterobacteriaceae isolated from patients attending KIU-TH.
Ethics and consent to participate
Ethical Approval No: NrUG-REC-023/201,838 was granted by the Research Ethics Committee for ethical review and approval, Kampala International University-Western Campus. The Research Ethics Committee waived the need for informed consent to use already coded archived samples in this study.
Limitations
This study did not look at carbapenem resistance, yet carbapenemase production confers resistance to all beta-lactams. Phenotypic resistance in isolates that neither harboured ESBLs nor AmpCs may be due to the presence of carbapenemase genes. Primers used to detect ESBLs encoding genes in this study targeted most gene variants. Thus, sequencing of the amplicons should be done to distinguish between the several gene variants.
Authors’ contributions
This work was carried out in collaboration between all authors. Eddie Wampande and Kenneth Ssekatawa conceptualized and designed the format for this study. Kenneth Ssekatawa and Herbert Mbyemeire carried out all the laboratory experiments. Kenneth Ssekatawa conducted data analysis. All authors drafted and managed manuscript revisions. All authors read and approved the final manuscript.
Acknowledgments
We are thankful to the Microbiology Laboratory and Molecular Laboratory, College of Veterinary Medicine Animal Resources and Biosecurity for providing laboratory space.
Availability of data and materials
Supplementary data or any other forms of data have been submitted. Raw data and any other forms data generated by this project can be obtained from the authors on request by e-mail.
Disclosure statement
The authors declare that they have no competing interests.
Additional information
Funding
Notes on contributors
Herbert Mbyemeire
Herbert Mbyemeire is an M.Sc. student and teaching assistant at the Department of Biochemistry, Faculty of Biomedical Sciences, Kampala International University-Western Campus. Herbert's research interests are in molecular microbiology and antimicrobial resistance.
Kenneth Ssekatawa
Kenneth Ssekatawa is a Research Scientist at MAPRONANO ACE, Makerere University and Lecturer Department of Biochemistry, Faculty of Biomedical Sciences, Kampala International University-Western Campus. Kenneth specializes in molecular microbiology, antimicrobial resistance, phage therapy and nanobiotechnology.
Charles D. Kato
Charles D. Kato is a lecturer at the College of Veterinary Medicine Animal Resources and Biosecurity, Makerere University. Charles' research interests are in biomarker research regarding disease co-infection and host-parasite interactions in infectious and zoonotic diseases. Charles also runs various antimicrobial resistance projects.
Eddie M. Wampande
Eddie M. Wampande is a senior lecturer at the College of Veterinary Medicine Animal Resources and Biosecurity, Makerere University. Eddie specializes in molecular microbiology and parasitology, as well as antimicrobial resistance.
References
- Deck D, Winston L. Beta-lactam and other cell wall-and membrane-active antibiotics. J Basic Clin Pharmacol. 2012.
- Cherubin CE, Corrado ML, Nair SR, et al. Treatment of gram-negative bacillary meningitis: role of the new cephalosporin antibiotics. Reviews of Infectious Diseases. 1982;4(Supplement_2):S453–S464.
- Vading M, Nauclér P, Kalin M, et al. Invasive infection caused by Klebsiella pneumoniae is a disease affecting patients with high comorbidity and associated with high long-term mortality. PLOS ONE. 2018;13(4):e0195258.
- Iredell J, Brown J, Tagg K. Antibiotic resistance in Enterobacteriaceae: mechanisms and clinical implications. J Bmj. 2016;352.
- Stokes HW, Gillings MR. Gene flow, mobile genetic elements and the recruitment of antibiotic resistance genes into Gram-negative pathogens. J FEMS Microbiol Rev. 2011;35(5):790–819.
- Rayamajhi N, KANG S, Lee D, et al. Characterization of TEM-, SHV-and AmpC-type β-lactamases from cephalosporin-resistant Enterobacteriaceae isolated from swine. Int J Food Microbiol. 2008;124(2):183–187.
- Park Y-J, Yu JK, Lee S, et al. Prevalence and diversity of qnr alleles in AmpC-producing Enterobacter cloacae, Enterobacter aerogenes, Citrobacter freundii and Serratia marcescens: a multicentre study from Korea. J Antimicrob Chemother. 2007;60(4):868–871.
- Tian G-B, et al. Detection of CTX-M-15, CTX-M-22, and SHV-2 extended-spectrum β-lactamases (ESBLs) in Escherichia coli fecal-sample isolates from pig farms in China. 2009;6(3):297–304.
- Seni J, Najjuka CF, Kateete DP, et al. Antimicrobial resistance in hospitalized surgical patients: a silently emerging public health concern in Uganda. BMC Res Notes. 2013;6(1):298. .
- Moses A, Bwanga F, Boum Y, et al. Prevalence and genotypic characterization of extended-spectrum beta-lactamases produced by gram negative bacilli at a tertiary care hospital in Rural South Western Uganda. J Br Microbiol Res J. 2014;4(12):1541.
- Ronald A. The etiology of urinary tract infection: traditional and emerging pathogens. Am J Med. 2002;113(1):14–19.
- Aldeyab MA, Kearney MP, McElnay JC, et al. A point prevalence survey of antibiotic prescriptions: benchmarking and patterns of use. J Br Clin Pharmacol. 2011;71(2):293–296.
- Bullock NO, Aslanzadeh J. Biochemical profile-based microbial identification systems. In: Advanced techniques in diagnostic microbiology. Springer; 2013. p. 87–121.
- Alves MS, Dias RCDS, De Castro ACD, et al. Identification of clinical isolates of indole-positive and indole-negative Klebsiella spp. J Clin Microbiol. 2006;44(10):3640–3646.
- CLSI. Performance standards for antimicrobial disk susceptibility tests for bacteria isolated from animals: CLSI supplement VET01SEd5E; replaces VET01-S2. Pennsylvania:Clinical and Laboratory Standards Institute; 2020.
- Jørgensen RL, Nielsen JB, Friis-Møller A, et al. Prevalence and molecular characterization of clinical isolates of Escherichia coli expressing an AmpC phenotype. J Antimicrobial Chemother. 2010;65(3):460–464.
- Sato T, Harada K, Usui M, et al. Tigecycline susceptibility of Klebsiella pneumoniae complex and escherichia coli isolates from companion animals: the prevalence of tigecycline-nonsusceptible K. pneumoniae complex, including internationally expanding human pathogenic lineages. J Microbial Drug Resistance. 2018;24(6):860–867. .
- Poulou A, Grivakou E, Vrioni G, et al. Modified CLSI extended-spectrum β-lactamase (ESBL) confirmatory test for phenotypic detection of ESBLs among Enterobacteriaceae producing various β-lactamases. J Clin Microbiol. 2014;52(5):1483–1489.
- Hemalatha V, et al. Detection of Amp C beta lactamases production in Escherichia coli & Klebsiella by an inhibitor based method. J Med Res. 2007;126(3):220.
- Coudron PE. Inhibitor-based methods for detection of plasmid-mediated AmpC β-lactamases in Klebsiella spp., Escherichia coli, and Proteus mirabilis. J Clin Microbiol. 2005;43(8):4163–4167.
- Voets GM, Fluit AC, Scharringa J, et al. A set of multiplex PCRs for genotypic detection of extended-spectrum β-lactamases, carbapenemases, plasmid-mediated AmpC β-lactamases and OXA β-lactamases. J Int Antimicrobial Agents. 2011;37(4):356–359.
- Dallenne C, Da Costa A, Decré D, et al. Development of a set of multiplex PCR assays for the detection of genes encoding important β-lactamases in Enterobacteriaceae. J Antimicrob Chemother. 2010;65(3):490–495.
- Bradford PA. Extended-spectrum β-lactamases in the 21st century: characterization, epidemiology, and detection of this important resistance threat. J Clin Microbiol Rev. 2001;14(4):933–951.
- Memariani M, Najar Peerayeh S, Zahraei Salehi T, et al. Occurrence of SHV, TEM and CTX-M β-lactamase genes among enteropathogenic Escherichia coli strains isolated from children with diarrhea. Jundishapur J Microbiol. 2015;8(4). DOI:https://doi.org/10.5812/jjm.8(4)2015.15620.
- Kateregga JN, Kantume R, Atuhaire C, et al. Phenotypic expression and prevalence of ESBL-producing Enterobacteriaceae in samples collected from patients in various wards of Mulago Hospital, Uganda. BMC Pharmacol Toxicol. 2015;16(1):14. .
- Andrew B, Kagirita A, Bazira J. Prevalence of extended-spectrum beta-lactamases-producing microorganisms in patients admitted at KRRH, Southwestern Uganda. Int J Microbiol. 2017;2017:3183076.
- Ampaire L, Nduhura E, Wewedru I. Phenotypic prevalence of extended spectrum beta-lactamases among enterobacteriaceae isolated at Mulago National Referral Hospital: uganda. BMC Res Notes. 2017;10(1):448.
- Reuland EA, Hays JP, de Jongh DMC, et al. Detection and occurrence of plasmid-mediated AmpC in highly resistant gram-negative rods. PloS One. 2014;9(3):e91396.
- Chaudhary U, Agarwal S, Raghuraman K. Identification of extended spectrum beta lactamases, AmpC and carbapenemase production among isolates of Escherichia coli in North Indian tertiary care centre. Avicenna J Med. 2018;8(2):46–50.
- Rizi KS, Mosavat A, Youssefi M, et al. High prevalence of blaCMY AmpC beta-lactamase in ESBL co-producing Escherichia coli and Klebsiella spp. clinical isolates in the northeast of Iran. J Glob Antimicrob Resist. 2020;22:477–482.
- Lan NPH, Hien NH, Le Thi Phuong T, et al. Phenotypic and genotypic characteristics of ESBL and AmpC producing organisms associated with bacteraemia in Ho Chi Minh City, Vietnam. Antimicrob Resist Infect Control. 2017;6(1):105. .
- Kurittu P, Khakipoor B, Aarnio M, et al. Plasmid-borne and chromosomal ESBL/AmpC Genes in Escherichia coli and Klebsiella pneumoniae in global food products. Frontiers in Microbiology. 2021;12(125). DOI:https://doi.org/10.3389/fmicb.2021.592291.
- Centre for Disease Dynamics Economics and Policy-CDDEP. The state of the world’s antibiotics. Washington DC-New Delhi:CDDEP; 2015.
- Paterson DL, et al. Extended-spectrum β-lactamases in Klebsiella pneumoniae bloodstream isolates from seven countries: dominance and widespread prevalence of SHV-and CTX-M-type β-lactamases. J Antimicrobial Agents Chemotherapy. 2003;47(11):3554–3560.
- Al-Subol I, Youssef N. Prevalence of CTX-M, TEM and SHV beta-lactamases in clinical isolates of Escherichia Coli and Klebsiella Pneumoniae isolated from Aleppo University Hospitals, Aleppo, Syria. J Archives Clinical Infectious Dis. 2015;10(2):e22540.
- Fernandes R, Amador P, Prudêncio C. β-Lactams: chemical structure, mode of action and mechanisms of resistance. J Rev Med Microbiol. 2013;24(1):7–17.
- Feizabadi MM, Delfani S, Raji N, et al. Distribution of bla TEM, bla SHV, bla CTX-M Genes Among Clinical Isolates of Klebsiella pneumoniae at Labbafinejad Hospital, Tehran, Iran. J Microbial Drug Resistance. 2010;16(1):49–53.
- Yazdi M, et al. Genotypic versus Phenotypic methods to detect extended-spectrum beta-lactamases (ESBLs) in uropathogenic Escherichia coli. J Ann Biol Res. 2012;3(5):2454–2458.
- Nasehi L, et al. PER, CTX-M, TEM and SHV Beta-lactamases in clinical isolates of Klebsiella pneumoniae isolated from Tehran, Iran. Iran J Basic Med Sci. 2010;13(3):111–118.
- Mohsen SMY, et al. Antimicrobial susceptibility of Klebsiella pneumoniae and Escherichia coli with extended-Spectrum β-lactamase associated genes in hospital Tengku ampuan afzan, Kuantan, Pahang. J Med Sci. 2016;23(2):14.
- Nordmann P, Dortet L, Poirel L. Carbapenem resistance in Enterobacteriaceae: here is the storm! J Trends Molecular Med. 2012;18(5):263–272.
- Tängdén T, Adler M, Cars O, et al. Frequent emergence of porin-deficient subpopulations with reduced carbapenem susceptibility in ESBL-producing Escherichia coli during exposure to ertapenem in an in vitro pharmacokinetic model. J Antimicrobial Chemotherapy. 2013;68(6):1319–1326. .
- Novais A, Rodrigues C, Branquinho R, et al. Spread of an OmpK36-modified ST15 Klebsiella pneumoniae variant during an outbreak involving multiple carbapenem-resistant Enterobacteriaceae species and clones. J Clin Microbiol Infectious Dis. 2012;31(11):3057–3063. .
- Lavigne J-P, Sotto A, Nicolas-Chanoine M-H, et al. An adaptive response of Enterobacter aerogenes to imipenem: regulation of porin balance in clinical isolates. J Int Antimicrobial Agents. 2013;41(2):130–136.
- Ssekatawa K, Byarugaba DK, Wampande E, et al. A systematic review: the current status of carbapenem resistance in East Africa. BMC Res Notes. 2018;11(1):629.
- Ssekatawa K, Byarugaba DK, Nakavuma JL, et al. Prevalence of pathogenic Klebsiella pneumoniae based on PCR capsular typing harbouring carbapenemases encoding genes in Uganda tertiary hospitals. Antimicrob Resist Infect Control. 2021;10(1):57.
- Ssekatawa K, Byarugaba DK, Nakavuma JL, et al. Carbapenem resistance profiles of Pathogenic Escherichia coli in Uganda. J Biol Biotechnol. 2021;2(2):63–73. .
- [cited 2021 May 16]. https://www.citypopulation.de/en/uganda/cities/?cityid=12020
- Bruinsma N, et al. Influence of population density on antibiotic resistance. J Antimicrobial Chemotherapy. 2003;51(2):385–390.
- Honda R, Watanabe T, Sawaittayotin V, et al. Impacts of urbanization on the prevalence of antibiotic-resistant Escherichia coli in the Chaophraya River and its tributaries. J Water Sci Technol. 2016;73(2):362–374.