ABSTRACT
Objective
Diffuse large B-cell lymphoma (DLBCL) is an aggressive type of lymphoma. Circulating cell-free DNA (cfDNA) is the promising liquid biopsy in malignancy.
Method
Assessment of cfDNA concentration and DNA integrity index (DII) in 30 newly diagnosed DLBCL patients and correlating them with the international prognostic index.
Results
Circulating cfDNA concentration was significantly higher in newly diagnosed DLBCL patients than controls, while DII was significantly lower. There was a significant correlation between cfDNA concentration and adverse prognostic factors.
Conclusion
cfDNA concentration is potentially strong diagnostic and prognostic marker of DLBCL, however DII is only useful as a diagnostic biomarker.
1. Introduction
Lymphoma is a group of heterogenous malignancies originating from lymphoid cells at different stages of development, either B-cells, T-cells or natural killer (NK) cells [Citation1]. Hodgkin lymphoma accounts for 10% of lymphoma while non-Hodgkin lymphoma accounts for 90% of cases [Citation2]. The most prevalent lymphoma worldwide is Diffuse large B-cell lymphoma (DLBCL). It is characterized as a diffusely growing malignancy of giant B-lymphocytes with nuclei that are larger than or equal to the size of normal macrophage nuclei or more than twice the size of a lymphocyte. Patients with DLBCL typically present with a rapidly enlarging mass either involving a single or several lymph nodes or an extra nodal site [Citation1]. Despite being possibly treatable, DLBCL is a clinically aggressive cancer that develops quickly and typically distributes malignant cells throughout the body in its early stages; therefore, it requires early diagnosis, urgent & intensive therapy, and close monitoring [Citation3].
Clinical evaluation, invasive biopsies, CT and PET scans are currently the main methods used in DLBCL diagnosis, prognosis and disease monitoring. Those techniques are highly valuable; however, a simpler tool is desperately needed to evaluate prognosis, continuously track the patient’s response to treatment, and enable earlier detection of relapse [Citation4]. Circulating cell free DNA (ccfDNA) is this promising biomarker; the liquid gold that must be further studied to obtain such an easily accessible biomarker that can reveal a great deal about the underlying malignant clone [Citation5].
Circulating cfDNA is mainly composed of double stranded DNA fragments circulating in the bloodstream of both healthy and diseased individuals [Citation6]. In general, ccfDNA is found in low concentrations; however, ccfDNA levels are elevated in certain conditions such as pregnancy, malignant diseases and nonmalignant diseases such as inflammatory conditions, autoimmune diseases, trauma, myocardial infarction, stroke, sepsis and allograft transplant rejection [Citation5–7].
Circulating cfDNA is believed to originate from apoptotic cells, necrotic cells or actively secreted by cells [Citation8]. Under normal circumstances, leukocytes and other normal cells shed some cfDNA into the blood. In cancer patients, ccfDNA contains tumor derived DNA that’s shed by malignant cells into peripheral blood which can be used to determine the molecular profile and tumor burden [Citation9,Citation10].
The DNA integrity which describes the size distribution of cfDNA, thereby reflecting its fragmentation, varies according to cellular origin and/or the mechanism of cellular death. It has been found that cfDNA in cancer patients show a different fragmentation profile from cfDNA in healthy individuals; therefore, the integrity of cfDNA has been investigated as a potential diagnostic and prognostic marker in several cancers [Citation8,Citation11].
Since the DNA integrity index is calculated as the ratio of longer DNA fragments to shorter DNA fragments, those measurements can be obtained by the amplification of two reference genes of different lengths using real time quantitative PCR (qPCR). In recent years, Alu elements have been the most used qPCR target to assess DNA integrity in cancer patients [Citation12]. ALU elements are repetitive non-coding DNA elements that constitute at least 11% of the human genome [Citation13]. ALU 111 primer can be used to amplify shorter cfDNA fragments and ALU 260 primers can be used to amplify longer fragments. The DNA integrity index (DII) can be calculated as the ratio between longer and shorter cfDNA fragments [Citation14].
Recently, there is a great interest in using circulating cell free DNA in the diagnosis and prognosis of several malignancies. Therefore, the aim of our study was to evaluate the cfDNA concentration and DNA integrity index in DLBCL patients and to correlate them with the international prognostic index of DLBCL.
2. Methods
The study included 30 newly diagnosed patients with DLBCL and 30 healthy adults as a control group. The patients’ ages varied from 43 to 91 years old, while the controls were between the ages of 40 and 85. Males represented 50% of the cases and 53.3% of the controls. Patients who were pregnant or had any other malignancy, autoimmune disease, myocardial infarction, hepatic insufficiency, or renal insufficiency were excluded from the study. Written informed consent was obtained from all enrolled participants. The study was conducted after the approval of the Medical Ethics Committee, in accordance with the 1964 Helsinki Declaration and its subsequent iterations.
2.1. Sample collection and cfDNA extraction
Peripheral blood samples were withdrawn into EDTA tubes. Within 4–6 hours the blood samples were firstly centrifuged at 1600 × g for 10 min at 4°C. To avoid any contamination from cellular DNA, the supernatant plasma was carefully collected and then centrifuged again at a higher speed of 6000 × g for 10 min at 4°C. The supernatant plasma was collected and kept for a maximum of two weeks at −80°C until extraction. ccfDNA was extracted using Magcore® circulating DNA large volume kit, (RBC Bioscience Corp., Belgium). The extracted cfDNA was eluted in 60 μl solution and stored at −20°C until further analysis.
2.2. Quantification of cfDNA
The extracted cfDNA was quantified by fluorometry using the Qubit dsDNA HS Assay kit (ThermoFisher Scientific, USA) according to the manufacturer’s instructions. The two standard solutions included in the kit were used to create a standard curve from which the concentrations of cfDNA were determined.
2.3. Assessment of DNA integrity index
The DII was assessed by Quantitative Real time PCR using Quanti Nova SYBR Green PCR kit (catalog numbers: 208052) to amplify ALU 111 and ALU 260 primers which represent shorter and longer DNA fragments respectively. Since the annealing sites of ALU 111 are contained within the annealing sites of ALU 260, ALU 111 amplified both shorter and longer fragments (total cfDNA fragments) and ALU 260 amplified only longer fragments. The sequence of the primers was: ALU 260: forward, 5′- ACG CCT GTA ATC CCA GCA −3′ and reverse, 5′- CGG AGT CTC GCT CTG TCG −3′; and ALU 111: forward, 5′- CTG GCC AAC ATG GTG AAA C −3′ and reverse, 5′- AGC GAT TCT CCT GCC TCA G −3′.
To achieve a concentration of 0.8 ng DNA/reaction and a total reaction volume of 20 ul, each quantitative PCR reaction mixture contained 10 ul of 2× SYBR Green PCR Master Mix, 1 ul of forward primer, 1 ul of reverse primer, and the appropriate volumes of template DNA and RNase-free water. The used real time PCR protocol was comprised of forty cycles of denaturation at 95°C for five seconds and annealing at 60°C for ten seconds.
The absolute concentrations of shorter and longer cfDNA fragments were determined using serial dilutions of a standard human genomic DNA G304A (Promega, USA). The DII was calculated as the ratio of the absolute concentration of longer cfDNA fragments to the absolute concentration of shorter cfDNA fragments (ALU260/ALU111).
2.4. Statistical analysis of the data
Data were analyzed using IBM SPSS software package version 20.0. (Armonk, NY: IBM Corp). Qualitative data were described using number and percent. Quantitative data were described using mean, standard deviation, median and interquartile range (IQR). Significance of the obtained results was judged at the 5% level. The used tests were Chi-square test, Student t-test,Mann Whitney test and Spearman coefficient. The diagnostic performance was measured by Receiver operating characteristic curve (ROC).
3. Results
3.1. Clinical and prognostic assessment of the DLBCL patients
Among the 30 studied cases, 80% exhibitedB-symptoms including fever, night sweats and weight loss, while 20% didn’t have any B-symptoms. 33.3% of the cases had splenomegaly whereas 6.7% of had hepatomegaly. According to their performance status at diagnosis: 26.7% were grade zero, 23.3% were grade 1, 26.7% were grade 2, 10% were grade 3 and 13.3% were grade 4. Twenty percent of the studied cases had bulky disease which is defined as the presence of a nodal or extranodal lesion with a diameter ≥7.5 cm according to the NCCN (National Comprehensive Cancer Network) guidelines for DLBCL [Citation15].
According to the Ann Arbor classification, 16.7% of the studied patients were diagnosed at stage II, while 36.7% were at stage III and 46.7% were at stage IV. The prognostic status of the patients was assessed at the time of diagnosis by calculating the international prognostic index (IPI) score. 6.7% of the cases scored zero, 23.3% scored one, 26.7% scored two, 36.7% scored three and 6.7% scored four.
3.2. Laboratory parameters of DLBCL cases
Kidney function evaluation showed that the ranges for urea and creatinine were 17–78 mg/dL and 0.55–2 mg/dL, respectively. The measured level of lactate dehydrogenase (LDH) was 344.50 ± 169.71 U/l on average. White blood cells (WBCs) ranged from 3.4 to 36.3 × 10^9/L, platelets from 9.9 to 500 × 10^9/L, and hemoglobin from 8.1 to 15.4 g/dL, according to complete blood counts.
3.3. Cell free DNA concentrations
Our study showed that DLBCL newly diagnosed cases had significantly higher ccfDNA as compared to healthy controls with a p value < 0.001 as shown in . In DLBCL patients, the median concentration of cfDNA was 396 ng/ml (Inter quartile range (IQR): 100 –21,200 ng/ml), whereas in healthy controls, the median concentration was 167 ng/ml (IQR: 20–492 ng/ml). illustrates the results of Roc curve analysis that identified patients as having cfDNA concentrations more than 227 ng/ml with an AUC of 0.821, 70% sensitivity, and 90% specificity, indicating a very good diagnostic power.
Figure 1. Comparison between the two studied groups according to: (a) cFDNA concentration. (b) DNA integrity index.
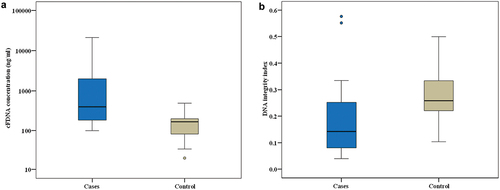
Figure 2. ROC curve showing the diagnostic performance for: (a) cFDNA concentration. (b) DNA integrity index.
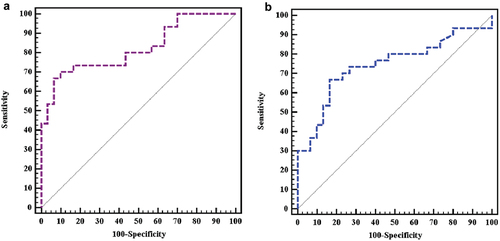
A statistically significant correlation was observed between the concentrations of cfDNA and adverse prognostic factors like performance status scores (p value is < 0.001), stage at diagnosis (p value is 0.001), IPI score (p value is < 0.001), the presence of B-symptoms (p value = 0.021), the presence of bulky disease (p value = 0.044) and levels of LDH (p value is < 0.001). Furthermore, there was a statistically significant association between the cfDNA concentrations and the levels of blood urea (p value is 0.048), hemoglobin levels (p value is 0.001) and WBC counts (p value is 0.004). These correlations are demonstrated in ).
Table 1. Correlation between cfDNA concentration and DII with prognostic and laboratory parameters.
Table 2. Relation between cfDNA concentration and DII with clinical parameters.
3.4. DNA integrity index
Our study revealed that DLBCL patients had a considerably lower DNA integrity index (DII) than healthy controls, with a p value of 0.001 as shown in . Among DLBCL patients, the median DII was 0.141 (IQR: 0.039–0.576). While among healthy controls, the median DII was 0.258 (IQR: 0.103–0.500).
As seen in , a cut off value of 0.1933 was found to distinguish cases from controls. Cases are defined as having a DII of equal to or less than 0.1933, with an AUC of 0.742, 66.67% sensitivity, and 83.33% specificity, indicating a good diagnostic power. present that there was no statistically significant correlation between DII of DLBCL cases and any of the adverse prognostic factors or laboratory or clinical parameters.
4. Discussion
DLBCL is the most common type of aggressive NHL worldwide [Citation1]. Its rapidly escalating clinical course urges the need for novel tools to facilitate earlier diagnosis and better prognosis and disease monitoring. cfDNA is an emerging liquid biopsy that requires more research to optimize its use in the field of hematological cancers especially lymphoma as it provides an image of the original tumor [Citation9].
The present study demonstrated that newly diagnosed DLBCL cases showed significantly higher ccfDNA as compared to healthy controls (p value < 0.001) in agreement with a study conducted on lymphoma patients by Mao Li et al. [Citation5] They showed that cfDNA levels >340 ng/ml can discriminate DLBCL cases from controls in harmony with our cut off value at 227 ng/ml with an AUC of 0.821. This reveals the strong diagnostic power of cfDNA concentrations in DLBCL. Mao Li et al. had a bigger sample size (174 lymphoma patients and 80 healthy individuals) than we did, which may have led to their higher cut off point because their control subjects may have had more nonmalignant variables present that might have elevated the cfDNA in them.
In another study by Hohaus et al. [Citation16] that was conducted on 142 lymphoma patients including 63 DLBCL patients and 41 normal controls, the cfDNA concentrations were significantly higher in patients than controls despite using a different quantification method which was quantitative PCR using primers for the β-globin gene.
Our study also showed that higher cfDNA concentrations were significantly associated with adverse prognostic factors like higher performance status scores, late stage at diagnosis, higher IPI scores, the presence of B-symptoms, the presence of bulky disease and higher levels of LDH. The positive correlation between higher cfDNA concentrations and these adverse prognostic factors show that cfDNA concentration can reflect both the active proliferation of the disease and the burden of lymphoma [Citation5]. Therefore, cfDNA concentration can serve as a promising prognostic tools in DLBCL. Those findings were supported by Mao Li et al. [Citation5] study which demonstrated the strong correlation between cfDNA concentration and advanced stage at diagnosis, higher LDH levels and the presence of B-symptoms. Moreover, their study demonstrated that DLBCL patients with higher cfDNA concentrations had an inferior 2 year-rate of progression free survival.
Consistent with our findings, Rivas-Delgado et al.’s study [Citation17] showed that high circulating tumor DNA levels (identified by NGS) were positively correlated with clinical parameters of tumor burden which included higher IPI score, advanced stage at diagnosis as well as laboratory parameters as higher LDH levels and higher β2-microglobulin levels and volumetric parameters of tumor burden like TMVT (total metabolic tumor volume) measured at baseline PET scan/CT scan. The research by Rivas-Delgado et al. demonstrated the robust prognostic value of cfDNA in DLBCL patients. This study additionally elucidated the effectiveness of using cfDNA to assess mutations in DLBCL patients, suggesting that cfDNA might be a very good and readily available technique for genotyping DLBCL patients, which is crucial for tailoring therapy for each patient [Citation17].
According to our study, DLBCL patients had considerably lower DII than healthy controls, which is consistent with the findings of Madhavan et al. [Citation18]and Bortul et al. [Citation19]who evaluated the DNA integrity of cfDNA in breast cancer patients using ALU 260/ALU 111. Additionally, Bortul et al. [Citation19] used LINE-1 266 and LINE-1 97 primers to assess DII and showed the same results. Interestingly, all studies that used ALU 260/ALU 111 or LINE-1 266/LINE-1 97 as an index for DNA integrity of cfDNA found the DII to be significantly lower in cancer patients than in healthy individuals [Citation19].
Arecent study in 2022 by Kumar et al. [Citation20] showed a lower DII in hepatocellular carcinoma patients using long and short primer sets for ALU elements (115bp & 247 bp) and GAPDH gene (110 bp & 205 bp) compared to healthy individuals or even chronic liver disease patients. Their study found that DII could significantly discriminate liver cancer patients from healthy persons or chronic liver disease patients at a cutoff value of <0.02 for DII using GAPDH gene and 0.05 for DII using ALU elements. However, DII using LINE-1elements and β-actin gene didn’t show any significant difference between cancer patients and healthy individuals or chronic liver disease patients [Citation20].
Our results are also supported by a study by Poulet et al. [Citation8] which showed much higher fragmentation of cfDNA in metastatic colorectal cancer patients compared to normal individuals. They used a completely different approach to assess DNA integrity index of cfDNA. They target three mutant forms of KRAS gene and its wild type using digital droplet PCR (ddPCR) to amplify sequences of different lengths. Nonetheless, metastatic colorectal cancer patients showed significantly lower DII as compared to normal individuals in agreement with our results.
Contrasting our DII results, Mao Li et al. [Citation5] found that the DII of DLBCL patients was significantly higher than DII in healthy controls arguing that cancer patients have higher levels of longer cfDNA fragments. This difference could be explained by the fact that Mao Li et al. [Citation5] amplified a different gene by the quantitative real time PCR to assess DII, which is the APP gene (Amyloid beta precursor protein gene) using APP 67 primer and APP 180 primer to amplify shorter and longer fragments respectively.
Moreover, in a study conducted on breast cancer patients, Elhelaly et al. [Citation21] used ALU 247 and ALU 115 primers and showed that DII was significantly higher in breast cancer patients compared to healthy women or women with benign breast conditions. However, in their study, serum samples were used for cfDNA extraction. Serum has been shown by different studies to contain higher cfDNA concentrations due to contamination by DNA released from white blood cells after their lysis due to clotting and this might be the source of the increased long fragments in their study [Citation22,Citation23]. Furthermore, the Elhelaly et al. performed 2 centrifugation steps at 3000 rpm during processing of their serum samples and this low speed might have led to some cellular DNA contamination which could alter the DII results. Madhavan et al. [Citation18] emphasized the importance of the second high speed centrifugation step in the processing of plasma samples before cfDNA extraction to eliminate contamination of cfDNA with cellular debris. They showed that significantly lower cfDNA concentrations and altered cfDNA integrity were observed in plasma samples obtained by two centrifugation steps including a second high speed one at 15,500 g in contrast to plasma samples that were obtained by a single low-speed centrifugation step at 4000 g.
The results of cfDNA integrity index in cancer patients among different studies are quite heterogenous. While some studies show increased DII in cancer patients, other studies show decreased DII in cancer patients as compared to healthy individuals, so it’s quite controversial whether malignancies result in more fragmentation of cfDNA or less fragmentation [Citation24]. However, many supporting studies argue that the pathological death of cells in cancer patients lead to more fragmented cfDNA resulting in lower DII, in contrast to normal apoptosis in healthy individuals [Citation20,Citation25–27].
The primary explanation for the variation in DII results among research is the quantitative PCR-based evaluation approach, which indirectly estimates real values. This can lead to wide variation between results according to the properties of the primers used or the real-time amplification. Furthermore, the DII findings can be significantly impacted by preanalytical parameters such as the time interval between blood draw and processing as well as the centrifugation steps particularly the second high-speed phase that efficiently removes cellular debris [Citation18]. Additionally and perhaps more importantly, earlier studies of cfDNA in cancer patients that showed DII to be higher in cancer patients than normal individuals, used ubiquitous genes that code for normal proteins like b-actin and leptin, which couldn’t actually discriminate whether the increased longer DNA fragments were derived from cancer cells or not. More recent research employing genes specific to cancer cells, such as KRAS or mutant APC (Adenomatous Polyposis Coli) in colorectal cancer confirmed that circulating cell free tumor DNA is mainly composed of shorter DNA fragments [Citation28].
The more fragmented cfDNA in cancer patient compared to healthy individuals, was also verified by Cristiano et al. [Citation29] study that performed genome-wide fragmentation analysis using next generation sequencing (NGS). The fragmentation profiles of cfDNA (ratio between short fragments and long fragments) showed that healthy persons have similar cfDNA fragmentation profiles while cancer patients have highly variable cfDNA fragmentation profiles with longer and shorter fragments at different locations in the genome [Citation29]. This can partially explain the great variation in DII results obtained from amplification of different genes among the different studies. To optimize the use of cfDNA as a liquid biopsyin DLBCL, cfDNA fragmentation in DLBCL has to be more investigated using different genes or molecular elements. Standardization of DII assessment methods is also required. In addition, studying cfDNA’s role in minimum residual disease monitoring in DLBCL patients following therapy is highly recommended.
Our study concludes that cfDNA is a promising valuable noninvasive method for DLBCL patients. Circulating cfDNA concentration and DII are appealing diagnostic tools since they can effectively discriminate DLBCL patients from healthy individuals. Moreover, cfDNA concentration is considered a reliable prognostic biomarker in DLBCL, whereas DII is not and requires further research to be used for prognosis.
Disclosure statement
No potential conflict of interest was reported by the author(s).
References
- Krause JR. WHO classification of tumours of haematopoietic and lymphoid tissues: an overview. Crit Values. 2009;2(2):30–32. doi: 10.1093/criticalvalues/2.2.30
- Armitage JO, Gascoyne RD, Lunning MA, et al. Non-Hodgkin lymphoma. Lancet. 2017;390(10091):298–310. doi: 10.1016/S0140-6736(16)32407-2
- Ernst M, Oeser A, Besiroglu B, et al. Chimeric antigen receptor (CAR) T‐cell therapy for people with relapsed or refractory diffuse large B‐cell lymphoma. Cochrane Database Syst Rev. 2021;2021(9):9. doi: 10.1002/14651858.CD013365.pub2
- Jones K, Nourse JP, Keane C, et al. Tumor‐specific but not nonspecific cell‐free circulating DNA can be used to monitor disease response in lymphoma. Am J Hematol. 2012;87(3):258–265. doi: 10.1002/ajh.22252
- Li M, Jia Y, Xu J, et al. Assessment of the circulating cell-free DNA marker association with diagnosis and prognostic prediction in patients with lymphoma: a single-center experience. Ann Hematol. 2017;96(8):1343–1351. doi: 10.1007/s00277-017-3043-5
- Breitbach S, Tug S, Simon P. Circulating cell-free DNA: an up-coming molecular marker in exercise physiology. Sports Med. 2012;42(7):565–586. doi: 10.2165/11631380-000000000-00000
- Ranucci R. Cell-free DNA: applications in different diseases. Cell-Free DNA Diagn Markers: Methods Protoc. 2019:3–12.
- Poulet G, Garlan F, Garrigou S, et al. Characterization of plasma cell-free DNA integrity using droplet-based digital PCR: toward the development of circulating tumor DNA-dedicated assays. Front Oncol. 2021;11:639675. doi: 10.3389/fonc.2021.639675
- Cirillo M, Craig AF, Borchmann S, et al. Liquid biopsy in lymphoma: Molecular methods and clinical applications. Cancer Treat Rev. 2020;91:102106. doi: 10.1016/j.ctrv.2020.102106
- Arzuaga-Mendez J, Prieto-Fernández E, Lopez-Lopez E, et al. Cell-free DNA as a biomarker in diffuse large B-cell lymphoma: A systematic review. Crit Rev Oncol Hematol. 2019;139:7–15. doi: 10.1016/j.critrevonc.2019.04.013
- Cheng J, Holland-Letz T, Wallwiener M, et al. Circulating free DNA integrity and concentration as independent prognostic markers in metastatic breast cancer. Breast Cancer Res Treat. 2018;169(1):69–82. doi: 10.1007/s10549-018-4666-5
- Zonta E, Nizard P, Taly V. Assessment of DNA integrity, applications for cancer research. Adv Clin Chem. 2015;70:197–246.
- Larsen PA, Hunnicutt KE, Larsen RJ, et al. Warning SINEs: Alu elements, evolution of the human brain, and the spectrum of neurological disease. Chromosome Res. 2018;26(1–2):93–111. doi: 10.1007/s10577-018-9573-4
- Cheng J, Tang Q, Cao X, et al. Cell-free circulating DNA integrity based on peripheral blood as a biomarker for diagnosis of cancer: a systematic review. Cancer Epidemiol Biomarkers Prev. 2017;26(11):1595–1602. doi: 10.1158/1055-9965.EPI-17-0502
- Buteau JP, Seymour JF, Hofman MS. The evolving definition of bulky disease for lymphoma. Leukemia Lymphoma. 2020;61(7):1525–1528. doi: 10.1080/10428194.2020.1797014
- Hohaus S, Giachelia M, Massini G, et al. Cell-free circulating DNA in Hodgkin’s and non-Hodgkin’s lymphomas. Annals Oncology. 2009;20(8):1408–1413. doi: 10.1093/annonc/mdp006
- Rivas-Delgado A, Nadeu F, Enjuanes A, et al. Mutational landscape and tumor burden assessed by cell-free DNA in diffuse large B-cell lymphoma in a population-based study. Clin Cancer Res. 2021;27(2):513–521. doi: 10.1158/1078-0432.CCR-20-2558
- Madhavan D, Wallwiener M, Bents K, et al. Plasma DNA integrity as a biomarker for primary and metastatic breast cancer and potential marker for early diagnosis. Breast Cancer Res Treat. 2014;146(1):163–174. doi: 10.1007/s10549-014-2946-2
- Bortul M, Giudici F, Tierno D, et al. A case–control study by ddPCR of ALU 260/111 and LINE-1 266/97 copy number ratio in circulating cell-free DNA in plasma revealed LINE-1 266/97 as a potential biomarker for early breast cancer detection. Int J Mol Sci. 2023;24(10):8520. doi: 10.3390/ijms24108520
- Kumar S, Nadda N, Paul S, et al. Evaluation of the cell-free DNA integrity index as a liquid biopsy marker to differentiate hepatocellular carcinoma from chronic liver disease. Front Mol Biosci. 2022;9:1024193. doi: 10.3389/fmolb.2022.1024193
- Elhelaly R, Effat N, Hegazy M-F, et al. Circulating cell free DNA and DNA integrity index as discriminating tools between breast cancer and benign breast disease. Asian Pac J Cancer Prev: APJCP. 2022;23(2):545. doi: 10.31557/APJCP.2022.23.2.545
- Bronkhorst AJ, Aucamp J, Pretorius PJ. Cell-free DNA: Preanalytical variables. Clinica Chimica Acta. 2015;450:243–253. doi: 10.1016/j.cca.2015.08.028
- El Messaoudi S, Rolet F, Mouliere F, et al. Circulating cell free DNA: Preanalytical considerations. Clinica Chimica Acta. 2013;424:222–230. doi: 10.1016/j.cca.2013.05.022
- Yu Z, Qin S, Wang H. Alter circulating cell‐free DNA variables in plasma of ovarian cancer patients. J Obstetrics Gynaecol Res. 2019;45(11):2237–2242. doi: 10.1111/jog.14102
- Giacona MB, Ruben GC, Iczkowski KA, et al. Cell-free DNA in human blood plasma: length measurements in patients with pancreatic cancer and healthy controls. Pancreas. 1998;17(1):89–97. doi: 10.1097/00006676-199807000-00012
- Jin Z, El-Deiry WS. Overview of cell death signaling pathways. Cancer Biol Ther. 2005;4(2):147–171. doi: 10.4161/cbt.4.2.1508
- Stroun M, Anker P, Maurice P, et al. Neoplastic characteristics of the DNA found in the plasma of cancer patients. Oncology. 1989;46(5):318–322. doi: 10.1159/000226740
- Lo YD, Han DS, Jiang P, et al. Epigenetics, fragmentomics, and topology of cell-free DNA in liquid biopsies. Science. 2021;372(6538):eaaw3616. doi: 10.1126/science.aaw3616
- Cristiano S, Leal A, Phallen J, et al. Genome-wide cell-free DNA fragmentation in patients with cancer. Nature. 2019;570(7761):385–389. doi: 10.1038/s41586-019-1272-6