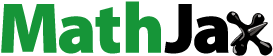
ABSTRACT
Species composition, structure, and regeneration status of trees were assessed in five dry Afromontane forests. In each of the forests, trees having ≥5 cm diameter at breast height were identified and measured in 80 quadrats (20 × 20 m2). We compared tree species composition and similarities, stem densities, frequencies, basal area and importance value index of trees. Harvesting index was calculated to 13.3% in the least disturbed and 22.5% in highly disturbed forests. A total of 1,655 individuals representing 38 species and 28 families were recorded. The tree densities varied from 364.1 to 664.1stem ha−1 with an average basal area of 20.7 m2 ha−1. The analysis showed significant differences in basal areas of tree species between the least and highly disturbed forests. Seedling densities ranged from 2000 to 8162.5 stem ha−1 and sapling densities ranged from 512.5 to 2562.5 stem ha−1. The anthropogenic disturbances revealed high degradation of population structure and regeneration statutes of the trees in the studied forest ecosystem. In conclusion, the study provided empirical results on the dominance, population structure, importance value and regeneration status of tree species, which would be instrumental for the development of successful rehabilitation strategies for the studied forests.
Introduction
Studies on population structure and density of major canopy tree species can help to understand the status of regeneration of species as well as management history and ecology of the forest (Harper Citation1977; Tesfaye et al. Citation2010). Plant population structure shows whether or not the population has a stable distribution that allows continuous regeneration to take place. If regeneration was taking place continuously, then the distribution of species cohorts would show reverse J shape curve, which is an indicator of healthy/good regeneration (Harper Citation1977; Silvertown Citation1982; Teketay Citation2005). Such population structures are common in natural forests where external disturbances are limited (Teketay Citation2005; Tesfaye et al. Citation2010).
Regeneration is a central component of forest ecosystem dynamics and restoration of degraded forest lands. Sustainable forest utilization is only possible if adequate information on the regeneration dynamics and factors influencing important canopy tree species is available (Tesfaye et al. Citation2010). Tropical forests revealed variation in patterns of regeneration both through differences in their constituent species and the environmental variables in which they grow (Whitmore Citation1996; Teketay Citation2005). Seedling densities in the forest under stories are dynamic and rates may vary among species and in gap and shade environments (Bazzaz Citation1991). The rates also vary due to mortality, which could include abiotic stresses such as light, drought, and biotic factors that include herbivory, disease, or competition (Augspurger Citation1984). Information on tree seedling ecology can provide options for forest development through improvement in recruitment, establishment, and growth of the desired species (Swaine Citation1996). Thus, regeneration studies have significant implications on the management, conservation, and restoration of degraded natural forests (Tesfaye et al. Citation2010).
Production of seeds from adult trees, seedling survival, and growth into saplings are also ecological characteristics of forests that determine successful regeneration in the forests (Khumbongmayum, Khan, and Tripathi Citation2006; Saikia and Khan Citation2013). Tree species are fundamental to the overall maintenance of forest biodiversity because trees provide resources and habitats for almost all other forest species (Rahman et al. Citation2011). Inventory of tree species that provides information on diversity helps to identify ecologically useful species and species of special concern that results from deforestation and forest degradation. It is also useful to identify conservation efforts for sustainability of forest biodiversity and represent an important tool to maximize biodiversity conservation. Abiotic factors, disturbance, and competition might result in variations of regeneration status of trees, causing differences in the floristic and structural composition of plant communities (Mori and Takeda Citation2004).
Tropical forests naturally regenerate under influence of abiotic, anthropogenic and biotic factors (Mori and Takeda Citation2004). These factors influence the species composition of tropical forests at different spatial scales (Peña‐Claros et al. Citation2012). The abiotic factors, e.g., precipitation, temperature, availability of resources, such as light and nutrients, affect primary production (Vargas-Rodriguez, Vázquez-García, and Williamson Citation2005). Natural and anthropogenic disturbances strongly influence regeneration at the regional and local scales in forests (Sheil and Burslem Citation2003) because both forms of disturbance alter environmental conditions, ecosystem processes, nutrient availability and plant–plant interactions (Winter Citation2012; Sheil and Burslem Citation2003). The biotic factors, on the other hand, operate through competition for available resources. The capacity of a species to exclude other species from its niche depends on its status within a competitive hierarchy.
Stand structure assists to understand forest ecosystems and biodiversity (Su et al. Citation2010). Assessment of the composition, structure and regeneration status of species is required to ascertain the successful conservation and management of forest resources (Teketay Citation2005; Mishra et al. Citation2013). The assessment can be carried out using age structure and height class distribution for seedlings and sapling. The evaluation of composition and stand structures has been used in assessing long-term changes and restoration of conditions of forests (Smith and Nichols Citation2005) to determine plant diversity, and protect threatened and economic species as well as for monitoring plant communities (Ssegawa and Nkuutu Citation2006). Studies on natural regeneration are also important to understand the forest dynamics and structure that help in the management and monitoring of environmental changes. They do not only show the status but also provide clues about the possible changes in species composition in forests (Sharma et al. Citation2014).
Many studies have shown the relationship between vegetation attributes and topography, particularly slope, aspect and elevation (Soromessa, Teketay, and Demissew Citation2004; Tesfaye et al. Citation2010; Aynekulu et al. Citation2012; Kebede et al. Citation2013), soils (Soromessa, Teketay, and Demissew Citation2004) and anthropogenic disturbances (Tesfaye et al. Citation2010) in the forests of Ethiopia. Although such evidences for the influence of ecological factors on vegetation attributes have been gathered over the years, much of this understanding has come from studies conducted in protected forests. The present study focused on five fragmented forests that are intersected by agricultural and grazing lands as well as settlements in Awi Zone of northwestern Ethiopia. Historically, agricultural activities were the main causes of forest cover decline during the Transitional Government of Ethiopia (1991–1994) because local communities depended on the forests to support mixed agricultural activities and rearing of livestock (Awi Zone Agricultural Office, unpublished). After 1994, the forest areas were protected from agricultural activities, and were designated as state forests, which are protected and managed by the Amhara Regional State of Ethiopia. There is a lack of understanding of how the type of disturbances (severity and intensity) affects stand structure and regeneration in dry Afromontane forests. Therefore, the objective of the study was to assess the impacts of anthropogenic disturbances and topographic factors on composition, stand structure, and regeneration status of trees species in five dry Afromontane fragmented forests.
Materials and methods
Study area
The study was conducted in Awi Zone of Amhara National Regional State (ANRS), northwestern Ethiopia (). It is located at 10°52ʹ to 11° 3ʹ N and 36° 38ʹ to 37° 8ʹ E. The four forest sites (Apini, Bari, Dabkuli and Kahtasa) and one forest site (Tsahare Kan) are located in Banja and Guangua districts, respectively. Among different administration districts in the Zone, Guangua and Banja districts were selected for their representativeness of the districts’ vegetation, and since they are among the least studied natural forest sites. The total area of these forests is 3,188 ha, and Dabkuli and Tsahare Kan forests cover the lowest and highest forest areas, respectively (). The four forests are state forests, which are protected and managed by the regional government, while Tsahare Kan forest is managed by the communities since the last 15 years. The forests are still under heavy pressure due to free grazing and illegal harvesting of tee species. The soils of the northwestern highlands of the country are largely developed from parent materials of volcanic origin, mainly, reddish or brown in color, draining freely and are of medium to heavy texture. The study areas have unimodal pattern of weather condition with annual precipitation reaching 2241 mm and average temperatures of 18.7°C at “Banja” District ()), and 1679 mm rainfall and an average temperature of 20.8°C at “Guangua” District with maximum precipitation occurring from June to October ().
Figure 2. Mean annual rainfall and temperature registered at Kidamaja (1987–2014) (A) and Chagni (1986–2016) (B) weather stations

Table 1. Locations and topographic characteristic of studied forests
Data collection
The vegetation data were collected in the study forests from January to May 2017. Systematic sampling design, with random starting points, was adopted to collect vegetation data (Kent Citation2011). A total of 19 parallel transect lines, 500 m apart from each other, were used in each of the forests. Quadrats, measuring 20 × 20 m2, were also placed 50 m away from forest margins to avoid edge effects related to degrees of forest management and disturbances. The first quadrat was laid down randomly on the top, and the subsequent quadrats were established at 50 m intervals along the transect lines. In this study, seedlings are defined as woody plants with a height less than 130 cm, saplings as woody plants with heights >130 cm and diameter at breast height (dbh) <5 cm, and adult trees as plants with dbh ≥ 5 cm. A total of 80 main quadrats were used in each of the study forests. In each of the main quadrats, diameter and height of all woody species, with a diameter at breast height (dbh) greater than 5 cm, were measured using diameter tape and clinometers, respectively. When the branching of multi-stemmed individuals occurred below the dbh, the dbh of each stem was measured separately. The identity and abundance of seedlings and saplings were identified and counted from two subplots (5 x 5 m2), i.e., 160 subplots, in the main quadrats. The cover of herbaceous species was estimated in five subplots (1 x 1 m2), i.e., 400 subplots, in the four corners and center of the main quadrats. The following herbaceous percent cover-abundance classes were used: 1 (<0.5%), 2 (0.5–1.5%), 3 (1.5–3%), 4 (3–5%), 5 (5–12.5%), 6 (12.5–25%), 7(25–50%), 8 (50–75%) and 9 (75–100%) (Van Der Maarel and Franklin Citation2012). The site characteristics, suc
h as elevation (m), slope (%), aspect, grazing intensity, and canopy openness were measured and recorded. Topographic elevation and slope were measured using Garmin GPS and clinometers, respectively. To determine light availability, canopy openness was measured using densiometer positioned in the center of each quadrat. Grazing intensity was measured using the presence – absence method. This means that if the signs of livestock browsing and feeding of branches or the whole species were present in the quadrats, it was scaled as one, and if all livestock activities were absent in the quadrats, it was scaled as zero. The number of stumps from illegally harvested tree species was counted and recorded in the main quadrat of forests.
Identification and nomenclature of plants
Plants were identified in the field using the published volumes of the Flora of Ethiopia as well as Flora of Ethiopia and Eritrea. For those difficult to identify in the field, specimens were collected, processed and identified through comparing them with already identified specimens deposited in the National Herbarium (ETH) of Ethiopia. Nomenclature of plants in this article follows those published in the Flora of Ethiopia as well as Flora of Ethiopia and Eritrea (Hedberg and Edwards Citation1989, Citation1995; Edwards, Tadesse, and Hedberg Citation1995, Citation1997, Citation2000).
Statistical analyses
Data were entered in an excel spreadsheet and performed in the statistical software package R. Before performing the statistical analyses, data were subjected to a Shapiro-Wilk normality test. Species richness (SR) was determined as a total number of species in each forest. The data on stem density, frequency, dominance and importance value index (IVI) were analyzed. Population structure was analyzed by comparing the distribution of diameter classes. The dominance of tree species, with stem diameter at breast height (dbh) ≥5 cm, was determined from the estimation of their basal area (BA). Basal area (BA) was calculated using the following equation that converts the dbh in cm to the basal area in m2 (Husch, Beers, and Kershaw Citation2003). The mean dominance of each species was then computed by converting the total basal area of individuals of tree species to equivalent basal area per hectare.
The Importance Values Index (IVI), which indicates the ecological importance of a tree species (Husch, Beers, and Kershaw Citation2003), was determined using the following equation:
where RD = the relative density of species, RF = relative frequency of tree species and RDo = the relative dominance of species.
The similarity in tree species composition of the five forests was computed by using Jaccard’s Similarity Coefficient (Magurran Citation2004) as follows:
where SJ = Jaccard’s Similarity Coefficient; a = number of tree species common to sites A and B, b = number of tree species recorded only the site A and c = number of tree species recorded only in site B. The values of SJ range between 0 and 1, 0 indicates complete dissimilarity and 1 indicates complete similarity in species composition.
The regeneration status of species was determined based on the population size of seedlings and saplings (Chauhan et al. Citation2008b) as good regeneration if seedlings >saplings >adults, and fair regeneration if seedlings >or ≤ saplings ≤ adults. Also, the regeneration status was considered as poor if the species is represented by only saplings (saplings ≤or ≥adults) and as not regenerating when a species is represented by only trees. Variation of adult, sapling, seedling densities and basal area was determined by analysis of variance (ANOVA). Harvesting Index (HI) was calculated following the modified method by Sagar, Raghubanshi, and Singh (Citation2003) and derived from the relative densities of stumps of tree species. The relative density of stumps was calculated as the sum of the density of stumps divided by the total density (stumps + live trees). In this study, stumps are defined as a small part of stem remains after cutting down of trees reaching a minimum diameter of ≥5 cm. Correlation and regression analyses were used to analyze the status of natural regeneration in response to site characteristic (elevation, slope, grazing intensity, harvesting index, canopy openness and proportion of herbaceous cover).
Results
Species composition
A total of 1,655 individuals with stem diameter at breast height (dbh) of≥5 cm representing38 tree species and 28 families were recorded in all the studied forests (–). Rubiaceae was the most species-rich family with four species (10.53%), followed by Fabaceae, which contained three species (7.89%). Euphorbiaceae, Loganiaceae, Meliaceae, and Rosaceae were equally represented by two species (21.05%). The remaining 23 families were represented by a single species each. Out of these, 16 species belonging to 16 genera, 15 families, and 431 individual trees were recorded in Tsahare Kan forest (); 17 families representing 21 genera, 21 species and 278 individual trees were recorded in Apini forest (); 20 species belonging to 20 genera, 16 families, and 232 individual trees were recorded in Dabkuli forest (); 16 families comprising 18 genera, 18 species, and 397 individual trees were recorded in Bari forest (); and Kahatsa forest was represented by 317 individual trees, belonging to 23 species, 23 genera, and 22 families ().
Table 3. Stand structure and importance value of species (in descending order of density) in Tsahare Kan forest (N = 16). For all forests in Tables (3–), N = number of quadrats, D = density (stem ha−1), DO = dominance (m 2 ha−1), Fr = frequency, RD = Relative density, RDO = Relative dominance, RF = relative frequency, IVI = importance value index
Table 4. Stand structure and importance value of species (in descending order of density) in Apini forest N = 16)
Table 5. Stand structure and importance value of species (in descending order of density) in Dabkuli forest (N = 16)
Table 6. Stand structure and importance value of species (in descending order of density) in Bari forest (N = 16)
Table 7. Stand structure and importance value of species (in descending order of density) in Kahatsa forest (N = 16)
Density, frequency, and dominance
The density, frequency, and dominance varied among forests (–). The highest tree density was observed in Tsahare Kan forest (664.1stem ha−1) (), whereas the lowest tree density was observed in Dabkuli forest (364.1 stem ha−1) (), and the difference was significant (F = 15.42, df = 30, P = 0.0005). Similar, the tree densities were significantly different (P < 0.05) in the least disturbed Bari () and highly disturbed Kahtasa () forests (F = 4.20, df = 30, P = 0.049) (). The densities of trees did not show a significant difference between Dabkuli and Apini forests. The four species with the highest density were Albizia gummifera, Prunus africana, Apodytes dimidiata and Croton macrostachyus in all the studied forests (–). Specifically, A. gummifera (375.0 stem ha−1) followed by C. macrostachyus (71.8 stem ha−1) accounted for the highest densities and Ehretia cymosa had the lowest density (1.6 stem ha−1) in Tsahare Kan forest ().
Table 2. Stand attributes of trees and analysis of variance in five forests
The dominance ranged from 16.2 to 30.2 m2 ha−1, with the lowest and highest values recorded in highly harvested regime in Kahatsa and least harvested regime in Bari forests, respectively (–). The mean dominance of the five forests was 20.7 ± 2.5 m2 ha−1. The ANOVA showed that the dominance was significantly different (P < 0.05) in the Kahtasa and Bari forests (F = 6.60, P = 0.015) (). The dominance among Tsahare Kan, Apini and Dabkuli did not show significant difference (F = 0.21, P = 0.81). Similarly, the dominance of these forests and Kahatsa forest did not show a significant difference (F = 2.39, P = 0.08). Albizia gummifera (71.1%) followed by S. guineense (10.1%) exhibited the highest dominance value while E. cymosa and L. volkensii had very low dominance values (0.01%, each) in Tsahare Kan forest.
Albizia gummifera (29.1%) followed by P. africana (26.1%) had the highest dominance values, and C. malosana, E. cymosa, G. saxifraga, G. ferruginea, L. volkensii, P. oliveriana exhibited very low values of dominance (0.01%, each) in Apini forest. In Dabkuli forest, A. gummifera (26.6%) and P. africana (18.4%) accounted for the highest dominance values, and B. abyssinica, B. micrantha, B. polystachya, G. ferruginea, M. ferruginea, O. capensis, P. oliveriana, and R. neglecta exhibited the lowest dominance values (0.01%). Similarly, A. gummifera (34.8%) and P. africana (25.5%) showed the highest dominance values in Bari forest while C. malosana and L. volkensii accounted for the lowest dominance values (0.01%). In the case of Kahtasa forest, A. dimidiata (32.7%) followed by A. gummifera (14.8%) were more dominant than D. abyssinica, H. abyssinica, H. revolutum, M. lanceolata and Mimusops kummel (0.01% each).
Similarly, A. gummifera (168.8 stem ha−1) and C. macrostachyus (54.7 stem ha−1) had the highest densities, and the four species with lowest densities were E. cymosa, Ficus sur, Galiniera saxifraga and Cassipourea malosana (1.6 stem ha−1 each) in Apini forest (). Furthermore, A. gummifera (131.3 stem ha−1) followed by C. macrostachyus (64.1 stem ha−1) accounted for the highest densities and seven species had the lowest densities (1.6 stem ha−1each) in Dabkuli forest (). Albizia gummifera (303.1 stem ha−1) and P. africana (100 stem ha−1) had the highest densities, while Dombeya torrida, Ekebergia capensis, and Schefflera abyssinica exhibited the lowest densities (1.6 stem ha−1 each) in Bari forest (). Apodytes dimidiata and R. neglecta had the highest densities (65.6stem ha−1 each), while Ficus sur, Hagenia abyssinica, and Hypericum revolutum exhibited the lowest densities (1.6 stem ha−1 each) in Kahtasa forest ().
The frequency values of the tree species ranged from 1.1% to 19.8% in each forest (–). Similar to density, A. gummifera, A. dimidiata, and P. africana were the most frequently found species in the studied forests. A. gummifera (19.7%) and T. nobilis (14.8%) appeared most frequently while Dracaena steudneri, E. cymosa, F. sur, and Syzygium guineense rarely occurred (1.2% each) in Tsahare Kan forest. A. gummifera (16.8%), Celtis africana (11.6%), and C. macrostachyus (11.6%) exhibited the highest frequency values in Apini forest. But, B. polystachya, C. malosana, E. cymosa, G. saxifrage, G. ferruginea, and P. oliveriana were found rarely (1.1% each) in Apini forest. In Dabkuli forest, A. gummifera (18.9%) and L. volkensii (14.9%) had the highest frequency values, while eleven species exhibited low-frequency values (1.4% each). Albizia gummifera (14.7%) and P. africana (12.0%) had the highest frequency values, but each of Ekebergia capensis, F. sur, Schefflera abyssinica, and D. torrida had the lowest frequency values (1.3%) in Bari forest. In Kahatsa forest, A. dimidiata (12.6%) and R. neglecta (9.5%) occurred most frequently while eight species had the lowest frequency values (1.1% each).
Importance value index
The importance value index (IVI) of tree species varied greatly, ranging from 1.4% to 147.1% among forests (‒7). The IVI revealed that 12.5%, 19%, 20%, 16.7%, and 13% of the tree species in Tsahare Kan, Apini, Dabkuli, Bari, and Kahatsa forests, respectively, had high IVI (≥25%). In contrast, 25%, 27.8%, 34.8%, 40%, and 42.8% of the tree species in Tsahare Kan, Bari, Kahtasa, Dabkuli, and Apini forests, respectively, exhibited low IVI (<5%). The remaining species in each forest had IVI ranging from 5-25%. Albizia gummifera, A. abyssinicus, A. dimidiata, C. macrostachus, P. africana, T. nobilis, and L. volkensii were the top most important species in terms of their IVI among those tree species common to the five studied forests (–). The IVI of the tree species in Tsahare Kan forest suggested that A. gummifera (IVI = 147.1%) and E. cymosa (IVI = 1.4%) had the highest and lowest IVI, respectively. In Apini forest, A. gummifera (IVI = 84.7%) accounted for the highest IVI and C. malosana, E. cymosa and G. saxifrage had the lowest IVI (1.4%, each). In Dabkuli forest, A. gummifera (IVI = 81.5%) exhibited the highest IVI, while B. micrantha and P. oliveriana (IVI = 1.4% each) scored the lowest IVI. Albizia gummifera (IVI = 98.3%) followed by P. africana (IVI = 53.6%) accounted for the highest and D.torrida (IVI = 1.6%) for the lowest IVI in Bari forest. In Kahatsa forest, A. dimidiata (IVI = 58.6%), and A. gummifera (IVI = 35.9%) had the highest IVI, and D. abyssinica, H. abyssinica, H. revolutum, and M. kummel exhibited the lowest importance value (IVI = 1.4%, each).
Similarity in species composition
The highest similarity in tree species composition was 64% among Tsahare Kan and Dabkuli, Tsahare Kan and Bari as well as Apini and Dabkuli forests while the lowest similarity was 31% between Tsahare Kan and Kahatsa forests (). Tsahare Kan and Apini, as well as Dabkuli and Bari, had tree species composition similarity of 52% each.
Table 8. Similarity of species composition among forests
Population structure and regeneration status
Density-diameter distribution exhibited decreasing toward higher diameter classes (). The population structure of tree species recorded in the studied forests exhibited four general diameter class distribution patterns ().
The first pattern was composed of species that exhibited a higher number of individuals at the lowest diameter class and progressively declining numbers with increasing diameter classes. This pattern was illustrated by A. gummifera in all forests ()). In addition to this, it was illustrated by Teclea nobilis in Dabkuli and Rothmannia urcelliformis in Apini forests.
The second pattern was formed by species with an irregular J shape population pattern (). This pattern was illustrated by: (i) C. macrostachyus and M. ferruginea in Tsahare Kan forest; (ii) C. macrostachyus, C. africana and M. ferruginea in Apini forest; (iii) C. macrostachyus, Ehretia cymosa and L. volkensii in Dabkuli forest; (iv) B. abyssinica in Bari forest; and (v) B. abyssinica, C. macrostachyus and P. africana in Kahtasa forest.
The third pattern was bell-shape, which showed that the number of individuals were high in the middle classes and decreased toward the lower and higher diameter classes (). This pattern was illustrated by: (i) A. abyssinicus in Dabkuli forest; (ii) C. macrostachus and P. africana in Bari forest; and (iii) R. neglecta and O. capensis in Kahtasa forest, but such pattern was not illustrated by species in Tsahare Kan and Apini forests.
The fourth pattern consisted of species with a low number of individuals at the lowest diameter class and progressively increasing numbers with increasing diameter classes (). This pattern was illustrated by only A. dimidiata in Kahtasa forest, but such pattern was not observed by species in other forests.
In addition to population structure, the regeneration patterns varied in each forest. Seventy species (44.7%) among seedlings and 20 species (52.6%) among saplings belonging to 16 and 17 families, respectively, were found in all forests (–). Regeneration status of the forest was examined by comparing different attributes (adult density) with the regenerating populations (seedlings and saplings) of species. The seedling density (2000 stem ha−1) in Kahtasa forest was four times lower than Dabkuli forest (8162.5 stem ha−1). The analysis of variance (ANOVA) of log-transformed seedling density between Kahatsa and Dabkuli forests showed statistically significant differences (F = 15.15, P = 0.0006). Similarly, sapling density in Kahtasa forest (512.5 stem ha−1) was five times lower than the density of Tsahare Kan forest (2562.5 stem ha−1) and the difference was statistically significant (F = 23.34, P = 0.0001). The sapling density of Bari forest was also approximately three times higher than Kahatsa forest and the difference was also statistically significant (F = 7.06, P = 0.012). Only a few species had shown regeneration in all the studied forests (–).
Table 10. Natural regeneration status of species (in descending order of density) in Apini forest
Table 11. Natural regeneration status of species (in descending order of density) in Dabkuli forest
Table 12. Natural regeneration status of species (in descending order of density) in Bari forest
Table 9. Natural regeneration status of species in five forests. In all forests in Tables (9–13): D = adult density, SD = seedling density, and SP = sapling density (Stem ha−1), regeneration status (RS) (G = Good, F = Fair, and P = Poor) and ‘0ʹ refers to absence of individuals in one or two of the stages. Natural regeneration status of species (in descending order of density) in Tsahare Kan forest
Table 13. Natural regeneration status of species (in descending order of density) in Kahtasa forest
The densities of seedlings were higher than those of saplings and adults in the studied forests, thus, exhibiting overall good regeneration. Accordingly, 12.5% in Tsahare Kan, 23.8% in Apini, 25% in Dabkuli, 11.1% in Bari, and 17.4% of species in Kahtasa forests showed good regeneration. Also, 43.8% in Tsahare kan, 23.8% in Apini, 10% in Dabkuli, 55.6% in Bari and 26.1% of species in Kahtasa forest showed fair regeneration (–). The dominant tree species, A. gummifera exhibited a good regeneration status in all of the forests where it had a maximum seedling density (3187.5 stem ha−1) with highest sapling and adult densities of 1075 and 375 stem ha−1, respectively, in Tsahare Kan forest. Prunus africana exhibited relatively good regular regeneration in all the studied forests except Tsahare Kan. Fifteen species (39.47%), namely Acacia abyssinica, Bridelia micrantha, Dovyalis abyssinica, Dracaena steudneri, Ekebergia capensis, Galiniera saxifraga, Hagenia abyssinica, Hypericum revolutum, Juniperus procera, Maesa lanceolata, Maytenus senegalensis, Nuxia congesta, Pavetta oliveriana, Schefflera abyssinica and Vernonia amygdalina did not have seedlings and saplings in all the studied forests, suggesting hampered natural regeneration.
Correlation between natural regeneration of trees and site factors
Pearson correlation (r) analysis showed that site factors exhibited both positive and negative relationship with the densities of seedlings and saplings (). Elevation and grazing intensity exhibited a negative relationship with both seedlings and sapling densities. The correlation coefficient between the density of seedling and elevation was negative (r = −0.20, P = 0.081). In contrast, slope had a significant positive relationship with the density of seedlings (r = 0.27, P = 0.015). Grazing intensity (r = −0.31, P = 0.005) and harvesting index (r = −0.25, P = 0.026) had significant negative relationships with sapling density. The harvesting index also exhibited a strong negative relationship with the density of adult trees (r = −0.56, P = 0.001). The abundance of the herbaceous cover showed a negative correlation (r = −0.15, P = 0.172) with the density of seedlings.
Table 14. Pearson correlation matrix in the density of seedlings, saplings, and adult species (density, stem ha−1, bold fonts referred to significant at P < 0.05)
The correlation of densities of seedlings and saplings with topographic factors indicated that elevation and slope were the two most important factors affecting regeneration (). The regression analysis showed that the densities of seedlings of all species exhibited quadratic decline pattern along increasing elevation (R2 = 0.11, P = 0.001) () and slope gradient (R2 = 0.12, P < 0.05) (). The densities of saplings of all species declined significantly with increasing elevation (R2 = 0.07, P = 0.017) (). The regression analyses also revealed that the seedling density of A. gummifera declined significantly along increasing elevation (R2 = 0.21, P < 0.05) ().
Discussion
Species composition and stand structures
The result revealed that the five studied forests contain different tree species, which fulfill critical ecological, e.g., provision of habitats for other plants and wild animals, as well as economic functions. The species composition recorded in the studied forests was comparable to those of another dry Afromontane forest of Ethiopia (36 species) (Girma and Mosandl Citation2012) and a mopane woodland in northern Botswana (35 species) (Teketay et al. Citation2018). However, it was lower than tropical forests (55 species) of Kenya (Mutiso et al. Citation2015). The variations of species richness of adult trees remained insignificant among forests, but the numbers of species of adult tree species were much higher than saplings and seedlings. This suggests that some of the tree species exhibit hampered regeneration. The degrees of harvesting in the forests were the main source of variations in species composition. For instance, 60.8% of adult species in Kahatsa forest did not have either saplings or seedlings. In contrast, 22.2% of adult species did not have seedlings and saplings. Also, 43.7%, 42.8%, and 40% of adult species in Tsahare Kan, Apini and Dabkuli forests, respectively, did not have saplings and seedlings. Therefore, human interventions are required to reduce the effects of disturbances, maintain the integrity of the forests as well as enhance their composition and structure.
The similarity of the studied forests in their species composition ranged from 31% to 64%, indicating that some of the tree species are commonly found in the forests. The lowest similarity in species composition was found between Tsahare Kan and Kahatsa forests, which may be attributed to the differences in their elevation gradients and levels of disturbance. Conversely, the small variations in their topography and levels of disturbance might have contributed to the high similarity of Apini and Dabkuli forests in their species composition. A recent study also reported that Jaccard dissimilarity of species composition increased significantly with increasing elevation distance (Haider et al. Citation2018).
The stem densities varied with species, diameter size classes, and forests. The stand densities of the tree species in the five forests, which ranged from 365 to 664 stems ha−1, are lower than those reported from Munessa-Shashemene forest (734 stems ha−1) (Abebe and Mosandl Citation2012), and Wof-Washa forest (698.8 stems ha−1) (Fisaha, Hundera, and Dalle Citation2013) in Ethiopia and a moist tropical forest (843 stems ha−1) (Gautam, Manhas, and Tripathi Citation2016). However, the density of Kahtasa forest (505 stems ha−1) was comparable to the tree density reported from other tropical forests (515 stems ha−1) (Chauhan et al. Citation2008a) and higher than a disturbed forest in northern India (290 stems ha−1) (Sharma and Chaudhry Citation2018). The variation of tree densities in the studied forests was due to variations in elevation, slope, aspect and disturbance levels. Similarly, the previous study reported that the altitudinal variation, species composition, age structure (Ademoh, Muoghalu, and Onwumere Citation2017) and degree of disturbance (Sharma and Chaudhry Citation2018) contributed to variation in densities of tree species in forests. Like adult tree densities, densities of seedlings and saplings varied among species and forests due to topographical variations and levels of disturbance. For instance, at higher elevations and disturbance levels, Kahatsa forest exhibited lower seedling and sapling densities than at lower elevations and disturbance levels in Tsahare Kan forest. Thus, increased levels of disturbance and grazing intensity might have contributed to the reduction of seed sources, leading to lower densities of seedlings and saplings and, hence, hampered regeneration in Kahtasa forest. In contrast, Bari forest supported higher densities of seedlings and saplings than the other studied forests due to the lower incidences of disturbance.
The dominance, which is dependent on the size of trees, also varied among the studied forests. The heavy harvesting of large species affected the structure, resulting in variation of the dominance of trees in the forests. The total dominance range (16.2 to 30.2 m2 ha−1) in the five forests was close to the range of dominance (17 to 40 m2 ha−1) reported for dry forests across the world (Murphy and Lugo Citation1986). However, it was much lower than those recorded in Manna (94.2 m2 ha−1) (Lulekal et al. Citation2008) and Taragedam (115 m2 ha−1) (Zegeye, Teketay, and Kelbessa Citation2011) forests. This variation of the dominance of tree species in the forests was due to the variations in the levels of disturbance. The increase in dominance from 16.2 m2 ha−1 in Kahtasa to 30.2 m2 ha−1 in Bari forest was because of a high number of individuals in the high diameter size classes and the lower incidences of disturbance than the other forests. In contrast, the dominance of tree species in Tsahare Kan, Apini and Dabkuli forests exhibited small variation. This might be due to similar levels of disturbance, particularly illegal harvesting of the tree species.
A. gummifera, P. africana, and A. dimidiata ranked first in the dominance of tree species in all forests due to their large stem diameter. However, the abundance of these pioneer species declined in disturbed Kahatsa forest. Similarly, these species had the highest IVI in all the studied forests, reflecting their relatively high ecological importance. Kahatsa, Dabkuli and Apini forests had many species with low IVI and relatively lower densities of trees due to higher incidences of disturbance than the other forests. Low numbers may just result because of these species are rare or their population size is small (Henle et al. Citation2004). Therefore, human intervention, e.g., in the form of enrichment planting, is required for the conservation and management of those tree species to maintain and enhance their ecological and economic importance. In addition to dominant species, T. nobilis also showed good regeneration except in Bari forest. Lepidotrichilia volkensii had a maximum seedling density (1400 stems ha−1) with high sapling and adult densities of 212.5 and 54.7 stem ha−1, respectively, in Dabkuli forest, but sharply declining densities in other forests.
Population structure and regeneration status of species
The population structure and regeneration status varied among the tree species because of poor forest management that has exposed the trees to illegal harvesting. The overall stand densities of the five studied forests exhibited the reverse J-shaped diameter class distribution, suggesting a stable population structure. This is similar to those reported from other forests in Ethiopia (Abebe and Mosandl Citation2012; Birhanu, Bekele, and Demissew Citation2018) and other countries (Sahu, Dhal, and Mohanty Citation2012; Ademoh, Muoghalu, and Onwumere Citation2017; Rawat et al. Citation2018).
The overall numbers of seedlings and sapling varied among forests. Similar to the densities of tree species, a few species accounted for the number of species of seedlings and saplings. This suggests that human disturbance may have influenced seed dispersal mechanisms, fruiting, germination, and regeneration in most species. As a result, some of the tree species failed to naturally regenerate in each of the studied forests. The tree species, which had no seedlings and saplings exhibited discontinuous population structures. Earlier studies also reported that a lack of stand-level regeneration occurred commonly in Ethiopian forests (Girma and Mosandl 2012; Fisaha, Hundera, and Dalle Citation2013) and across tropical forests (Anitha et al. Citation2010). In Kahtasa forest, 15 tree species were represented only by few individuals in the adult stages. Harvesting of trees and livestock grazing/browsing contributed to the reduction or absence of seedlings and saplings at the early stages of the tree species. The absence of seedlings and saplings of tree species indicates the urgent need for targeted forest management plan to enhance natural regeneration.
Ecological factors affecting natural regeneration of species
The correlation analysis showed a negative relation between seedling and sapling densities with elevation. However, the weak negative relation reflects that inherently, multiple factors might be involved in natural regeneration of the forests. The most observed root causes were grazing intensity and harvesting of tree species. This result was consistent with the findings of other tropical forests (Bhat, Negi, and Todaria Citation2015). The topographic slope also played a positive relationship with seedling density, but negative correlation with the density of saplings and adult trees, owing to the difficulty of accessing areas for grazing activities with increasing steepness of the slope. However, steep slopes of forests do not provide stable environment due to loose soil structure that will influence the growth of seedlings to saplings and, then, adult trees. Therefore, loose soil structure on steep slopes of forests might cause mortality of seedlings.
The illegal tree harvesting reduces the stand-level regeneration of species. For instance, the canopy opening exhibited a negative correlation with the densities of seedlings and saplings of tree species. Heavy removal of tree species leads to a reduction of seed sources and stimulate the growth of herbaceous and semi-woody species. It also imposes unfavorable abiotic stress, such as high vapor pressure deficits and high temperatures that restrain regeneration (White Citation2012; Kuijper et al. Citation2010). It was also reported that canopy characteristics of forests affect the species composition, richness, and regeneration of tree species (Lemenih, Gidyelew, and Teketay Citation2004). Natural regeneration may decline in open forests, although previous studies reported that most species had increased densities of seedlings and saplings with increasing area of canopy openings (Vargas-Rodriguez, Vázquez-García, and Williamson Citation2005; Romell Citation2011). This might be due to the properties of shade-tolerant and intolerant species that exhibit variations of regeneration in relation to the degree of canopy openness. Canopy openings might facilitate regeneration of shade-intolerant species and, therefore, had positive effects on seedling recruitment.
Grazing intensity also showed negative impacts on natural regeneration because of browsing or consumption and foot trampling on shoots, leaves or whole of seedlings and saplings. But, with an increase in the steepness of the slope of montane forests, grazing intensity declined. Livestock grazing may, thus, be a threat to the regeneration of tree species on the flatter parts of montane forests. The result is consistent with findings from previous studies that reported grazing to clearly affect the densities of seedlings and saplings (Norden et al. Citation2011; White Citation2012; Kuijper et al. Citation2010). This implies that uncontrolled grazing intensity results in changing the species composition of montane forests. In general, selective harvesting results in creating forest gaps, while grazing intensity removes ground vegetation cover. Both disturbance types, ultimately, resulted in forest degradation.
Several structural attributes influence the regeneration of species, especially the stem density of trees and abundance of herbaceous cover. In this study, the densities of adult trees had a significant repulsion effect on seedlings, suggesting high competition by adult trees causing a strong negative effect on the survival of seedlings. This result concurs with previous findings in tropical forests (Mohammadi, Gholamali, and Theresa Citation2017). In other studies, however, positive correlations were found between densities of seedling and mature trees (Kuijper et al. Citation2010; Darrigo, Venticinque, and Dos Santos Citation2016), indicating that competitive interactions among plant species govern the regeneration process as was reported from other tropical forests (Norden et al. Citation2011).
The biotic and abiotic factors, as well as competition, may not only govern the spatial distribution of adult species but also determine growth phases from seedlings to adult stages. For instance, the herbaceous cover played a negative effect on the spatial distribution of seedlings. The interactions between seedlings and understory vegetation result in forest dynamics because dense understory vegetation decreases light availability near the forest floor and results in declining of seedling regeneration (Montgomery, Reich, and Palik Citation2010). The density of seedlings was reduced in response to high herbaceous cover, indicating competitive effects for space and resources between seedlings with their non-tree competitors. High herbaceous cover played a major role in preventing successful seed germination, seedling establishment, growth and survival (Kobe and Vriesendorp Citation2011).
Conclusions and implication for rehabilitation of the studied forests
The present study showed that tree species in these remnant forests have a potential to support biodiversity. The forests can support other plants and serve as seed sources and offer economic benefits as sources of firewood, construction material and charcoal production for local communities. The abundance and dominance of A. gummifera, A. dimidiata, P. afriana, A. abyssinicus and C. Macrostachyus in the studied forests indicate that the forests could serve as important seed sources for seedling production. Millettia ferruginea and Rhus glutinosa were the endemic species recorded with regenerating individuals in the forest sites. P. africana has been reported as threatened species in Ethiopia and other African countries, but it was recorded as one of the dominant as well as economically and ecologically important species in the studied forests.
The results on the dominance, importance value, composition, and regeneration status of tree species, which would be instrumental for the development of successful rehabilitation and restoration strategies for the studied forests. Some species exhibited considerable densities, population structure, IVI and regeneration in the five studied forests. Diameter class distributions and IVI indicated the harvesting potential of some of the tree species. In contrast, most of the tree species exhibited hampered natural regeneration and, hence, low IVI. The regeneration potential of the majority of tree species was observed to decline and exhibited poor regeneration or no regeneration, which could affect the diversity levels of the studied forests. The grazing intensity and canopy openness created by harvesting of large tree species resulted in declined numbers of seedlings and saplings of the tree species in the forests. These results indicate the urgent need for the development and implementation of a strong forest management plan to rehabilitate and sustain the forests. Therefore, urgent priority is needed to conserve ecologically and economically important tree species before they are locally exterminated. The establishment of area exclosure to prevent cattle grazing and strong regulation systems against illegal woody species harvesting would also enhance natural regeneration and rehabilitation of the studied forests. Further research is required to investigate and identify the factors responsible for the poor regeneration of the tree species in the studied forests.
Acknowledgments
We are grateful to Addis Ababa University (AAU) and Ethiopian Environment and Forestry Research Institute (EERIE) for their financial support. We extend special thanks to Mr. Melaku Wondafrash for his assistance in plant identification. Finally, we are thankful to the two anonymous reviewers for their valuable comments on an earlier version of the manuscript.
Disclosure statement
The authors declare that they have no conflict of interest.
References
- Abebe, G., and R. Mosandl. 2012. “Structure and Potential Regeneration of Degraded Secondary Stands in Munessa-shashemene Forest, Ethiopia.” Journal of Tropical Forest Science 24 (1): 46–53.
- Ademoh, F. O., J. I. Muoghalu, and B. Onwumere. 2017. “Temporal Pattern of Tree Community Dynamics in a Secondary Forest in Southwestern Nigeria, 29 Years after a Ground Fire.” Global Ecology and Conservation 9: 148–170. doi:10.1016/j.gecco.2016.11.005.
- Anitha, K., S. Joseph, R. J. Chandran, E. Ramasamy, and S. N. Prasad. 2010. “Tree Species Diversity and Community Composition in a Human-dominated Tropical Forest of Western Ghats Biodiversity Hotspot, India.” Ecological Complexity 7: 217–224. doi:10.1016/j.ecocom.2010.02.005.
- Augspurger, C. K. 1984. “Pathogen Mortality of Tropical Tree Seedlings: Experimental Studies of the Effects of Dispersal Distance, Seedling Density and Light Conditions.” Oecologia 61: 211–217. doi:10.1007/BF00396763.
- Aynekulu, E., R. Aerts, P. Moonen, M. Denich, K. Gebrehiwot, T.-G. Vågen, W. Mekuria, and H. J. Boehmer. 2012. “Altitudinal Variation and Conservation Priorities of Vegetation along the Great Rift Valley Escarpment, Northern Ethiopia.” Biodiversity and Conservation 21: 2691–2707. doi:10.1007/s10531-012-0328-9.
- Bazzaz, F. 1991. “Regeneration of Tropical Forests: Physiological Responses of Pioneer and Secondary Species.” In Rainforest Regeneration and Management, edited by A. Gomez-pompa, T. Whitmore, and M. Hadley, 91–118. Paris: Parthenon Publishing, UNES.
- Bhat, J. A., A. K. Negi, and N. P. Todaria. 2015. “Regeneration Status of Woody Species in a Protected Area of Western Himalaya.” Acta Ecologica Sinica 35: 51–58. doi:10.1016/j.chnaes.2015.04.006.
- Birhanu, L., T. Bekele, and S. Demissew. 2018. “Woody Species Composition and Structure of Amoro Forest in West Gojjam Zone, North Western Ethiopia.” Journal of Ecology and the Natural Environment 10 (4): 53–64. doi:10.5897/JENE2018.0688.
- Chauhan, D., C. Dhanai, B. Singh, S. Chauhan, N. Todaria, and M. Khalid. 2008a. “Regeneration and Tree Diversity in Natural and Planted Forests in a Terai-Bhabhar Forest in Katarniaghat Wildlife Sanctuary, India.” Tropical Ecology 49: 53–67.
- Chauhan, P., J. Negi, L. Singh, and R. Manhas. 2008b. “Regeneration Status of Sal Forests of Doon Valley.” Annals of Forestry 16: 178–182.
- Darrigo, M. R., E. M. Venticinque, and F. A. M. Dos Santos. 2016. “Effects of Reduced Impact Logging on the Forest Regeneration in the Central Amazonia.” Forest Ecology and Management 360: 52–59. doi:10.1016/j.foreco.2015.10.012.
- Edwards, S., M. Tadesse, and I. Hedberg, eds. 1995. Flora of Ethiopia and Eritrea. Vol. 2 vols. part 2. Addis Ababa: Addis Ababa University. 456.
- Edwards, S., M. Tadesse, and I. Hedberg. 1997. Flora of Ethiopia and Eritrea. Vol. 6 vols. Addis Ababa: Addis Ababa University. 586 .
- Edwards, S., M. Tadesse, and I. Hedberg, 2000. Flora of Ethiopia and Eritrea. Vol. 2 vols part 1. Addis Ababa: Addis Ababa University. 532.
- Fisaha, G., K. Hundera, and G. Dalle. 2013. “Woody Plants’ Diversity, Structural Analysis and Regeneration Status of Wof Washa Natural Forest, North‐east Ethiopia.” African Journal of Ecology 51: 599–608. doi:10.1111/aje.2013.51.issue-4.
- Gautam, M. K., R. K. Manhas, and A. K. Tripathi. 2016. “Overstory Structure and Soil Nutrients Effect on Plant Diversity in Unmanaged Moist Tropical Forest.” Acta Oecologica 75: 43–53. doi:10.1016/j.actao.2016.06.010.
- Girma, A., and R. Mosandl. 2012. Journal of Tropical Forest Science 24(1):46-53.
- Haider, S., C. Kueffer, H. Bruelheide, T. Seipel, J. M. Alexander, L. J. Rew, J. R. Arévalo, L. A. Cavieres, K. L. Mcdougall, and A. Milbau. 2018. “Mountain Roads and Non‐native Species Modify Elevational Patterns of Plant Diversity.” Global Ecology and Biogeography. doi:10.1111/geb.12727.
- Harper, J. L. 1977. Population Biology of Plants. London: Academic press.
- Hedberg, I., and S. Edwards. 1989. Flora of Ethiopia. Vol. 3 vols. AddisAbaba: Addis Ababa University. 659 .
- Hedberg, I., and S. Edwards. 1995. Flora of Ethiopia. Vol. 7 vols. AddisAbaba: Addis Ababa University. 420 .
- Henle, K., K. F. Davies, M. Kleyer, C. Margules, and J. Settele. 2004. “Predictors of Species Sensitivity to Fragmentation.” Biodiversity & Conservation 13: 207–251. doi:10.1023/B:BIOC.0000004319.91643.9e.
- Husch, B., T. Beers, and J. J. Kershaw. 2003. Forest Mensuration. 41h ed. New York: John Wiley & Sons.
- Kebede, M., M. Kanninen, E. Yirdaw, and M. Lemenih. 2013. “Vegetation Structural Characteristics and Topographic Factors in the Remnant Moist Afromontane Forest of Wondo Genet, South Central Ethiopia.” Journal of Forestry Research 24: 419–430. doi:10.1007/s11676-013-0374-5.
- Kent, M. 2011. Vegetation Description and Data Analysis: A Practical Approach. UK: John Wiley & Sons.
- Khumbongmayum, A. D., M. Khan, and R. Tripathi 2006. “Biodiversity Conservation in Sacred Groves of Manipur, Northeast India: Population Structure and Regeneration Status of Woody Species.” Human Exploitation and Biodiversity Conservation Springer 15: 2439–2456.
- Kobe, R. K., and C. F. Vriesendorp. 2011. “Conspecific Density Dependence in Seedlings Varies with Species Shade Tolerance in a Wet Tropical Forest.” Ecology Letters 14: 503–510. doi:10.1111/j.1461-0248.2011.01612.x.
- Kuijper, D. P., J. P. Cromsigt, B. Jędrzejewska, S. Miścicki, M. Churski, W. Jędrzejewski, and I. Kweczlich. 2010. “Bottom‐up versus Top‐down Control of Tree Regeneration in the Białowieża Primeval Forest, Poland.” Journal of Ecology 98: 888–899. doi:10.1111/j.1365-2745.2010.01656.x.
- Lemenih, M., T. Gidyelew, and D. Teketay. 2004. “Effects of Canopy Cover and Understory Environment of Tree Plantations on Richness, Density and Size of Colonizing Woody Species in Southern Ethiopia.” Forest Ecology and Management 194: 1–10. doi:10.1016/j.foreco.2004.01.050.
- Lulekal, E., E. Kelbessa, T. Bekele, and H. Yineger. 2008. “Plant Species Composition and Structure of the Mana Angetu Moist Montane Forest, South-eastern Ethiopia.” Journal of East African Natural History 97: 165–185. doi:10.2982/0012-8317-97.2.165.
- Magurran, A.E. 2004. An Index of Diversity. Measuring Biological Diversity, p.256. Oxford: Blackwell Publishing Company.
- Mishra, A., S. Behera, K. Singh, L. Chaudhary, R. Mishra, and B. Singh. 2013. “Influence of Abiotic Factors on Community Structure of Understory Vegetation in Moist Deciduous Forests of North India.” Forest Science and Practice 15: 261–273. doi:10.1007/s11632-013-0415-3.
- Mohammadi, F., S. Gholamali, and A. Theresa. 2017. “Tree Species Composition, Biodiversity and Regeneration in Response to Catena Shape and Position in a Mountain Forest.” Scandinavian Journal of Forest Research 32 (1): 1-28.
- Montgomery, R. A., P. B. Reich, and B. J. Palik. 2010. “Untangling Positive and Negative Biotic Interactions: Views from above and below Ground in a Forest Ecosystem.” Ecology 91: 3641–3655. doi:10.1890/09-1663.1.
- Mori, A., and H. Takeda. 2004. “Effects of Undisturbed Canopy Structure on Population Structure and Species Coexistence in an Old-growth Subalpine Forest in Central Japan.” Forest Ecology and Management 200: 89–100. doi:10.1016/j.foreco.2004.06.036.
- Murphy, P. G., and A. E. Lugo. 1986. “Ecology of Tropical Dry Forest.” Annual Review of Ecology and Systematics 17: 67–88. doi:10.1146/annurev.es.17.110186.000435.
- Mutiso, F. M., M. J. Mugo, J. Cheboiwo, F. Sang, and G. K. Tarus. 2015. “Floristic Composition, Affinities and Plant Formations in Tropical Forests: A Case Study of Mau Forests in Kenya.” International Journal of Agriculture and Forestry 5: 79–91.
- Norden, N., R. C. Mesquita, T. V. Bentos, R. L. Chazdon, and G. B. Williamson. 2011. “Contrasting Community Compensatory Trends in Alternative Successional Pathways in Central Amazonia.” Oikos 120: 143–151. doi:10.1111/j.1600-0706.2010.18335.x.
- Peña‐Claros, M., L. Poorter, A. Alarcón, G. Blate, U. Choque, T. S. Fredericksen, M. J. Justiniano, C. Leaño, J. C. Licona, and W. Pariona. 2012. “Soil Effects on Forest Structure and Diversity in a Moist and a Dry Tropical Forest.” Biotropica 44: 276–283. doi:10.1111/j.1744-7429.2011.00813.x.
- Rahman, M. H., M. A. S. A. Khan, B. Roy, and M. J. Fardusi. 2011. “Assessment of Natural Regeneration Status and Diversity of Tree Species in the Biodiversity Conservation Areas of Northeastern Bangladesh.” Journal of Forestry Research 22: 551. doi:10.1007/s11676-011-0198-0.
- Rawat, D. S., S. S. Dash, B. K. Sinha, V. Kumar, A. Banerjee, and P. Singh. 2018. “Community Structure and Regeneration Status of Tree Species in Eastern Himalaya: A Case Study from Neora Valley National Park, West Bengal, India.” Taiwania 63 (1): 16-24.
- Romell, E. 2011. Aspects of Regeneration in Tropical Secondary Forests of Northeast Borneo Affected by Selective Logging, El Niño-induced Drought and Fire 57: 1-56.
- Sagar, R., A. Raghubanshi, and J. Singh. 2003. “Tree Species Composition, Dispersion and Diversity along a Disturbance Gradient in a Dry Tropical Forest Region of India.” Forest Ecology and Management 186: 61–71. doi:10.1016/S0378-1127(03)00235-4.
- Sahu, S., N. Dhal, and R. Mohanty. 2012. “Tree Species Diversity, Distribution and Population Structure in a Tropical Dry Deciduous Forest of Malyagiri Hill Ranges, Eastern Ghats, India.” Tropical Ecology 53: 163–168.
- Saikia, P., and M. Khan. 2013. “Population Structure and Regeneration Status of Aquilaria Malaccensis Lam. In Homegardens of Upper Assam, Northeast India.” Tropical Ecology 54: 1–13.
- Sharma, C., A. K. Mishra, O. Prakash, S. Dimri, and P. Baluni. 2014. “Assessment of Forest Structure and Woody Plant Regeneration on Ridge Tops at Upper Bhagirathi Basin in Garhwal Himalaya.” Tropical Plant Research 1: 62–71.
- Sharma, V., and S. Chaudhry. 2018. “Vegetation Composition and Plant Diversity in Mining Disturbed Tropical Thorn Forest of Asola-Bhatti Wildlife Sanctuary, Northern India.” Taiwania 63 (3): 267-280.
- Sheil, D., and D. F. Burslem. 2003. “Disturbing Hypotheses in Tropical Forests.” Trends in Ecology & Evolution 18: 18–26. doi:10.1016/S0169-5347(02)00005-8.
- Silvertown, J. 1982. Introduction to Plant Population Ecology. New York: Longman group.
- Smith, R., and J. Nichols. 2005. “Patterns of Basal Area Increment, Mortality and Recruitment Were Related to Logging Intensity in Subtropical Rainforest in Australia over 35 Years.” Forest Ecology and Management 218: 319–328. doi:10.1016/j.foreco.2005.08.030.
- Soromessa, T., D. Teketay, and S. Demissew. 2004. “Ecological Study of the Vegetation in Gamo Gofa Zone, Southern Ethiopia.” Tropical Ecology 45: 209–222.
- Ssegawa, P., and D. N. Nkuutu. 2006. “Diversity of Vascular Plants on Ssese Islands in Lake Victoria, Central Uganda.” African Journal of Ecology 44: 22–29. doi:10.1111/j.1365-2028.2006.00609.x.
- Su, D., D. Yu, L. Zhou, X. Xie, and Z. Liu. 2010. “Differences in the Structure, Species Composition and Diversity of Primary and Harvested Forests on Changbai Mountain, Northeast China.” Journal of Forest Science 56: 285–293. doi:10.17221/84/2009-JFS.
- Swaine, M. D., ed. 1996. Ecology of Tropical Forest Tree Seedlings. Paris: Parthenon Publishing, UNES.
- Teketay, D. 2005. “Seed and Regeneration Ecology in Dry Afromontane Forests of Ethiopia: I. Seed Production-population Structures.” Tropical Ecology 46: 29–44.
- Teketay, D., K. Kashe, J. Madome, M. Kabelo, J. Neelo, M. Mmusi, and W. Masamba. 2018. “Enhancement of Diversity, Stand Structure and Regeneration of Woody Species through Area Exclosure: The Case of a Mopane Woodland in Northern Botswana.” Ecological Processes 7: 5. doi:10.1186/s13717-018-0116-x.
- Tesfaye, G., D. Teketay, M. Fetene, and E. Beck. 2010. “Regeneration of Seven Indigenous Tree Species in a Dry Afromontane Forest, Southern Ethiopia.” Flora-Morphology, Distribution, Functional Ecology of Plants 205: 135–143. doi:10.1016/j.flora.2008.12.006.
- Van Der Maarel, E., and J. Franklin. 2012. Vegetation Ecology. UK: John Wiley & Sons.
- Vargas-Rodriguez, L. Y., J. A. Vázquez-García, and G. B. Williamson. 2005. “Environmental Correlates of Tree and Seedling–Sapling Distributions in a Mexican Tropical Dry Forest.” Plant Ecology 180: 117–134. doi:10.1007/s11258-005-3026-9.
- White, M. A. 2012. “Long-term Effects of Deer Browsing: Composition, Structure and Productivity in a Northeastern Minnesota Old-growth Forest.” Forest Ecology and Management 269: 222–228. doi:10.1016/j.foreco.2011.12.043.
- Whitmore, T. 1996. “A Review of Some Aspects of Tropical Rainforest Seedling Ecology with Suggestions for Further Enquiry.” In The Ecology of Tropical Forest Tree Seedlings, edited by M. Swaine, 3–9. Paris: Parthenon Publishing.
- Winter, S. 2012. “Forest Naturalness Assessment as a Component of Biodiversity Monitoring and Conservation Management.” Forestry 85: 293–304. doi:10.1093/forestry/cps004.
- Zegeye, H., D. Teketay, and E. Kelbessa. 2011. “Diversity and Regeneration Status of Woody Species in Tara Gedam and Abebaye Forests, Northwestern Ethiopia.” Journal of Forestry Research 22: 315. doi:10.1007/s11676-011-0176-6.