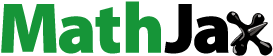
ABSTRACT
Introduction
Sediment and nutrient retention have significant impacts on agricultural productivity and water quality. Obvious changes of climate and land-use have deeply affected freshwater ecosystem services in the last several decades. Nevertheless, it is unclear what the differences are in the impact of climate and land-use changes on freshwater ecosystem services.
Outcomes
The impacts of climate and land-use change on sediment and nutrient retention (two essential freshwater ecosystem services) were evaluated with the help of the Integrated Valuation of Environmental Services and Tradeoffs (InVEST) model. The results from the study area suggest a decreasing trend in sediment and nutrient retention from 1995 to 2015 when climate and land-use were changed simultaneously. A decreasing trend in sediment retention and an increasing trend in nutrient retention were presented under scenario A (climate change from 1995 to 2015 with land-use unchanged), while the sediment exported and nutrient retention exhibited a decreasing trend except sediment retained under scenario B (land-use change from 1995 to 2015 with unchanged climate). Rainfall erosivity and soil erodibility were more sensitive to the output of sediment retention, while retention efficiency produced more of an effect on nutrient retention.
Discussion and Conclusion
Climate change played a dominant role in the change in sediment retention, while land-use change had a more significant effect on nutrient retention change than climate change. The results of this study provide explicitinformation for land-use managers to reduce the negative effects associated with sediment and nutrient retention.
Introduction
The natural ecosystem provides important ecosystem services for human beings (e.g., carbon sequestration, soil retention, biodiversity, climate regulation, and recreation), which are crucial for social and economic development (Costanza et al. Citation1997; Tan et al. Citation2020). Retention of sediment and nutrients is an important freshwater ecosystem service that is deeply affected by natural and human-managed processes (Uwimana et al. Citation2018). The quantities of sediment and retained have serious effects on agricultural productivity and water quality (Lenka et al. Citation2017; Griffiths and Mitsch Citation2020). These services have been degraded in the past several decades (MA Citation2005). Therefore, it is vital to enhance sediment and nutrient retention to control soil erosion and ensure water quality (Hopkins et al. Citation2018).
Land managers can obtain explicit information to reduce damage and costs by learning from the changes in sediment and nutrient retention resulting from climate and land-use change (Fan, Shibata, and Chen Citation2018; Uwimana et al. Citation2018). Previous studies have demonstrated that climate and land-use change has serious effects on sediment and nutrient retention (Su and Fu Citation2013; Fan, Shibata, and Wang Citation2016). Climate change deeply affects the intensity and spatial pattern of soil erosion and nutrient delivery (Bangash et al. Citation2013). For example, the average monthly nutrient load in South Australia decreases due to a decrease in average precipitation under a future climate (Shrestha et al. Citation2017). However, land-use change has an effect on sediment and nutrient retention capacity. The intensity of soil erosion has tended to increase resulting from deforestation and moving from no-till to till agriculture (Skaalsveen, Ingram, and Clarke Citation2019). The soil erosion control service in the Loess Plateau was improved by the Grain-for-Green project (conversion of farmland to forestland and grassland in mountainous region) (Fu et al. Citation2011).
Guizhou Province in China is a typical vulnerable region of the world. This region suffers from serious soil erosion resulting from steep terrain, heavy precipitation, and inappropriate cultivation (Huang et al. Citation2016). The heavy use of chemical fertilizers and pesticides for agricultural production has resulted in significant deterioration of water quality in most catchments in Guizhou Province (Zheng et al. Citation2013). The government set up the Grain-for-Green project and a forest protection program in the late 1990s to reduce the adverse impact of soil erosion and improve water quality (Cheng et al. Citation2016). Built-up land has increased due to rapid economic growth in recent years (Han, Yang, and Zhang Citation2015). There has been a prominent change in the climate element in recent decades in this area under the background of climate warming (Chen et al. Citation2018). These drastic changes in climate and land-use are likely to have deep impacts on soil erosion and nutrient delivery capacity, which is crucially important for sustainable development in this region. Thus, it is necessary to explore the effects of land-use and climate change on variations in sediment and nutrient retention.
Although many scholars have examined the impact of changes in climate and land-use on ecosystem services in many regions of the world (Schirpke et al. Citation2017; Sample, Baber, and Badger Citation2016), differences in the impact of land-use and climate changes on ecosystem services have attracted little attention. In this study, Guizhou Province is considered in a case study to quantify the effects of climate and land-use change on sediment and nutrient retention. Our objectives were to: 1) analyze the spatiotemporal evolution of sediment and nutrient retention between 1995 and 2015 using the Integrated Valuation of Environmental Services and Tradeoffs (InVEST) model; and 2) explore the effects of climate and land-use change on sediment and nutrient retention using sensitivity and scenario analyses.
Methodology
Study area
As a typical mountainous area, Guizhou Province (between 24°37′–29°13′ N and 103°36′–109°35′ E) is situated in southwest China where it covers an area of 176,000 km2 (). Altitude of the area is between 154 m and 2,859 m, and the climate type is subtropical monsoon. The temperatures range from 4 ℃ to 25 ℃, and annual precipitation varies between 850 mm and 1600 mm (Han et al. Citation2020). The main land-use types in 1995 and 2015 were farmland, forestland, grassland and shrubland, and the minor land-use types included waterbodies, unused land, and built-up land (). This area is a vulnerable region in China (Xia et al. Citation2019). The population and gross domestic product increased 3.87% and 1.551% from 1995 to 2015, respectively, resulting in great pressure on the natural environment.
Data
Guizhou Province land-use maps in 1995 and 2015 were extracted by Landsat TM/ETM data at a spatial resolution of 30 m. We used ENVI 4.8 software to interpret the images. A digital elevation model with a 30 m resolution was used in this study, which was provided by the ASTER GDEM (Advanced Space-borne Thermal Emission and Reflection Radiometer Global Digital Elevation Model) data (NASA, Houston, TX, USA). The climate data were derived from the China Meteorological Administration (www. cdc.nmic.cn), and the soil map was from the China Soil Scientific Database (www.soil.csdb.cn) (Appendix ). In order to reduce the impact of inter-annual climate fluctuations on the results of the research, the average climate value from 1990 to 1999 is represented as the climate value (including precipitation, temperature, etc.) in 1995, while the average climate value from 2000 to 2019 is represented as the climate value in 2015.
InVEST model
The InVEST model was developed by the Natural Capital Project (www.naturalcapitalproject.org). This model is used to calculate multiple ecosystem services (e.g., habitat quality, water yield, nutrient retention, marine water quality, carbon sedimentation, soil retention, pollination, and crop production) and provide explicit spatial information for land-use management at the watershed or grid scale. The advantages of the model are spatial visualization, wide scope of application, and its ability to reflect the ecological process. Therefore, this model has been widely applied in many countries and regions worldwide (Rukundo et al. Citation2018; Sun et al. Citation2018).
The outputs of the InVEST model are sensitive to input parameters. An error can be present due to multiple model inputs. For example, rainfall erosivity (R) and soil erodibility (K) are necessary to calculate sediment retention. However, there are several calculation methods for the two parameters, and the adaptability of each calculation method differs in different countries and regions. An unsuitable method results in a large error in the assessment result (Sharp et al. Citation2014). The water and soil conservation bulletin and water resources bulletin of Guizhou, which are based on field data, were used to calibrate the model parameters in this study.
The universal soil loss equation (ULSE) (Wischmeier and Smith Citation1978) was used in the InVEST sediment retention model. Sediment retention was calculated using maximum potential soil loss (RKLS), which hypothesizes that the landscape is bare minus the actual soil loss (USLE) (Leh et al. Citation2013). Rainfall erosivity, soil erodibility, the digital elevation model (DEM), land-use type, and a biophysical table (containing C and P as the land-use types) were inputted into the InVEST sediment retention model. The advantage demonstrated by InVEST sediment retention model over others like RUSLE and CLSE lies in the calculation of sediment delivery ratio, which is conducive to producing more reasonable and accurate calculation results (Hu et al. Citation2014).
where is rainfall erosivity,
is soil erodibility,
is the slope length-gradient,
is crop-management,
is support practice.
The InVEST nutrient retention model was used to evaluate the water quality purification services. Total nitrogen and phosphorus were used as representative nutrient pollutants. The vegetation and soil are recognized in the model as key factors to remove or reduce nutrient pollutants through storage and conversion. Precipitation, DEM, the land-use type, the biophysical table (contained by output coefficients and removal efficiency of each land-use type) were inputted into the InVEST nutrient retention model. The advantage of InVEST nutrient retention model is that the effect of vegetation and soil on nutrient delivery is taken into consideration and the spatial pattern of nutrient retention can be put into practice (Sharp et al. Citation2014).
where is the loading value on pixel x,
is the output coefficient on pixel x,
is the hydrological sensitivity on pixel x,
is the runoff coefficient on pixel x,
is the average runoff coefficient in the watershed,
is the sum of the water yield of pixels along the flow path above pixel x.
The sources of the demand data and their calculation methods in the InVEST sediment and nutrient retention modules are shown in Appendix . The sources of the parameters in the two modules were referred from Han and Dong (Citation2017) and Han et al. (Citation2016), Han et al. (Citation2019) (Appendix ).
We used the InVEST sediment retention module as an example to present how the model was validated. The annual soil retention bulletin of Guizhou Province was used to calibrate and validate the module. First, the amount of soil erosion in the 2015 soil retention bulletin of Guizhou Province was used as the reference value. Second, the output value of the module and reference value were compared. Finally, the two calibration parameters (default values of kb and IC0 were 2 and 0.5, respectively) in this module were calibrated until the output value was close to the reference value.
The spatial sediment and nutrient retention maps in 1995 and 2015 were produced by the InVEST model, respectively. The changes in the spatial map between 1995 and 2015 were analyzed with help of the spatial analyst tool in ArcGIS software.
Scenario analysis
Firstly, two scenarios were assumed in this study. Scenario A was defined as climate change with no land-use change from 1995 to 2015. Scenario B was defined as land-use change with no climate change from 1995 to 2015. Secondly, the climate data in 2015 and the land-use data in 1995 were inputted into the InVEST sediment and nutrient retention model. For example, rainfall erosivity calculated using the 2015 precipitation data and the 1995 land-use data was inputted into the sediment retention model, while soil erodibility, DEM, C, and P of the land-use types remained unchanged under scenario A. Sediment and nutrient retention were assessed under scenario A. Similarly, the land-use data in 2015 and the climate data in 1995 were inputted into the InVEST sediment and nutrient retention model. For example, the 2015 land-use data and rainfall erosivity calculated with the 1995 precipitation data were inputted into the sediment retention model, while allowing soil erodibility, DEM, C, and P of the land-use types to remain unchanged under scenario B. Sediment and nutrient retention were assessed under scenario B. Finally, the estimates for these two scenarios were compared to the original values (assessment value in 1995) ().
Sensitivity analysis
The relative importance of different factors in the sediment and nutrient retention models was revealed using the sensitivity analysis method. We used a method by Scardi and Harding (Citation1999) to analyze the sensitivity of the InVEST nutrient and sediment retention models. The minimum and maximum values of each parameter in the InVEST nutrient and sediment retention models were confirmed based on the InVEST User’s Guide. The values were scaled to [0, 1] intervals. The normalized value was adjusted to ±10%, ±8%, ±6%, ±4%, and ±2%. The outputs of InVEST nutrient and sediment retention models were compared between the adjustment and original values. Sensitivity was determined by rainfall erosivity in the InVEST sediment retention model as an example. First, sediment retention output was produced when rainfall erosivity and land-use type in 2015 as well as DEM, soil erodibility, C, and P of the land-use types were inputted into the InVEST sediment retention model. Second, we assumed that other parameters would remain unchanged except rainfall erosivity. Sediment retention output was produced when rainfall erosivity was adjusted to +2%, but was unchanged for the other parameters. Third, the sediment retention outputs between the adjusted and original values for rainfall erosivity were compared. The larger change in output indicated a larger sensitivity. Finally, the sensitivities of the other parameters were determined by a similar method.
Results
3.1 Changes in sediment and nutrient retention when climate and land-use were changed simultaneously.
In this study, we explain the quantity of sediment retained using the amount of sediment exported and the amount of sediment retained. There was an initial sharp decrease in exported sediment and retained sediment in the entire area from 1995 to 2015. A similar decline was found between exported sediment and retained sediment (). Most regions at a watershed scale in the study area showed a downward trend for the amount of sediment exported and sediment retained from 1995 to 2015. A sharp decrease in the region was detected in northern and southwestern parts of Guizhou Province. Only a few watersheds in the central and western parts of the study area showed an increasing trend for the amount of sediment exported. Simultaneously, an increase in the sediment retained was only observed in the western part of the study area ( a(3) and b(3)).
Table 1. Changes in average sediment retention from 1995 to 2015 in Guizhou Province.
Figure 4. Spatial pattern of changes in exported sediment (a) and retained sediment (b) at the watershed scale from 1995 to 2015 in Guizhou Province.
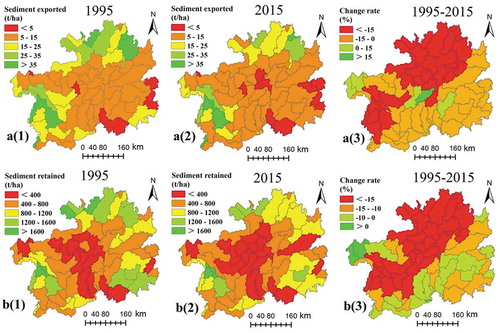
Nutrient retention in this study was explained by nitrogen and phosphorus retention. The average nitrogen and phosphorus retention in the entire area mainly represented a decreasing trend in both parameters from 1995 to 2015. The decline in nitrogen retention was lower than that of phosphorus retention (). Most regions at a watershed scale in Guizhou Province showed a decreasing trend for nitrogen retention from 1995 to 2015, except a few watersheds in the central and southern sectors of the region. A sharp decline in nitrogen retention was observed in the northern and southwestern parts, whereas a sharp decline in phosphorus retention was detected in northern, central, and southwestern parts of the region ( a(3) and b(3)).
Table 2. Changes in average nutrient retention from 1995 to 2015 in Guizhou Province.
Figure 5. Spatial pattern of changes in nitrogen retention (a) and phosphorus retention (b) at a watershed scale from 1995 to 2015 in Guizhou Province.
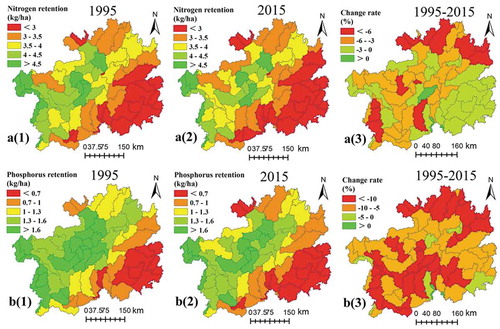
Changes in sediment and nutrient retention under the two different scenarios
The sediment exported and sediment retained decreased by 15.08% and 14.63% from 1995 to 2015 under scenario A, respectively. However, the amount of exported sediment declined by only 0.73%, while the amount of sediment retained increased by 0.06% from 1995 to 2015 under scenario B (). A similar spatial pattern was observed between the change in sediment exported and the change in sediment retained from 1995 to 2015 under scenario A. Namely, the regions with decreasing trends for exported and retained sediment were primarily located in most watersheds, except in the western part of the study area. In addition, the regions in the central and western parts of the study area showed an increasing trend for the amount of sediment exported, while sediment exported in the northern and southern regions of the study area showed a decreasing trend from 1995 to 2015 under scenario B. The amount of retained sediment showed an increasing trend in northern and southern parts of the study area, while it was represented by a decreasing trend in central and western parts of the study area from 1995 to 2015 under scenario B ( a(4), a(5) b(4) and b(5)).
Table 3. Changes in average sediment retention from 1995 to 2015 under different scenarios in Guizhou Province.
Figure 6. Changes in the spatial pattern of exported sediment (a) and retained sediment (b) at a watershed scale from 1995 to 2015 under different scenarios in Guizhou Province.
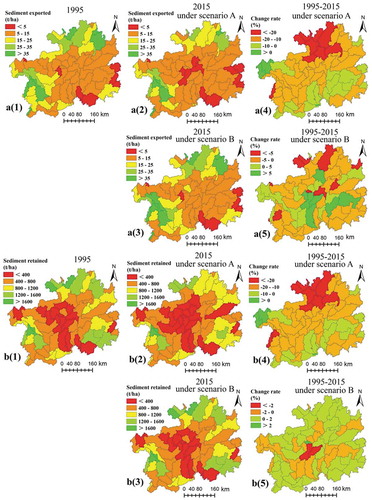
Average nitrogen and phosphorus retention showed a slightly increasing trend from 1995 to 2015 under scenario A, while it represented a relatively large decreasing trend from 1995 to 2015 under scenario B. The variation in average phosphorus retention was higher than average nitrogen retention under scenarios A and B (). Nitrogen and phosphorus retention in most watersheds tended to increase from 1995 to 2015 under scenario A. The regions with a high increasing rate of nitrogen retention were located in the northern part of the study area, while the high increasing rate regions for phosphorus retention were located in the northern and eastern parts of Guizhou Province from 1995 to 2015 under scenario A. In addition, nitrogen and phosphorus retention tended to decrease in most watersheds from 1995 to 2015 under scenario B. The regions with a high decreasing rate of nitrogen and phosphorus retention were located in northern and central-western parts of the study area under scenario B ( a(4), a(5) b(4) and b(5)).
Table 4. Changes in average nutrient retention from 1995 to 2015 under different scenarios in Guizhou Province.
Sensitivity analysis
The sensitivity of sediment retention in response to rainfall erosivity and soil erodibility exhibited a significant influence, whereas elevation, the cover-management factor, and the support practice factor had little effect on sediment retention. For example, the amount of sediment retention increased by 4.00% and 2.04%, respectively, when the rainfall erosivity and the soil erodibility were adjusted from 0% to 10%, whereas the amount of sediment retention increased by 0.02%, 0.09%, and 0.11%, respectively, when the elevation, the cover-management factor, and the support practice factor were adjusted from 0% to 10% (). Retention efficiency exhibited a dominant effect on nutrient retention. The effects of rainfall, elevation, and the calibration parameter on nutrient retention were relatively small. For example, the amount of nutrient retention decreased by 0.41% when the retention efficiency was adjusted from 0% to 10%, whereas the amount of nutrient retention increased by 0.01%, 0.02%, and 0.04%, respectively, when the elevation, the calibration parameter, and the rainfall were adjusted from 0% to 10% ().
Discussion
Effects of climate and land-use change on sediment and nutrient retention
The results revealed that climate change had a more important role in sediment retention change than land-use change, which echoed studies from other countries and regions (Bangash et al. Citation2013; Notebaert et al. Citation2011). Nevertheless, the role played by climate change in sediment retention in this study shows difference to the findings of some scholars (Hoyer and Chang Citation2014; Jiang et al. Citation2017), which was largely attributed to the difference in spatial scale (Bai, Ochuodho, and Yang Citation2019). Furthermore, the results indicated that the effect of land-use change on nutrient retention was more significant compared to climate change, as shown in many similar studies to those carried out by Lorencová et al. (Citation2016) and Sannigrahi et al. (Citation2020). It is worth noting that there were evident difference in the effects of various land-use categories on sediment and nutrient retention. The decline of nutrient retention from 1995 to 2015 under scenario B was closely related to the increased area of dry land with high output coefficients of Nitrogen and Phosphorus as well as relatively large quantitative change. The main reason for increased sediment retention from 1995 to 2015 under scenario B was the substantial increase of built-up land and forestland ( and ). Those differences were demonstrated by Hoyer and Chang (Citation2014) and Sannigrahi et al. (Citation2020).
Table 5. Changes of land-use and climate from 1995 to 2015 in Guizhou Province.
Specific information is provided by a scenario analysis, which is helpful to analyze the results that are sensitive to climate and land-use change (Runting et al. Citation2016; Martinez-Harms et al. Citation2017). For example, when we assumed that land-use changed from 1995 to 2015 and climate remained unchanged, the rate of change in average nutrient retention was relatively high. However, when the climate was changed from 1995 to 2015 while land-use remained unchanged, the rate of change in average nutrient retention was relatively small. Those results suggest that the effect of climate change on nutrient retention was much less than that of land-use change.
Due to no changes to DEM and soil erodibility within a short period of time, climate and land-use changes have an impact on sediment retention by affecting rainfall erosivity, the cover-management factor, and the support practice factor. As a major influencing factor for the change in sediment retention, as shown in , the decline of rainfall erosivity resulted in the reduction to sediment retention from 1995 to 2015. Despite the worsening soil erosion caused by the reduction of shrubland and grassland, the increase of forestland and built-up land is conducive to reducing soil erosion and improving the capacity of sediment retention, as indicated by . Thus, a decline of sediment retention could be observed between 1995 and 2015 under the context of declining rainfall erosivity and increasing forestland and built-up land. Similarly, compared to other factors, nutrient retention was significantly affected by the retention efficiency of each land-use category, as shown in . The reduction to shrubland and grassland caused the purifying capacity to decline, as shown in . Moreover, the decrease of rainfall reduced the delivery of nutrients in the water, as a result of which a downward trend of nutrient retention can be observed from 1995 to 2015.
Implications for land management
Environmental management considers the impact of a land-use change on sediment and nutrient retention in a watershed scale according to the InVEST model results (Sharp et al. Citation2014). The results of this study indicate that sediment and nutrient retention decreased in some watersheds. Therefore, the amount of natural vegetation should increase in these watersheds. On the one hand, Green for Grain Project needs to be stepped up for the local government. The increase of vegetation coverage and the declining proportion of slope farmland should be continued in the future. On the other hand, the expansion of rocky-desertification shall be disencouraged and the amount of unused land shall be reduced. The amount of built-up land experienced a sharp increase from 1995 to 2015 (increased by 187.57%) due to rapid economic development. This factor will certainly impact on natural vegetation and farmland in the future, resulting in negative effects on sediment and nutrient retention. Accordingly, we must control the increase in built-up land. In order to balance economic development and ecological protection for the study area, ecological conservation redline and the boundary of the expansion of built-up land should first be delimited, and strategies such as the economical and intensive use of built-up land as well as residential land exit in hollow villages should be pushed forward. In addition, the farmland and ecologically sustainable land occupied for built-up land ought to be reduced and production-living-ecological space should be optimized.
Limitations of the InVEST model and prospects of future study
The sedimentation process was simplified by the InVEST sediment retention model; however, this model does not consider all sedimentary processes (Leh et al. Citation2013; Su and Fu Citation2013). For instance, this model does not consider rill, gullies, and stream-bank erosion, or deposition processes (Sharp et al. Citation2014). Furthermore, it was difficult to obtain the filtration velocity of the land-use types in the study area. Therefore, we referred to the values in other regions, which negatively impacted accuracy of the assessment. The InVEST nutrient retention model does not consider chemical or biological interactions with vegetation. In addition, tile drainage and ditches could cut the path of nutrient movement and result in inaccurate estimates (Sharp et al. Citation2014). Besides, the impacts of crop types and the amount of fertilizer usage were not considered in the InVEST model. Land-use and climate changes exerted influence on ecosystem services in a different way depending on the exact spatial scale. Therefore, it is a significant direction of further study to compare the differences in the impact of land-use and climate changes on ecosystem services, for determining the underlying mechanism behind this. Furthermore, land-use optimization provides an important solution to mitigating the negative impact of climate change on ecosystem services. Such land optimization policies as the projects of Green for Grain and rocky-desertification treatment have been extensively launched across the Southwest of China. The role that land management policy plays in the mitigation of negative impact on ecosystem services due to climate change shall be reinforced in the future.
Conclusion
Average sediment and nutrient retention declined from 1995 to 2015 when climate and land-use change occurred simultaneously. Sediment exported and sediment retained tended to decline under scenario A. However, the amount of sediment exported tended to decrease, whereas sediment retained tended to increase under scenario B. Average nutrient retention tended to increase under scenario A and decrease under scenario B.
The effect of climate change on sediment retention was higher than that of land-use change, while land-use change played a more important role in nutrient retention than climate change. As revealed by the results of sensitivity analysis for the sediment retention model, rainfall erosivity and soil erodibility are more sensitive than other factors. Retention efficiency played a dominant role in the nutrient retention model. In the future, it is essential to enhance the mechanism by which land-use and climate changes impact on ecosystem services at different spatial scales and the role of land management policy in mitigating the negative impact of climate change on ecosystem services.
Acknowledgments
This work was supported by the Science and Technology Foundation of Guizhou Province (NO.[2020]1Y152), the Humanity and Social Science Youth foundation of the Ministry of Education (18YJCZH042) and the Scientific Research Foundation of the Guizhou Institute of Technology.
Additional information
Funding
References
- Bai, Y., T. O. Ochuodho, and J. Yang. 2019. “Impact of Land Use and Climate Change on Water-related Ecosystem Services in Kentucky, USA.” Ecological Indicators 102: 51–13. doi:10.1016/j.ecolind.2019.01.079.
- Bangash, R. F., A. Passuello, M. Sanchez-Canales, M. Terrado, A. López, F. J. Elorza, G. Ziv, V. Acuña, and M. Schuhmacher. 2013. “Ecosystem Services in Mediterranean River Basin: Climate Change Impact on Water Provisioning and Erosion Control.” Science of the Total Environment 458-460: 246–255. doi:10.1016/j.scitotenv.2013.04.025.
- Chen, H., D. Zhu, H. Chen, Y. Wen, D. Lin, and C. Wang. 2018. “Comparison of Climate Change Characteristics of Different Watersheds in Yangtze River Basin of Guizhou Province.” Bulletin of Soil and Water Conservation 38 (2): 189–197.
- Cheng, J., X. Lee, Y. Tang, W. Pan, W. Gao, Y. Chen, B. Wang, and H. Cheng. 2016. “Changes in Above- and Below-ground Nitrogen Stocks and Allocations following the Conversion of Farmland to Forest in Rocky Desertification Regions.” Agriculture, Ecosystems & Environment 232: 9–16. doi:10.1016/j.agee.2016.07.019.
- Costanza, R., R. d’Arge, R. de Groot, S. Farber, M. Grasso, B. Hannon, K. Limburg, et al.. 1997. “The Value of the World’s Ecosystem Services and Natural Capital”. Nature 387: 253–260. doi:10.1038/387253a0.
- Fan, M., H. Shibata, and L. Chen. 2018. “Assessing High Impacts of Climate Change: Spatial Characteristics and Relationships of Hydrological Ecosystem Services in Northern Japan (Teshio River Watershed).” Mitigation and Adaptation Strategies for Global Change 23: 525–552. doi:10.1007/s11027-017-9748-x.
- Fan, M., H. Shibata, and Q. Wang. 2016. “Optimal Conservation Planning of Multiple Hydrological Ecosystem Services under Land Use and Climate Changes in Teshio River Watershed, Northernmost of Japan.” Ecological Indicators 62: 1–13. doi:10.1016/j.ecolind.2015.10.064.
- Fu, B., Y. Liu, Y. Lü, C. He, Y. Zeng, and B. Wu. 2011. “Assessing the Soil Erosion Control Service of Ecosystems Change in the Loess Plateau of China.” Ecological Complexity 8: 284–293. doi:10.1016/j.ecocom.2011.07.003.
- Griffiths, L. N., and W. J. Mitsch. 2020. “Nutrient Retention via Sedimentation in A Created Urban Stormwater Treatment Wetland.” Science of the Total Environment 727: 138337. doi:10.1016/j.scitotenv.2020.138337.
- Han, H., G. Yang, and F. Zhang. 2015. “Spatio-temporal Analysis of Land Use Changes in Guizhou Province Based on Landform Characteristics.” Journal of Nanjing Forestry University (Natural Sciences Edition) 39: 99–105.
- Han, H., H. Gao, Y. Huang, X. Chen, M. Chen, and J. Li. 2019. “Effects of Drought on Freshwater Ecosystem Services in Poverty-stricken Mountain Areas.” Global Ecology and Conservation 17: e00537. doi:10.1016/j.gecco.2019.e00537.
- Han, H., X. Luo, R. You, X. Luo, and Y. Chen. 2016. “Analysis of Water Purification Function in the Pearl River Basin in Guizhou Province Using InVEST Model.” Journal of Nanjing Forestry University (Natural Sciences Edition) 40: 87–92.
- Han, H., and Y. Dong. 2017. “Assessing and Mapping of Multiple Ecosystem Services in Guizhou Province, China.” Tropical Ecology 58: 331–346.
- Han, H., Y. Liu, H. Gao, Y. Zhang, Z. Wang, and X. Chen. 2020. “Tradeoffs and Synergies between Ecosystem Services: A Comparison of the Karst and Non-karst Area.” Journal of Mountain Science 17: 1221–1234. doi:10.1007/s11629-019-5667-5.
- Hopkins, K. G., G. B. Noe, F. Franco, E. J. Pindilli, S. Gordon, M. J. Metes, P. R. Claggett, A. C. Gellis, C. R. Hupp, and D. M. Hogan. 2018. “A Method to Quantify and Value Floodplain Sediment and Nutrient Retention Ecosystem Services.” Journal of Environmental Management 220: 65–76. doi:10.1016/j.jenvman.2018.05.013.
- Hoyer, R., and H. Chang. 2014. “Assessment of Freshwater Ecosystem Services in the Tualatin and Yamhill Basins under Climate Change and Urbanization.” Applied Geography 53: 402–416. doi:10.1016/j.apgeog.2014.06.023.
- Hu, S., M. Cao, Q. Liu, T. Zhang, H. Qiu, W. Liu, and J. Song. 2014. “Comparative Study on the Soil Conservation Function of InVEST Model under Different Perspectives.” Geographical Research 33 (12): 2393–2406.
- Huang, W., H. C. Ho, Y. Peng, and L. Li. 2016. “Qualitative Risk Assessment of Soil Erosion for Karst Landforms in Chahe Town, Southwest China: A Hazard Index Approach.” Catena 144: 184–193. doi:10.1016/j.catena.2016.05.008.
- Jiang, C., H. Zhang, Z. Tang, and L. Labzovskii. 2017. “Evaluating the Coupling Effects of Climate Variability and Vegetation Restoration on Ecosystems of the Loess Plateau, China.” Land Use Policy 69: 134–148. doi:10.1016/j.landusepol.2017.08.019.
- Leh, M. D. K., M. D. Matlock, E. C. Cummings, and L. L. Nalley. 2013. “Quantifying and Mapping Multiple Ecosystem Services Change in West Africa.” Agriculture, Ecosystems & Environment 165: 6–18. doi:10.1016/j.agee.2012.12.001.
- Lenka, N. K., K. K. Satapathy, R. Lal, R. K. Singh, P. K. Agrawal, P. Choudhury, and A. Rathore. 2017. “Weed Strip Management for Minimizing Soil Erosion and Enhancing Productivity in the Sloping Lands of North-eastern India.” Soil & Tillage Research 170: 104–113. doi:10.1016/j.still.2017.03.012.
- Lorencová, K. E., Z. V. Harmáčková, L. Landová, A. Pártl, and D. Vačkář. 2016. “Assessing Impact of Land Use and Climate Change on Regulating Ecosystem Services in the Czech Republic.” Ecosystem Health and Sustainability 2 (3): e01210. doi:10.1002/ehs2.1210.
- Martinez-Harms, M. J., B. A. Bryan, E. Figueroa, P. Pliscoff, R. K. Runting, and K. A. Wilson. 2017. “Scenarios for Land Use and Ecosystem Services under Global Change.” Ecosystem Services 25: 56–68. doi:10.1016/j.ecoser.2017.03.021.
- Millennium Ecosystem Assessment (MA). 2005. Ecosystems and Human Well-being: The Assessment Series (Four Volumes and Summary). Washington, DC: Island Press.
- Notebaert, B., G. Verstraeten, P. Ward, H. Renssen, and A. V. Rompaey. 2011. “Modeling the Sensitivity of Sediment and Water Runoff Dynamics to Holocene Climate and Land Use Changes at the Catchment Scale.” Geomorphology 126: 18–31. doi:10.1016/j.geomorph.2010.08.016.
- Rukundo, E., S. Liu, Y. Dong, E. Rutebuka, E. F. Asamoah, J. Xu, and X. Wu. 2018. “Spatio-temporal Dynamics of Critical Ecosystem Services in Response to Agricultural Expansion in Rwanda, East Africa.” Ecological Indicators 89: 696–705. doi:10.1016/j.ecolind.2018.02.032.
- Runting, R. K., B. A. Bryan, L. E. Dee, F. J. F. Maseyk, L. Mandle, P. Hamel, K. A. Wilson, K. Yetka, H. P. Possingham, and J. R. Rhodes. 2016. “Incorporating Climate Change into Ecosystem Service Assessments and Decisions: A Review.” Global Change Biology 23: 28–41. doi:10.1111/gcb.13457.
- Sample, J. E., I. Baber, and R. Badger. 2016. “A Spatially Distributed Risk Screening Tool to Assess Climate and Land Use Change Impacts on Water-related Ecosystem Services.” Environmental Modelling and Software 83: 12–26. doi:10.1016/j.envsoft.2016.05.011.
- Sannigrahi, S., Q. Zhang, P. K. Joshi, P. C. Sutton, S. Keesstra, P. S. Roy, F. Pilla, et al.. 2020. “Examining Effects of Climate Change and Land Use Dynamic on Biophysical and Economic Values of Ecosystem Services of A Natural Reserve Region.” Journal of Cleaner Production 257: 120424. doi:10.1016/j.jclepro.2020.120424.
- Scardi, M., and L. Harding. 1999. “Developing an Empirical Model of Phytoplankton Primary Production: A Neural Network Case Study.” Ecological Modelling 120: 213–223. doi:10.1016/S0304-3800(99)00103-9.
- Schirpke, U., M. Kohler, G. Leitinger, V. Fontana, E. Tasser, and U. Tappeiner. 2017. “Future Impacts of Changing Land-use and Climate on Ecosystem Services of Mountain Grassland and Their Resilience.” Ecosystem Services 26: 79–94. doi:10.1016/j.ecoser.2017.06.008.
- Sharp, R., H. T. Tallis, T. Ricketts, A. D. Guerry, S. A. Wood, R. Chaplin-Kramer, E. Nelson, et al.. 2014. “InVEST User’s Guide.” The Natural Capital Project. Stanford.
- Shrestha, M. K., F. Recknagel, J. Frizenschaf, and W. Meyer. 2017. “Future Climate and Land Uses Effects on Flow and Nutrient Loads of A Mediterranean Catchment in South Australia.” Science of the Total Environment 590: 186–193. doi:10.1016/j.scitotenv.2017.02.197.
- Skaalsveen, K., J. Ingram, and L. E. Clarke. 2019. “The Effect of No-till Farming on the Soil Functions of Water Purification and Retention in North-western Europe: A Literature Review.” Soil & Tillage Research 189: 98–109. doi:10.1016/j.still.2019.01.004.
- Su, C., and B. Fu. 2013. “Evolution of Ecosystem Services in the Chinese Loess Plateau under Climatic and Land Use Changes.” Global and Planetary Change 101: 119–128. doi:10.1016/j.gloplacha.2012.12.014.
- Sun, X., J. C. Crittenden, F. Li, Z. Lu, and X. Dou. 2018. “Urban Expansion Simulation and the Spatio-temporal Changes of Ecosystem Services, A Case Study in Atlanta Metropolitan Area, USA.” Science of the Total Environment 622: 974–987. doi:10.1016/j.scitotenv.2017.12.062.
- Tan, P. Y., J. Zhang, M. Masoudi, J. B. Alemu, P. J. Edwards, A. Grêt-Regamey, D. R. Richards, J. Saunders, X. Song, and L. W. Wong. 2020. “A Conceptual Framework to Untangle the Concept of Urban Ecosystem Services.” Landscape and Urban Planning 2020: 103837. doi:10.1016/j.landurbplan.2020.103837.
- Uwimana, A., A. A. van Dam, G. M. Gettel, and K. Irvine. 2018. “Effects of Agricultural Land Use on Sediment and Nutrient Retention in Valley-bottom Wetlands of Migina Catchment, Southern Rwanda.” Journal of Environmental Management 219: 103–114. doi:10.1016/j.jenvman.2018.04.094.
- Williams, J. R., K. G. Renard, and P. T. Dyke. 1983. “EPIC: A New Method for Assessing Erosion’s Effect on Soil Productivity.” Journal of Soil and Water Conservation 38: 381–383.
- Wischmeier, W. H., and D. D. Smith. 1978. “Predicting Rainfall Erosion Losses – A Guide to Conservation Planning”. In Agriculture Handbook No. 537 USDA/Science and Education Administration. Washington, DC: US Govt. Printing Office.
- Xia, S., Y. Zhao, Q. Wen, X. Xu, P. Cui, and W. Tang. 2019. “Spatiotemporal Dynamics and Influencing Factors of Poverty in Ecologically Fragile Areas of A Karst Region: A Case Study of Guizhou Province.” Acta Ecologica Sinica 39 (18): 6869–6879.
- Zhang, W., and J. Fu. 2003. “Rain Erositivity Estimation under Different Rainfall Amount.” Recourses Science 25: 35–41.
- Zheng, J., H. Han, G. Cai, and H. Gao. 2013. “Spatial-Temporal Characteristic of Agricultural Non-point Source Nitrogen and Phosphorous Pollution in Guizhou.” Journal of Yangtze River Scientific Research Institute 30: 1–4. doi:10.3969 /j.issn.1001-5485.2013.06.001
Appendix A
Table A1. Data sources for InVEST sediment retention and nutrient retention model.
Table A2. Parameters of InVEST sediment retention and nutrient retention model.