Abstract
The Silk Road Economic Belt and the 21st-Century Maritime Silk Road (B&R) aims at facilitating the twenty-first Century economic development of China. However, climate change, air quality and related feedbacks are affecting the successful development of the environment and societies in the B&R geographical domain. The most urgent risks related to the atmospheric system, to the land system and to hydrospheric and cryospheric processes are changing climate – air quality interactions, air pollution, changing monsoon dynamics, land degradation, and the melting of Tibetan Plateau glaciers. A framework is needed in which a science and technology-based approach has the critical mass and expertise to identify the main steps toward solutions and is capable to implement this roadmap. The Pan-Eurasian Experiment (PEEX) program, initiated in 2012, aims to resolve science, technology and sustainability questions in the Northern Eurasian region. PEEX is now identifying its science agenda for the B&R region. One fundamental element of the PEEX research agenda is the availability of comprehensive ground-based observations together with Earth observation data. PEEX complements the recently launched international scientific program called Digital Belt and Road (DBAR). PEEX has expertise to coordinate the ground-based observations and initiate new flagship stations, while DBAR provides a big data platform on Earth observation from China and countries along the Belt and Road region. The DBAR and PEEX have joint interests and synergy expertise on monitoring on ecological environment, urbanization, cultural heritages, coastal zones, and arctic cold regions supporting the sustainable development of the Belt and Road region. In this paper we identify the research themes of the PEEX related Silk Road agenda relevant to China and give an overview of the methodological requirements and present the infrastructure requirements needed to carry out large scale research program.
1. Background
In 2013, President XI Jinping initiated the Silk Road Economic Belt and the twenty-first Century Maritime Silk Road (B&R) aimed at facilitating the twenty-first Century economic development of China (l). The B&R is estimated to be one of the world largest economic transport corridors of the twentieth century (Figure ). Without new scientific understanding of the land, atmosphere, society interactions and feedbacks along the Belt and Road region, it is not possible to carry out the successful implementation of the B&R. The holistic system understanding is the baseline for the sustainable development of the B&R societal, economic and transport corridor activities. The main challenges are (1) to understand the consequence of the global climate change and vice versa at regional scales across the B&R region, and (2) to estimate the local and global environmental effects of increased human activities and new infrastructure of the B&R transport corridors.
Figure 1. The “Silk Road Economic Belt” the Asia-Pacific economic circle in the east and the European economic circle in the west, and the “the 21st-Century Maritime Silk Road” links the major port cities in China and Southeast Asian countries with Europe. It is the longest economic corridor in the world with the high potential. The Belt and Road is composed over 65 countries with a population of 4.4 billion, which is 63% of the world’s population (left), figure adopted from (www.chinadaily.com.cn/bizchina/2014-06/28/content_17621525.htm) PEEX domain (right), figure adopted from the PEEX Science Plan (Lappalainen et al., Citation2014).
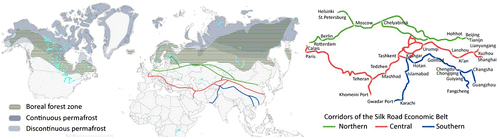
A multidisciplinary scientific approach designed to tackle large-scale environmental challenges, called in the Pan-Eurasian Experiment (PEEX) program, was initiated in 2012 (Kulmala, Petäjä et al. Citation2016; Lappalainen et al., Citation2014, Citation2016). The PEEX program is built on four pillars: (1) research agenda, (2) research infrastructures (development of comprehensive, coordinated observation systems), and (3) society impact (e.g. adaptation and mitigation, recommendations for the climate policy-making, recommendations to air quality issues) and (4) knowledge transfer (education). For the time being, the geographical focus of this program has been on the Northern Eurasian arctic – boreal region, the region where the warming has been twice as fast as in other regions of the Earth system (IPCC, Citation2013). China has been identified as part of the PEEX geographical region, its significance mainly as a hotspot region for climate, air quality, and long-term transport point of view (Kulmala et al., Citation2015; Lappalainen et al., Citation2016). The program released a science plan in 2015, which described the most important research topics and large-scale research questions relevant to the Northern Eurasian region (Kulmala et al., Citation2015; Lappalainen et al., Citation2014, Citation2016).
The motivation for launching the PEEX Arctic-boreal research agenda was based on the foreseen large-scale environmental and socio-economic changes driven by the Arctic warming and opening of the Arctic Ocean Northern sea route as new transport corridor (IPCC, Citation2013; Smith, Citation2010). Now the B&R program sets the focus on the Asian region. The Belt and Road is composed over 65 countries with a population of 4.4 billion, which is 63% of the world’s population. The Silk Road regions will be linked to trends of globalization, climate change, demography and use of natural resources. Land and water areas located along with the Silk Road corridors will undergo substantial changes during the upcoming decades. The economic transport corridors may not only enhance the urbanization of the Silk Road countries and the emergence of new megacities, but also increase the pollution loads to the atmosphere and ecosystems. PEEX is now recognizing the momentum to identify it’s China-specific agenda and to find the synergies with the recently released new China lead initiatives. PEEX complements the recently launched international scientific program called Digital Belt and Road (DBAR). PEEX has expertise to coordinate the ground-based observation and initiate new flagship stations, while DBAR provides a big data platform on earth observation from China and countries along the Belt and Road area. The DBAR and PEEX have joint interests and synergy expertise on monitoring of the ecological environment, urbanization, cultural heritages, and arctic cold regions and coastal zones, and supporting the sustainable development of the Belt and Road region.
The aim of this paper is to introduce the themes of the PEEX B&R program relevant to China. At present, atmospheric air pollution in China threatens the health of hundreds of millions of people (e.g. Kulmala, Citation2015; Lelieveld, Evans, Fnais, Giannadaki, & Pozzer, Citation2015; Maji, Dikshit, Arora, & Deshpande, Citation2018). In addition, it causes major problems to the environment and economy as a whole by decreasing, e.g. the agricultural and industrial productivity of the nation as a whole. China’s air pollution situation has worsened dramatically during the last 2−3 decades as emissions from industry, energy production and traffic have increased (e.g. de Leeuw et al., Citationin press; Wang et al., Citation2017), although emission regulations have been taking effects in recent years as shown by satellite observations of atmospheric trace gases (van der et al., Citation2017) and aerosols (de Leeuw et al., Citationin press). In the wider context, these regional air pollution processes relate significantly to the global-scale air quality–climate interactions, atmospheric circulation dynamics and extreme weather events. The atmospheric concentrations of primary and secondary pollutants in China are 10 to 100 times (sometimes even 1000 times) higher than currently in Europe or North America (Kulmala, Citation2015). The complex feedbacks related to air quality may influence and generate elements that have remediation in the Earth System level. In the Asia region these elements are “Indian monsoon transformation” and “Tibetan albedo change” (Schellnhuber, Cruzen, Clark, Claussen, & Held, Citation2004). In this paper, we give an overview of the methodological requirements to solve large-scale research questions and present the research infrastructure requirements needed to carry out a large-scale research approach. We introduce a collaboration framework and collaborators finding synergy in complementing the expertise for solving the environmental challenge of the B&R.
2. Research themes and examples of previous results
The PEEX B&R research agenda is underlining the research topics relevant to the sustainable development across the Northern corridor and in China (Figure ).
Figure 2. PEEX is interested in and contributing to understanding and quantification of the interlinks and feedbacks of the land-atmosphere-aquatic and society systems relevant to solving grand challenges such as climate change, air quality, food security, and water supply.
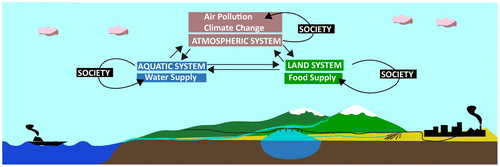
PEEX works toward understanding the interlinks and feedbacks of the land-atmosphere-aquatic system starting from climate–air quality interactions and expanding the understanding toward society-relevant sectors, such as food security and water. The leading edge of the PEEX-China agenda is the atmospheric system, which includes air quality and the feedbacks between air quality and weather and climate. The environments of the Silk Road Northern Corridor (Figure ) will be a very important source / sink area for transported air pollutants, also reflecting to the global climate dynamics. The air quality – weather – climate interactions are also involving a wide range of dynamical processes tied with both land and aquatic systems and with the society.
The research agenda includes the main features affecting the atmospheric system. Here we address the interaction between the atmospheric system and changing land ecosystems (land uses changes, ecosystems degradation), societal systems (industrialization, urbanization, migration, traffic etc.), and aquatic systems (sea surface dynamics and air-sea interaction). The main themes of the land system are (1) the land use change, which connects human actions to large-scale physical, chemical and biological processes, (2) monsoon dynamics, and (3) land degradation. The main theme of the hydrosphere/cryosphere system pays attention to the high-mountain cold regions, especially the Tibetan Plateau, where the temperatures are rising four times faster than anywhere else in Asia, and where glacier retreat and permafrost melting processes are affecting water supplies and hydrological cycles throughout Asia (Kraaijenbrink, Bierkens, Lutz, & Immerzeel, Citation2017).
2.1. Atmospheric system
2.1.1. Air-quality – climate interactions
A holistic scientific understanding of the atmospheric phenomena associated with air quality as a whole, as well as of the connection between air quality and climate, is currently lacking (Fiore et al., Citation2012; Fuzzi et al., Citation2015; Kulmala, Citation2015). Together with emission reductions, the key way to get forward is to make long-term, continuous and comprehensive observations of aerosol particles (mass, number, chemical composition, optical properties), trace gases (SO2, NOx, CO, VOCs, sulphuric acid, HONO, HNO3, NH3, etc.), and atmospheric oxidants (O3, HOx, ROx, NO3, Criegee intermediates etc.) as well as greenhouse gases (Kulmala et al., Citation2015). With a network of such observation stations (Hari et al., Citation2016, Kulmala, Citation2018), we will be able to understand the interactions and feedbacks associated with the urban pollution mixture and, ultimately, be ready to make targeted strategies for pollution control (Figure ). Recent advances in our theoretical understanding has made it possible to explain how enhanced pollution decreases atmospheric turbulence and mixing, reducing the boundary layer height and causing further enhancement of pollution levels (Petäjä et al., Citation2016).
Figure 3. The schematic diagram of the atmospheric chemistry – society – policy-making – infrastructure loop.
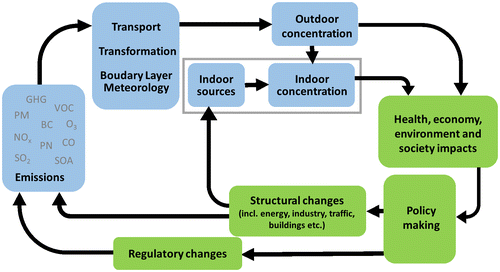
Megacities and industrial areas in China and Russia contribute significantly to the pollution load of the global atmosphere. These pollutants have the potential to impact atmospheric circulation and weather close to their sources areas (Ding et al., Citation2013a; Guo et al., Citation2017). Further away, and especially in boreal and Arctic regions, pollutants originating from China and Russia may significantly affect the chemical composition of the atmosphere (Monks et al., Citation2015), thereby affecting, e.g. the biosphere by altering VOC emissions, their atmospheric chemistry and resulting secondary aerosol formation. As described above, the air quality in polluted areas and climate change are tightly interlinked and therefore they cannot be solved separately.
Through the production of new secondary aerosol particles, the atmospheric gas-to-particle conversion (GTP) process affect local, regional and global aerosol loadings and alters aerosol size distributions and Cloud Condensation Nuclei (CCN) concentrations. In polluted conditions the main concern is air quality, whereas in pristine conditions the interest lies more in the changing climate. GTP – particularly in the sub-5 nm size range (nano GTP) – is a key element in understanding atmospheric chemistry and nanophysics. By quantifying the processes, interactions and feedbacks, we will be able to determine the most efficient steps towards significantly reducing secondary air pollution (in some cases by a factor of 5−10), to estimate the potential that the biosphere in Northern Hemisphere has in reducing global radiative forcing via the quantified feedbacks, and to estimate anthropogenic and biogenic contributions to global and regional aerosol loadings, particularly related to pollution in the arctic/boreal regions in a changing climate (Figure ).
Figure 4. Schematic picture of the interactions and feedbacks between the anthropogenic and biogenic components in the Arctic-boreal area affected by the megacities and industrial areas in China/Russia and consequences for air quality within China/Russia and for the global climate – air quality interactions.
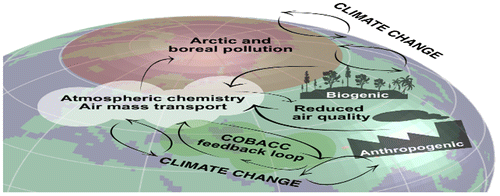
2.1.2. Urban air quality
“Air Quality” is addressing three sub-themes: “aerosol formation processes in urban environments,” “ozone pollution”, and “urban heat/cool islands” affected by the boundary layer dynamics. There are several major physical and chemical processes that are not yet well understood. Understanding of these dynamical processes is needed for developing effective emission policies and for the construction of reliable early warning systems and pollution-episode alerts, together with most effective technical solutions for clean environment.
2.1.3. Aerosols, gas-to-particle conversion and low volatile organic compounds (LVOCs)
The identification of unpredicted chemical reactions between pollutants is the first step in finding solutions to improve air quality in megacities (Kulmala, Citation2015). Highly non-linear processes, such as atmospheric chemistry and aerosol dynamics, transform the urban pollution cocktail and generate secondary pollution, such as ultrafine particles and ozone, during their residence in the atmosphere (Guo et al., Citation2014, Huang et al., Citation2014). The fact that new particle formation does occur in polluted Chinese megacities like Beijing and Shanghai (Xiao et al., Citation2015), or even during dust-storms (Nie et al., Citation2014), suggests that there are several major physical and chemical mechanisms in a heavily-polluted atmosphere that have not been recognized before and may not even be operating in clean or moderately polluted environments (Kulmala, Kerminen, Petäjä, Ding, & Wang, Citation2017).
Kulmala et al. (Citation2014) have recently revised the traditional view of the atmospheric nano-GTP process. The revised view includes two new steps to make a five-step process: (1) Formation of low volatile compounds via different oxidation pathways, (2) Clustering, (3) Nucleation or barrierless nucleation, (4) Activation of clusters with a second group of vapors, and (5) multicomponent condensation.
We need to explore how different organic compounds participate in the various steps of nano-GTP under different conditions, how they are formed in the gas phase, and by which mechanisms they participate in new-particle formation. We need to quantify all five steps related to nano-GTP and compare their mutual importance in different conditions and environments. More specifically, we will find out the relative importance of different processes and the boundary conditions controlling why nano-GTP sometimes produces new particles (>3 nm in size) but in most other cases this does not happen. The most important issue is to find out which compounds are needed for driving effective aerosol formation via nano-GTP. In the present day atmosphere, it seems that the simultaneous presence of sulfuric acid, base molecules, and organic vapors is required (see, e.g. Kulmala et al., Citation2013). More detailed fundamental process level understanding is required also to find out the effect of the ambient temperature, humidity and UV radiation, and the role of ions in, the atmospheric nano-GTP and NPF. A specific focus should be on the importance of different (extremely) low volatile organic compounds, i.e. (E)LVOCs, for nano-GTP.
The chemistry driving the formation of ELVOCs was unknown to atmospheric chemists until a few years ago when new research instruments were development and applied in both laboratory and field conditions (Ehn et al., Citation2014; Jokinen et al., Citation2012; Rissanen et al., Citation2014; Sipila et al., Citation2010). Improving our understanding of peroxy radical (RO2) chemistry, especially their ability to auto-oxidize (Crounse et al., Citation2013), is a critical part in the improved understanding. As an example, monoterpenes (C10H16) are known to produce (E)LVOCs with high yields, including highly oxidized C10 monomers, organic nitrates such as the species at m/z 339 (C10H15NO8) that has been seen to be crucial in clustering and initial steps of the growth in day-time NPF events in Hyytiälä, Finland (e.g. Kulmala et al., Citation2013), as well as C20 dimers, which appear to drive pure organic new-particle formation (Kirkby et al., Citation2016). The exact chemical environment will determine which of these pathways becomes dominant, and is expected to be critical for GTP dynamics.
Ozone (O3) is one of the most important secondary pollutants and greenhouse gases. An increasing trend of surface or tropospheric O3 has been reported in development regions in eastern China (Ding et al., Citation2008; Wang et al., Citation2009), together with a fast increase of O3 precursors like NOx. However, there is limited knowledge of temporal variations and their courses in the downwind region of fast-developed urban city clusters, such as the Yangtze River Delta (YRD). The first analysis of ozone in the western YRD region of China shows a positive trend of ozone (about 2.1 ppbv per year) and an increased frequency of ozone episodes in Nanjing during 2011–2015. A springtime ozone maximum was observed in the free troposphere and a summer maximum in boundary layer. A high concentration of O3 was observed in the upper boundary layer, which was mainly due to sub-regional transport from the city cluster in the YRD region and also influenced by the regional transport of biogenic emission from South China (Ding et al., Citation2013b, Petzold et al., Citation2015).
Urban environments are mainly characterized by cities in China with heavy anthropogenic emissions from local industry, traffic and housing, and by the megacity regions like Shanghai and Beijing with alarming air quality levels. In addition to serious health effects, the atmospheric pollutants and oxidants (SO2, O3, NOX, BC, sulfate, secondary organic aerosols) play a central role in climate change dynamics via their direct and indirect effects on global albedo and radiative transfer. Satellite observations show that NOx emissions have been increasing in SE China until 2010–2014 (depending on the province). In these years NOx emission reduction equipment was installed in power plants and strict air quality regulation was enforced, resulting in the reduction of NOx (van der et al., Citation2017). Low-volatile VOCs cannot be directly observed from satellites, but formaldehyde and isoprene are used as proxies. Satellite observations clearly show the seasonal variations over the North China Plain with peaks during the straw burning seasons in the summer before the arrival of the SEAS (South East Asia Summer Monsoon. These observations show a clear increasing trend in both isoprene (Stavrakou et al., Citation2014). More ground based observations on SO2, O3, NOX, BC, sulfate, secondary organic aerosols are needed to verify the satellite observations and to have a detailed understanding process understanding and complex atmospheric chemistry of these compounds.
2.1.3.1. Urban heat and cool islands
Urban areas modify regional weather and climate patterns in complex and nonlinear ways, so that compared with the surrounding areas, the values of mesoscale (i.e. local scale) meteorological variables are either increased or decreased within an urban planetary boundary layer (PBLs) depending on which of the conflicting impacts wins “the battle” at a given moment. Some of these variables are impacted in only one way including visibility which is always decreased, (mechanical and thermal) turbulence which is always increased and (synoptic and sea breeze) fronts which are always slowed, whereas some other parameters can be either increased or decreased (e.g. precipitation, wind speed). The wind direction either converges into the city or diverges around it, and thunderstorms can be triggered over the city or split (and go around it). Finally, cities are either warmer or cooler than surrounding areas, thus having either an urban heat island (UHI) or urban cool island (UCI), respectively. UHIs form because grass and soil surfaces are replaced by concrete and buildings, which alters surface sensible and latent heat fluxes into the atmosphere. In addition, fossil fuel use in high-latitude cities creates fluxes of pollutants, heat, and water vapor into the atmosphere. Finally, pollutant layers and building-structures decrease incoming solar and outgoing long wave IR terrestrial radiations. A net effect of many of these processes is that cities lose stored solar energy more slowly at night and thus remain warmer, i.e. nocturnal UHIs form; this process is a localized global warming analogy. The above processes can be observed during long-term climatological studies or short-term field campaigns, and can be simulated by the use of highly nonlinear computer codes (Bornstein, Citation1968; Bornstein, Duo, Miao, & Liang, Citation2016)
Urban weather and climate impacts produce important societal impacts, e.g. UHIs produce thermal stress and global-warming enhancements, while precipitation enhancement can produce flooding. In addition, altered urban dispersion (i.e. transport & diffusion) patterns can result in pollution episodes and impact emergency response decisions when reacting to toxic releases in urban atmospheres. Physical mechanisms by which cities alter precipitation patterns have not been completely examined, and future efforts should thus include both climatological and short-term intensive field-studies with PBL observations (Bornstein et al., Citation2016).
2.1.3.2. Urban planetary boundary layer (PBL)
The humankind’s immediate environment is Earth surface together with vegetation or urban canopies and strongly turbulent atmospheric PBL usually separated from weakly turbulent free atmosphere by cupping inversions. The latter restrict turbulence and PBL-free atmosphere exchanges which makes PBLs, microclimates, and air pollution, especially in cities, very sensitive to local impacts. PBL as a physical object together with intrinsic chemical and biological processes at its lover boundary and within PBL comprise a primitive cell of the Climate-Biosphere-Technosphere (CBT) system (Zilitinkevich, Kulmala, Esau, & Baklanov, Citation2015). The key parameters controlling this system are: (1) vertical fluxes at the surface responsible for local resistance, thermal impacts, and emissions (Zilitinkevich et al., Citation2006); (2) vertical fluxes at the PBL upper boundary responsible for PBL-free atmosphere interaction (Zilitinkevich, Citation2012; Zilitinkevich, Elperin, Kleeorin, Rogachevskii, & Esau, Citation2013); (3) PBL height and PBL-free flow exchange controlling sensitivity of CBT call to local impacts (Petäjä et al., Citation2016; Zilitinkevich, Esau, & Baklanov, Citation2007).
Especially critical is the problem of PBL height – yet poorly reproduced in weather, climate and air-quality modeling. We recall that the upper boundary of PBL (ca a few hundred meters) blocks local heating/cooling, emissions and other impacts within CBT cell over hours or even days (Zilitinkevich et al., Citation2015). This is precisely the reason why physical/chemical microclimates are so pronounced and heterogeneous. For homogeneous surfaces, we define horizontal scale of CBT-cell as doubled PBL height, which is just the maximal size of PBL-own motions digesting the finer-scale impacts. For complex surfaces, like urban canopy or broken ground, we attribute CBT-cells to the pronounced patches of landscape with length-scales from dozens to hundreds meters (block of buildings, street-canyon or natural canyon, garden, square, small lake, etc.) characterized by own microcirculations and microclimates.
The bottleneck in understanding and modeling of our immediate environment is insufficient knowledge of interaction between physical, chemical, and biological processes at the Earth surface and within PBL. This is just the reason why climate, biosphere and technosphere taken separately are understood supremely better than CBT as a whole. To resolve the problem, cross-disciplinary efforts are needed focusing on links and feedbacks between plant-physiological, biogeochemical, and physical processes inherent in PBL.
2.2. Land system
Land use and land cover change (LULCC) is primarily characterized as forest conversion to cropland for the development of agriculture. Approximately half of the world’s population is living in monsoon Asia, where the land cover has been dramatically changed by human activities over the past centuries. Many of the previous modeling studies on the climatic effects of land cover change did not consider ocean–atmosphere interactions (e.g. Fu, Citation2003), but recent works suggest that the ocean-atmosphere coupling effect may modulate the magnitude of the climate response to LULCC (Fu et al., Citation2016).
2.2.1. LULCC and changing monsoon dynamics
2.2.1.1. Regional scale
Among various parameters involved in the surface physics, the leaf area index (LAI) affects surface radiation balance, evapotranspiration, and biochemical process of vegetation, etc., and thus is a key parameter that affects surface-atmosphere water and energy exchange and transport (Liu et al., Citation2017). LAI shows a remarkable decrease over eastern China, the Indo-China peninsula, and India. The decrease in LAI is greater in India than in eastern China throughout the year because a higher percentage of forest is converted into cropland in India than in China. This causes a positive temperature-subsidence-moisture feedback (Fu, Citation2003; Fu et al., Citation2016), which likely plays an important role in enhancing the anomalous subsidence over India. Meanwhile, the decrease in the precipitation leads to a drier soil, which increases the land surface albedo and reduces evapotranspiration. This positive precipitation–soil moisture–evaporation feedback enhances the impact of LULCC on the regional climate (Fu et al., Citation2016). The atmospheric responses to LULCC are more obvious in spring and autumn when advection is weaker than in the summer and winter monsoon seasons. LULCC also results in detectable changes in the T2 m variability over India and over the north China–east Mongolia (NCEM) region. In autumn, the LULCC causes an eastward extension of the dry-to-wet transition zone in northern India, where the variability in T2 m is enhanced due to the strengthening land-atmosphere coupling. In winter, LULCC leads to enhanced T2 m variability in India.
The summer monsoon is one of the most unique components of the East Asian climate system. Currently, most regional climate models can realistically simulate the longitudinal movement of the monsoon rain belt in East Asia, whereas precipitation is often overestimated in high-latitudes. Various factors are involved in the ocean-land-atmosphere interaction and affect the intensity and evolution of East Asian summer monsoon. Surface characteristics directly determine the energy budget within a specific region and the surface-atmosphere moisture exchange and transport, and subsequently affect the model capability in regional climate simulation (Xue et al., Citation2004). In fact, the description of surface characteristics is largely focused on accurate representation of land use type and reasonable settings of parameters in surface physics (Figure ).
Continuous field measurements of LAI are relatively rare. The high inhomogeneity of land surface characteristics makes it hard to determine regional-scale LAI based on station observations. Satellite remote sensing can provide spatially and temporally continuous measurements of surface parameters at high-resolution. In recent years, many studies have been conducted to improve simulations of surface temperature and precipitation using satellite remote sensing data. Unfortunately, not many studies have been performed over the East Asian monsoon region. Results indicate that the CTL experiment overestimates precipitation in the semi-arid region of China, southern China, southwestern China, and northern India, while it underestimates precipitation in Huang-Huai River Valley, middle- and lower- Yangzi River valley, the Tibetan Plateau, and northern India Peninsula (Figure ) (Guo, Liu, & Xue, Citation2016).
2.2.1.2. Global scale
Monsoons are annual large-scale phenomena that form an integral part of global-scale circulations. Monsoon circulations are driven and maintained by seasonal contrasts in the thermal heating of land and sea. Beginning in spring, the land warms. Sensible and latent heat are released from the land surface into the atmosphere, driving the formation of low pressure that induces air flow from the ocean. The heating in the land triggers convection, producing clouds and generating rain. The monsoon flow is intensified by convective uplift as well as topographic forcing where mountains exist. These forcings and thermal gradients influence the strength, duration, and spatial distribution of large-scale monsoon systems (e.g. Webster et al., Citation1998; Xue et al., Citation2004). Therefore, land – atmosphere interaction plays a crucial role in monsoon systems by their very nature (Xue & Dirmeyer, Citation2015).
2.2.2. Urbanization and land degradation
The analysis of 30 megacities along the Belt and Road region from 1973 to 2015 demonstrates the city expansion and increased urbanization in the region (Guo, Citation2016). Land degradation is a process where land productive capacity continues to decline or even lost completely under the influence of natural forces and human activities. The scope of land degradation may be extended during this century, which threatens the safety of grain production in China (Gao et al., Citation2016).
2.3. Hydrosphere and cryosphere change over the B&R cold regions
The B&R land Silk Road cross over land High Mountain Asia and the snowy and icy regions of Northeast Asia, which will stretch to the seasonal ice-free area of the Arctic Ocean. The combined impacts of the northern cold regions on global climate may include the opening – up of new sea routes, sea level rising, the albedo feedback and decreasing sea ice extent. Changes in the extent, duration and mass of frozen water in high mountains have multiple and complex impacts on natural environment and human activities, such as on ecosystems, large-scale infrastructure, transportation, growing cities, water supply, hydroelectric power generation, agriculture development, and disaster mitigation. In many parts of the High Mountain and Cold Regions (HiMAC), vulnerability to such changes needs to be reduced by interventions toward adaptation.
The Arctic amplification is the fast climate warming in the Arctic, at least twice as fast as the global average in recent decades (Overland & Wang, Citation2016). The most dramatic changes in the Arctic climate have been the decrease in the sea ice extent, concentration, thickness, as well as the duration of the ice season in the past decades (e.g. Cavalieri & Parkinson, Citation2012).
The Arctic sea routes are very relevant to the commercial sea – bound communication between China and Europe, a core aspect of the B&R. The strong decline of sea ice cover is expected to continue, with estimates of an ice-free Arctic in late summer by 2050 (Overland & Wang, Citation2013). Less and thinner sea ice allows easier navigation with several shorter routes and other off-shore activities in the Arctic, which increases the need for operational marine and weather forecasts and services for route selection and planning of operations.
Central Asia and adjacent areas, such as Russia, western China and its southern neighboring countries, have a highly continental climate. Within the central region of the Eurasian continent, the water resources are mainly from precipitation and supplies from mountain glacier melting. Snow precipitation is an important water resource that balances the water redistribution through the atmosphere transportation. Through the snow depth observations, the snow precipitation accumulation can be estimated statistically using in situ and remote sensing observations. Geographically, the seasonal snow water in the winter covers most of the northern Center Asia, especially Kazakhstan and the Xinjiang Province in China. Over the last decades, global warming and the combined changes in temperature and water vapor had a large influence on the snow water distribution.
The Tibetan Plateau is about the size of Western Europe and supplies water to nearly 2 billion people in Asia as the source of several major rivers, including the Yangze, Mekong, Salween (Gyalmo Ngulchu), Indus, Brahmaputra, and Yellow rivers (Kraaijenbrink et al., Citation2017). Due to climate change, the glaciers are retreating rapidly, grasslands are shrinking as desertification expands, regional precipitation is becoming irregular, water levels in major rivers are dropping and the permafrost is thawing. The melting of Tibetan glaciers, the largest mass of frozen fresh water outside the polar regions, is linked to many environmental consequences both locally and globally, including heat waves in Europe. Chinese officials estimate that Tibet holds 14.5 percent of the world’s total glacier mass. While there are a few different theories on what is causing the glaciers to melt, researchers agree that the pace is staggering. (www.giss.nasa.gov/research/briefs/hansen_14/).
The analysis of time series of Landsat satellite images since the 1970s on the glacier area of the Tibetan Plateau (TP hereafter), evaluated at three study sites where previous measurements over matching periods were available (Mt. Qomolangma (Everest), Mt. Naimona’Nyi, Mt. Geladandong), has demonstrated that the glacier shrinkage on the TP was substantial but spatially variable between the 1970s and 2013. In the 1970s, glaciers covered 1.7% of the TP study area as a whole. The glacier area on the TP has decreased from 44,366 ± 2827 km2 in the 1970s to 42,210 ± 1621 km2 in 2001 (area change was −2156 km2), and further decreased to 41 137 ± 1616 km2 in 2013 (area change was −1073 km2). The glacier shrinkage rate was more marked in marginal (external) drainage basins of the south-east TP than in the interior drainage basins of the north-west TP, from the maximum of −0.43% a−1 (at −1.60% a−1 during 1994–2013) in the Mekong River catchment down to the minimum rate of −0.12% a−1 in the Tarim interior drainage from 1976 to 2013 (Ye et al., Citation2016)
3. Methodology and infrastructure framework to answer research themes
In order to study the research themes introduced in Chapter 2, we need to use several complementary methods and datasets, including satellite remote sensing, in situ measurements and targeted laboratory experiments together with the multi-scale modeling platform (Figure ).
Figure 6. To detect the initial conditions of the atmosphere, climate and air quality we need three components: ground-based observations, satellite observations, and models.
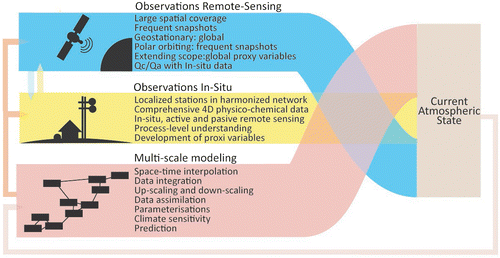
3.1. SMEAR concept
The ground-based observations of the Silk Road region should rely on the observation capacity of the existing station networks and new flagship stations. The geographical location of the in situ networks should cover different environments and climate – vegetation zones, also filling the current observation gap areas (Kulmala, Citation2018). The organization of the existing observation network should be based on the concept of a hierarchy of in situ stations aimed at providing continuous, comprehensive observations of atmospheric composition and ecosystem processes (Hari et al., Citation2016).
The SMEAR measurement concept, developed at the SMEAR II station in Hyytiälä Finland starting from 1995 (Station for Measuring Ecosystem-Atmosphere Relations, Hyytiälä, Finland, Hari & Kulmala Citation2005), provides a conceptual framework for a comprehensive observation system. It also acknowledges and applies the measurements standards of several European Environmental research infrastructures: ICOS (Integrated Carbon Observation System), ACTRIS (European Research Infrastructure for the observation of Aerosol, Clouds, and Trace gases), e-LTER (Long-Term Ecosystem Research) and ANAEE (Analysis and Experimentation on Ecosystems). In addition to atmosphere-biosphere measurements of 1200 standardized variables, the SMEAR concept includes technical descriptions of the data processing and data storage. The station is the best example in the world of the advantages of having the co-location and comprehensive measurements leading to outstanding scientific results (Ref.). The SMEAR-based measurement concept has already opened a new era in understanding the biosphere–atmosphere interactions (Kulmala et al., Citation2014) and in explaining atmospheric new particle formation and growth events (Kulmala et al., Citation2013). The SMEAR concept is based on measurements describing different energy and matter flows and is designed to meet the large-scale research questions (Hari & Kulmala, Citation2008).
3.2. In- situ component and key variables relevant to B&R
The cornerstone the SMEAR-network are comprehensive and continuous ground-based observations. Aerosol particle concentrations, their physical, chemical, and optical properties should be monitored together with a suite of trace gas concentrations. The observation capacity should utilize state-of-the-art techniques, such as Chemical ionization mass spectrometry (e.g. Jokinen et al., Citation2012) and Particle Size Magnifiers (PSM, Vanhanen et al., Citation2011) and follow-up investments on instrument upgrades should be considered to maintain high-quality observations. A tall tower extending at least to 100 m height enables to capture vertical variability for the clusters, aerosols, and trace gases. The in situ observations can be utilized to provide ground-truthing for satellite observations.
The observations should be conducted following the appropriate and established standards, such as ACTRIS, ICOS, and GAW standard operation procedures. Overall, the quality-controlled data series and versatile measurement equipment provide continuous information on the long-term trends in the aerosol, trace gas, and greenhouse gas loadings over the research area.
3.3. Ground-based remote sensing
To complement the ground-based in situ capacity, ground-based remote sensing provides information on the vertical variation and / or column-integrated of aerosol particles, trace gas and greenhouse gas concentrations and meteorological parameters such as temperature and water vapor concentrations, as well as cloud and aerosol parameters. A variety of techniques is used to provide that information, such as lidar (light detection and ranging) where laser pulse is emitted into the atmosphere and backscattered or Raman scattered light are detected by a telescope and electronics receiver system to provide information on the aerosol vertical distribution, as well as the inversion height and cloud base. Specialized systems, such as Differential absorption lidar (DIAL) provide information on trace gas concentrations, such as ozone Max-DOAS systems measure vertical profiles of trace gases and aerosol concentrations based on scattered sunlight. Sun/sky radiometers measure the intensity of direct sunlight irradiation near the surface, at a range of wavelengths, to derive the total atmospheric transmission and from that the aerosol optical depth (AOD) representing the integrated aerosol concentration in the atmospheric column. The wavelength dependence of the AOD, described by the Ångström Exponent (AE), provides information on the aerosol type. This can be compared against in situ derived source apportionment of aerosols. With additional scans in the vertical and almucantar, information becomes available on angular scattering which is used to retrieve aerosol physical properties. Data from ground-based remote sensing, apart from the own value, provide a reference data-set for satellite remote sensing (e.g. Holben et al., Citation1998). The combination of ground-based in situ and remote sensing techniques provides a comprehensive view to the composition of the atmosphere (e.g. Petäjä et al., Citation2016).
3.4. Satellite
3.4.1. Remote sensing and examples of key index relevant to B&R
Satellite data provide information on the spatial variation in atmospheric composition, land surface properties and vegetation as well as on ocean and lakes including snow and ice. Satellite data records for essential climate variables has been introduced by Hollmann et al. (Citation2013) from the European Space Agency perspectives and by Bondur (Citation2016) over Russia region.
In China, three receiving stations are operational as shown in Figure . China Remote Sensing Satellite Ground Station (RSGS) of RADI was established and put into operation in 1986. The RSGS is not only major national science and technology infrastructure but also a member of international ground stations group on Earth observation. As one of the world’s busiest ground stations, RSGS has received, processed, and archived more than 30 satellites, with some 4 million scenes of satellite data since 1986, boasting China’s biggest Earth observation historical database. With four satellite receiving stations located in Miyun, Kashi, Sanya, and Kunming, respectively, RSGS has the capacity to receive real-time satellite data that cover the entire Chinese territory and 70% the land area of Asia (Figure ).
Figure 7. Locations of Miyun (Beijing), Kashi, Sanya, and Kunming Satellite Receiving Stations (RADI).
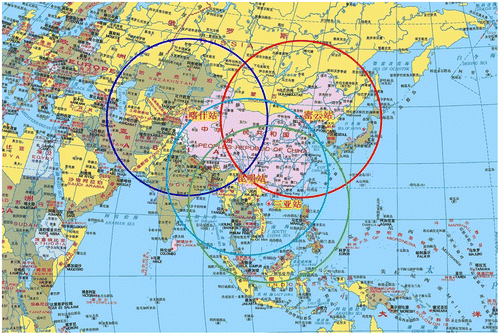
In 1986, RSGS began to receive and process data from LANDSAT-5, a US optical satellite. In 1993, it started to receive and process SAR (synthetic aperture radar) data from the ERS-1 satellite of ESA and the JERS-1 satellite of Japan, realizing all-weather Earth observation. In 1997 and 2008, respectively, RSGS succeeded in receiving and processing data from the RADARSAT-1 and RADARSAT-2 satellites of Canada, with multimode, full polarization capacity. From 1998, RSGS began to receive and process data from the French SPOT series satellites, providing high spatial resolution data to Chinese users. In 2015 RSGS started to receive and process French PLEIADES satellites data, whose spatial resolution is up to 0.6 meter. RSGS has been responsible for receiving data from all China’s Earth observation satellites since 1999. Satellites ever received or being received now are: CBERS-01, CBERS-02, CBERS-02B, HJ-1A, HJ-1B, HJ-1C, ZY-02C, ZY-03, SJ-9A, SJ-9B, GF-1, GF-2, and CBERS-04(english.radi.cas.cn/RD/crssgs/).
For atmospheric monitoring, various techniques are applied utilizing solar radiation reflected from the Earth system, i.e. surface and atmosphere at wavelengths in the ultraviolet (UV), visible (VIS), and near infrared (NIR) part of the electromagnetic system, and Earth emitted thermal infrared radiation (TIR). Radiometers designed for the retrieval of aerosol and cloud properties, such as MODIS, ideally have wavebands in each of these wavelengths regions, where TIR, together with other wavebands, is used for cloud detection and those in the UV–vis are used for aerosol retrieval (Levy et al., Citation2013; Sayer et al., Citation2014). Ideally, aerosol sensors have multiple viewing angles (MISR) and polarization (POLDER), which allow for the retrieval of aerosol properties such as layer height (Flower & Kahn, Citation2017; Kahn et al., Citation2007) and information on aerosol types (Kahn & Gaitley, Citation2015). The GRASP algorithm (Dubovik et al., Citation2011) applied to PARASOL data is proposed to be used as reference data-set (Popp et al., Citation2016). Other sensors, which were proposed for other purposes than the retrieval of aerosols and clouds but do provide quality aerosol information, include the ATSR-series (Along-Track Scanning Radiometer) of dual view instruments: ATSR-2 in ERS-2 (1995–2003), the Advanced ATSR (AATSR) on ENVISAT (2002–2012) and the Sea and Land Surface Temperature Radiometer (SLSTR) on Sentinel-3 (launched in 2016). ATSR and MODIS were used to provide two decades of AOD data over China (de Leeuw et al., Citationin press). The longest AOD time series are provided by the AVHRR series which have recently been retrieved over land (1983-present, Xue et al., Citation2017), see also Hsu et al. (Citation2017). Another long time series, for the absorbing aerosol index (AAI), a qualitative parameter which provides information on the occurrence of absorbing aerosol, is provided by spectrometers such as TOMS, SCIAMCHY, GOME-2, and OMI. TIR instruments such as IASI provide information on, e.g. dust aerosol and volcanic ash.
Optical instruments are not sensitive to particles smaller than about 100 nm (Sundström et al., Citation2015) and therefore the production of new aerosol particles through GTP cannot be directly observed. However, considering the processes a scheme was presented by Kulmala et al. (Citation2011) for the use of satellite data to detect nucleation on a global scale. This method utilizes NO2 and SO2 vertical column concentrations and UV radiation available from the Ozone Monitoring Instrument OMI aboard the Aura satellite which was launched in 2004. In 2016 the Tropomi instrument, an advanced instrument following up on OMI with better resolution and more channels, was launched aboard Sentinel-5P. OMI and Tropomi provide information on, e.g. O3, CH4, atmospheric trace gases and aerosol (AAI and layer height) (www.tropomi.eu/data-products) with a sub-nadir pixel of about 3x7 km2. First data show a very good data quality which allows observations on sub-city level.
MODIS AOD and cloud information are used together to obtain information on aerosol-cloud interaction. It appears that in conditions with very high pollution levels, such as those occurring sometimes over China, the effects of aerosols on cloud properties is different from those in cleaner conditions (Liu et al., Citation2017).
3.4.2. Drought index (MIDI)
Drought is one of the most common and costliest natural disasters, and has adversely affected water resources, agricultural production, and welfare of people especially in semi-arid regions, where precipitation limitation is already at high risk. Furthermore, semi-arid vegetation productivity is mainly constrained by precipitation, and further limited by temperature in mid- latitudes. Reliable monitoring of drought and its impact on vegetation at regional scale is essential and lacking. Moreover, it is also an important scientific issue of climate change and regional responses. To date, studies have been mainly based on investigating drought and its impact using meteorological data and ground observations, but the usage of satellite data is still limited. Recent studies has been focused on monitoring regional drought and its connection with vegetation using multi-sensor satellite based Microwave Integrated Drought Index (MIDI) and vegetation indicators over semi-arid ecosystem. The MIDI can consistently and continuously monitor drought cross space and time in all weather conditions. Spatial-temporal comparisons among MIDI, climate variables (precipitation and temperature), drought indices, and vegetation indices (Normalized Difference Vegetation Index, NDVI) over different semi-arid areas were carried out. In general, the spatial patterns of drought and vegetation trend were heterogeneous, while drought intensified accompanied by higher temperature and lower precipitation. The vegetation (NDVI) showed similar trend and variation as MIDI with lagged responses. These results are valuable for further investigating the impact of drought on vegetation especially over semi-arid ecosystems, and providing us operational tool for drought and its impacts detection based on satellite remote sensing.
3.4.3. Normalized Difference Vegetation Index (NDVI)
In recent years, satellite sensors have become an important tool for ecosystem mapping and monitoring at regional to global scales. The advanced very high resolution radiometer (AVHRR) is the most important source of remotely sensed data that include continuous global cover from 1981 to now, and is the major component of the long-term data record data-sets consisting of the Moderate Resolution Imaging Spectroradiometer visible infrared imaging radiometer suite (AVHRR-MODISVIIRS). The long-image time series of the normalized difference vegetation index (NDVI) data-sets generated from the AVHRR has been produced by the global inventory modeling and mapping studies (GIMMS) group and has become an important tool for understanding patterns of ecosystem and vegetation for providing temporally continuous observations at regional and global scales . The third-generation NDVI data-sets (GIMMS NDVI3 g) were recently released, providing a revised and updated extension of a previous NDVI version (GIMMS NDVIg). The NDVI3 g was carefully assembled from several AVHRR sensors, accounting for several deleterious effects, such as calibration loss, orbit drift, and volcanic eruption. The GIMMS NDVI3 g data-set especially aims to improve data quality for high latitudes, where the growing season is less than two months. It has also improved calibration tied to the Sea-Viewing Wide-Field-of-View-Sensor, as opposed to earlier versions of GIMMS NDVI data-sets that were based on inter-calibration with the SPOT sensor. Quantitative analysis has also shown how the NDVI3 g data-set captures seasonal variation where phenology is variable. It is thus important to compare the two NDVI data-set versions (NDVIg and NDVI3 g) to link existing studies with future applications of the NDVI3 g in monitoring vegetation activity change (Zhang, Zhang & Yuan, Citation2016).
3.4.4. Aerosol index
The aerosol index (AI) is the product of the AOD and the AE which provides information on the fine mode fraction of the AOD, i.e. the occurrence of sub-micron aerosol particles. However, it should be noticed that the AE is not an accurate parameter and has a much higher uncertainty, especially in low AOD conditions.
Cloud properties such as cloud fraction (CF), cloud optical thickness (COT), cloud droplet effective radius (CDR), cloud top pressure (CTP) and height (CTH), cloud liquid water path (CWO) can be obtained from satellites and from ground-based measurements with lidars and cloud radars. However, it should be noticed that satellites observe cloud properties from above and thus are sensitive to cloud top properties, whereas ground-based measurements are dominated by those at cloud base. This applies to optical measurements which have a small penetration depth. Radars work with longer wavelengths and therefore can look deeper into the cloud. Together, such measurements provide information on aerosol-cloud interaction. Ground-based and satellite measurements can also be used separately for such studies, as discussed briefly for satellites above (e.g. Liu et al., Citation2017).
3.5. Laboratory experiments – capacity to understand processes
PEEX is interested in continuous observations of the atmospheric composition including meteorology, trace gas concentrations, aerosol mass concentration, black carbon concentration, aerosol physical characterization with a particular focus on gas-to-particle conversion and aerosol formation in the atmosphere over the B&R region. One of the first research objectives would be a detailed analysis of atmospheric oxidation mechanisms and trace gas characterization with high-resolution mass spectrometry to resolve secondary aerosol processes in urban megacity environment. Reaction CLOUD-type chamber; (CERN The Cosmics Leaving Outdoor Droplets (CLOUD) experiment uses a special cloud chamber to study the possible link between galactic cosmic rays and cloud formation) is needed to explore and quantify the role of different trace gases in gas-to-particle conversion in controlled environment in controlled environment. The high resolution mass spectrometers would provide us the data on clustering process molecule by molecule.
3.6. Modeling platform
The PEEX Modeling Platform is a part of the PEEX Infrastructure. Since 2014, this platform has utilized more than 30 models at different scales, resolutions, domains, etc. and used as research tools capable to provide insights and valuable information for PEEX geographical regions in focus, and in particular, for China. Platform is based on a seamless approach to evolve from separate model components to seamless meteorology-composition-environment models to address challenges in weather, climate, and atmospheric composition as well as assessments for environment and population. Several dimensions are considered such as processes, components, scales, and tools. The integrated seamless approach combines specific models considering different processes at different temporal and spatial scales. These models are validated using available in situ, ground-based, and remote sensing data. Because expected large volumes of data (produced from modeling and observations), these will be supported using dedicated virtual research environment developing in PEEX.
The measurements in SMEAR Beijing and SORPES (Station for Observing Regional Processes of the Earth System) in Nanjing together with existing measurements in Finland (Värriö, Hyytiälä, Helsinki, Kuopio, GAW-Pallas) will provide a unique basis to diversify the existing aerosol, trace-gas and GHG measurements into different environments which, besides enhancing our scientific understanding, will provide crucial information for global climate models and regional air quality models ( Schutgens & Stier, Citation2014; Zhang, Bocquet, Mallet, Seigneur, & Baklanov, Citation2012). With these data and associated meta data, it will be possible to find out long-term changes in China.
The examples of the tools span from atmospheric clusters to regional air quality, global climate, and Earth System Models (ESMs) and may include cluster models like ACDC (McGrath et al., Citation2012), boundary layer models like SOSA (Boy et al., Citation2011) coupled with models simulating both aerosol dynamics and gas-phase chemistry like ADCHAM (Roldin et al., Citation2015) and MALTE-BOX (Boy et al., Citation2013), chemical transport models like WRF-Chem (Grell et al. Citation2005), seamless meteorology-chemistry-aerosols integrated model like Enviro-HIRLAM (Baklanov et al., Citation2017), and Earth System models like EC-EARTH (Hazeleger et al., Citation2012). Earth System Models are used to assess the impact of Russian and Chinese emissions on the boreal and the Arctic atmosphere between 1750 and 2100. Furthermore, interactive vegetation components of ESMs allow for the quantification of the feedbacks and particularly the effect of nano-GTP on it under varying driving forces (increasing GHGs vs. decreasing air pollution).
Seamless modeling approach of both meteorology and atmospheric composition provides additional value for the PEEX-related studies. For example, the Enviro-HIRLAM model is applied in both the research and operational modes for selected domains of China (in FP7 EU MarcoPolo project; http://www.marcopolo-panda.eu and continued under PEEX). In particular, it has been realized with a downscaling approach with operational forecasting at resolutions of 15, 5, and 2.5 km (regional– subregional – urban scale). Such runs are performed at CRAY-XC30 HPC with output available at: http://www.marcopolo-panda.eu/products/regional-air-quality-forecasts/enviro-hirlam). The entire downscaling chain is running twice per day at 00 & 12 UTCs for the full 48 h forecast length (with intermediate runs at 06 & 18 UTCs for 9 h forecast length). An example of such operational forecast is shown in Figure . The CPU time required for 1 run of the chain is about 7 + hours, disk space usage – 71 + Gb, using 208 mpi-tasks on 13 nodes.
3.7. Data system of the SMEAR typed stations
Raw and processed data will be saved in the centralized global smear database, in the smartSMEAR platform (Junninen et al., Citation2009). This enables development of new data processing algorithms and data updating without changing the existing database and reference to the data used, e.g. in publications is maintained. Saving multiple versions of the data with quality and processing level flags ensures data quality and transparency. As the data sets are large, various data mining techniques will be utilized, like clustering, factor analysis, discriminant analysis (e.g. Hyvönen et al., Citation2005). The SmartSMEAR enables parallel visualization of comprehensive atmospheric composition together with source apportionment and air mass trajectories (Figure ).
Figure 8. Enviro-HILRAM operational PM2.5 concentration forecasts for China in a downscaling chain (left-right: regional, sub-regional, urban – Shanghai metropolitan area) for 21 January 2018, 22 UTC.
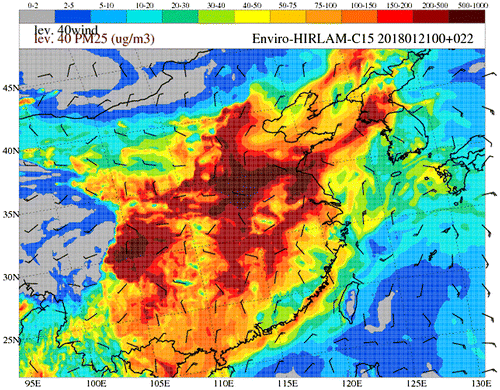
In a framework of data collaboration with the Russian and Chinese partners, we will promote and endorse Future Earth open data policies (Future Earth, Citation2014) and international coordination of the data formats and products. The Strategic Research Agenda states that in order to get a holistic understanding of the Earth system, open, and inclusive platforms for observing trends and thresholds of the planet are needed at different scales. SmartSMEAR serves as a platform for open data policy and data delivery to and sharing with collaborators and end-users. It also promotes the Future Earth 2025 vision that is also presented at the Strategic Research Agenda.
4. PEEX collaboration with Chinese initiatives
The PEEX-China agenda is contributing to topics introduced in the DBAR Science Plan (DBAR. A Science Plan for Digital Belt & Road Program [DBAR], Citation2017), which is having a special focus on climate change, urbanization, and research infrastructures providing big data on Earth System (Figure ). DBAR is the main collaborator of PEEX-China. DBAR and PEEX-China complementary expertise for establishing the big data pool for the B&R regions. DBAR is bringing together remote sensing communities across the B&R domain, while PEEX is interested in establishing a comprehensive ground based in situ observation network. The DBAR Science Foci has many synergies with the PEEX research agenda and selected focus areas. DBAR set its main scientific objective on the society relevant regional aspects and PEEX is more focused on understanding feedbacks between B&R regional changes and the climate system. The International Eurasian Academy of Sciences (IEAS) and the International Silk Road Academy of Sciences are providing a large scale collaboration framework for carrying out the PEEX-China agenda. The International Eurasian Academy of Sciences (IEAS) has an official UNESCO partnership and the IEAS China Scientific Center (Pacific Centre) and International Silk Road Academy of Sciences are hosted by Chinese Academy of Sciences (CAS).
Figure 9. The PEEX-China agenda is contributing to topics introduced in the DBAR Science Plan having a special focus on climate change, urbanization, and research infrastructures providing big data.
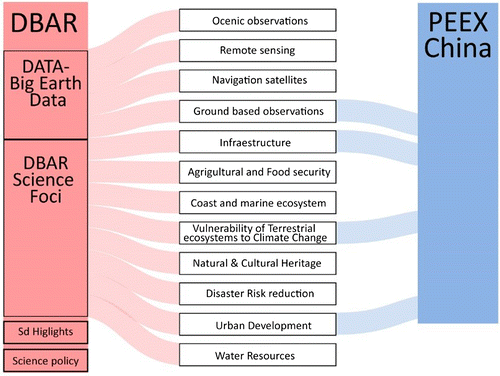
The DBAR Task force on the High Mountain and Arctic Cold Regions (DBAR-HiMAC; see Yubao et al., Citation2016), which is affiliated with Institute of Remote Sensing and Digital Earth, Chinese Academy of Sciences (RADI-CAS), has been developed in collaboration with the Arctic Space Center of Finnish Meteorological Institute (FMI). The activity aims on the research data exchange between FMI ASC satellite data archive system and Chinese satellites. The activity is conducting the satellite-derived geophysical product and information service for cold regions regarding the common Area of Interest (AOI) over cold regions. The interests include, but not limited in, the snow, permafrost, soil freezing/thaw, lake ice, and sea ice monitoring, and it’s processing with the Big Earth Data technologies. Those efforts will be also linked with the GEOSS GEO Cold Regions also at an international level, which are also being with the DBAR-PEEX International Center of Excellence in University of Helsinki.
5. Future views
The new understanding of the land–atmosphere interactions together with the coordinated, comprehensive observation system in the Silk Road domain has significant societal and economic impact at regional and global scales. The research themes “Air-quality – climate interactions” and “Melting of Tibetan Plateau glaciers” are connecting the processes taking place in the Silk Road region to the global climate system and vice versa. The changing climate and weather conditions are effecting everyday living conditions of millions of people and the world economy. The quantification of the large scale interaction and feedbacks between air quality and climate in the Asian geographical domain is fundamental information for understanding the climate change at the global scale. At the regional scale, increasing extreme climate events, frequent occurrence of major natural disasters, sea level rise, eco-environmental degradation, and water resource shortages are associated to the future B&R risks.
The process understanding of the melting of the Tibetan glaciers is one of the most crucial aspects of the successful B&R implementation. Understanding the air quality climate feedback and multiple linkages related to the melting of Tibetan Plateau glaciers also the backbone for carrying out the B&R successfully and ensuring that the new infrastructure is functioning under changing environmental conditions. The design of the transport corridors and other infrastructure needs most reliable predictions of the water system changes of the Tibetan regions and target areas at wider scale.
The themes “Urban air quality” and “LULCC and land-atmosphere climate interactions” are more focused on the regional scale and require information on urban air quality and heat islands. In addition to health effects, the pollution also reduces visibility and is thereby decreasing the attraction of the mega-cities for tourists, and hindering the possibilities to use solar energy as a source for a clean energy on a local scale. The role of LULCC is associated with urbanization and land degradation and is addressing a serious risk for the food production of the area.
As a summary the PEEX B&R agenda is addressing the research agenda and research infrastructure in the selected topical areas, which are needed to find practical solutions to ensure the sustainable development of the B&R environment, society and economy. For example, the China Government is underway to reduce the SO2, NOx, CO, and Particulate mass (PM) emissions in the 20–1000 nm size range. However it is important to recognize that due to multiple interactions between the chemical and physical environments the reduction of one pollutant can increase the concentrations of others. Hasty reduction solutions might improve the air quality only temporarily (Kulmala et al., Citation2015). Deep understanding of the composition of the complex urban atmospheric cocktail enables the establishment of sustainable air quality controlling mechanism in megacities. The improved air quality has direct health effects on the local populations. It also improves the profits of many economics sectors such as tourism. The decreased deposition of air pollutants improves the quality and amount of food production.
In Figure we introduce the PEEX B&R roadmap with a tentative timeline of separate phases. The first step is a joint science plan between PEEX and DBAR initiatives including plans for the in situ station network-based SMEAR concept and a service specification. These plans are needed for attracting government and / or private sector investments.
Disclosure statement
No potential conflict of interest was reported by the authors.
Funding
This work was support from the Academy of Finland Center of Excellence [grant number 307331], the Academy of Finland Academy Professor [grant number 307567], ERC Advanced grant [project ID 742206], Digital Belt & Road of CAS Strategic Priority Research Program [grant number XDA19030402], the Academy of Finland projects ABBA No. 280700 (2014–2017) and ClimEco No. 314 798/799 (2018–2020) and Russian Science Foundation projects No. 15-17-20009 (2015–2018) and No. 15-17-30009 (2015–2018).
Acknowledgements
Our sincere thanks for Dr Nuria Altimir, University of Helsinki, PEEX HQ Office, for the production of Figures .
Data availability statement
Data sharing is not applicable to this article as no new data were created or analysed in this study.
References
- Baklanov, A. , Smith Korsholm, U. , Nuterman, R. , Mahura, A. , Nielsen, K. P. , Sass, B. H. , … González-Aparicio, I. (2017). Enviro-HIRLAM online integrated meteorology–chemistry modelling system: Strategy, methodology, developments and applications (v7.2). Geoscientific Model Development , 10 , 2971–2999. doi:10.5194/gmd-10-2971-2017
- Bondur, V.G. (2016). Satellite monitoring of trace gas and aerosol emissions into the atmosphere of Northern Eurasia. In H. K. Lappalainen , A. Borisova , D. Liang , J. Enroth , and M. Kulmala (Eds.), Proceedings of the 2nd Pan-Eurasian Experiment (PEEX) Conference and the 6th PEEX Meeting, Report Series in Aerosol Science (Vol. 180, pp. 53–52).
- Bornstein, B. , Duo, J. , Miao, S. , & Liang, X. (2016). Guidelines for analysis of urban weather and climate data. In H. K. Lappalainen , A. Borisova , D. Liang , J. Enroth , & M. Kulmala (Eds.), Proceedings of the 2nd Pan-Eurasian Experiment (PEEX) Conference and the 6th PEEX Meeting Report Series in Aerosol Science (Vol. 180, pp. 55–61).
- Bornstein, R. (1968). Observations of the urban heat island effect in NYC. Journal of Applied Meteorology , 7 , 575–582.10.1175/1520-0450(1968)007<0575:OOTUHI>2.0.CO;2
- Boy, M. , Mogensen, D. , Smolander, S. , Zhou, L. , Nieminen, T. , Paasonen, P. , … Kulmala, M. (2013). Oxidation of SO2 by stabilized Criegee intermediate (sCI) radicals as a crucial source for atmospheric sulfuric acid concentrations. Atmospheric Chemistry and Physics , 13 , 3865–3879.10.5194/acp-13-3865-2013
- Boy, M. , Sogachev, A. , Lauros, J. , Zhou, L. , Guenther, A. , & Smolander, S. (2011). SOSA – A new model to simulate the concentrations of organic vapours and sulphuric acid inside the ABL- Part 1: Model description and initial evaluation. Atmospheric Chemistry and Physics , 11 , 43–51.10.5194/acp-11-43-2011
- Crounse, J. D. , Nielsen, L. B. , Jørgensen, S. , Kjaergaard, H. G. , & Wennberg, P. O. (2013). Autoxidation of organic compounds in the atmosphere. The Journal of Physical Chemistry Letters , 4 , 3513–3520.10.1021/jz4019207
- DBAR. A Science Plan for Digital Belt and Road Program (DBAR) . (2017). An international science program for sustainable development of the belt and road region using big earth data, released in Hongkong, China .
- de Leeuw, G. , Sogacheva, L. , Rodriguez, E. , Kourtidis, K. , Georgoulias, A. K. , Alexandri, G. , … van der, A. R. (in press). Two decades of satellite observations of AOD over mainland China. Atmospheric Chemistry Physics Discussion . doi:10.5194/acp-2017-838
- Ding, A. J. , Fu, C. B. , Yang, X. Q. , Sun, J. N. , Petäjä, T. , Kerminen, V.-M. , … Kulmala, M. (2013). Intense atmospheric pollution modifies weather: A case of mixed biomass burning with fossil fuel combustion pollution in eastern China. Atmospheric Chemistry and Physics , 13 , 10545–10554. doi:10.5194/acp-13-10545-2013
- Ding, A. J. , Wang, T. , Thouret, V. , Cammas, J.-P. , & Nédélec, P. (2008). Tropospheric ozone climatology over Beijing: analysis of aircraft Atmos. Chem.data from the MOZAIC program. Atmos. Chem. Phys. , 8 , 1–13. doi:10.5194/acp-8-1-2008.
- Ding, A. J. , Fu, C. B. , Yang, X. Q. , Sun, J. N. , Zheng, L. F. , Xie, Y. N. , … Kulmala, M. (2013). 2013b: Ozone and fine particle in the western Yangtze River Delta: An overview of 1 yr data at the SORPES station. Atmospheric Chemistry and Physics , 13 , 5813–5830. doi:10.5194/acp-13-5813-
- Dubovik, O. , Herman, M. , Holdak, A. , Lapyonok, T. , Tanré, D. , Deuzé, J. L. , … Lopatin, A. (2011). Statistically optimized inversion algorithm for enhanced retrieval of aerosol properties from spectral multi-angle polarimetric satellite observations. Atmospheric Measurement Techniques , 4 , 975–1018.10.5194/amt-4-975-2011
- Ehn, M. , Thornton, J. A. , Kleist, E. , Sipilä, M. , Junninen, H. , Pullinen, I. , … Mentel, T. F. (2014). A large source of low-volatility secondary organic aerosol. Nature , 506 , 476–479.10.1038/nature13032
- Fiore, A. M. , Naik, V. , Spracklen, D. V. , Steiner, A. , Unger, N. , Prather, M. , … Zeng, G. (2012). Global air quality and climate. Chemical Society Reviews , 41 , 6663–6683.10.1039/c2cs35095e
- Flower, V. J. , & Kahn, R. A. (2017). Assessing the altitude and dispersion of volcanic plumes using MISR multi-angle imaging from space: Sixteen years of volcanic activity in the Kamchatka Peninsula, Russia. Journal of Volcanology and Geothermal Research , 337 , 1–15. doi:10.1016/j.jvolgeores.2017.03.010
- Fu, C. (2003). Potential impacts of human-induced land cover change on East Asia monsoon. Global and Planetary Change , 37 (3–4), 219–229.
- Fu, C. , Xu, Z. , Mahmood, R. , Yang, Z. L. , Su, H. , Guo, W. , & Wan, S. (2016). Towards further understanding the impacts of land use and cover change on regional climate of monsoon Asia. In H. K. Lappalainen , A. Borisova , D. Liang , J. Enroth , and M. Kulmala (Eds.), Proceedings of the 2nd Pan-Eurasian Experiment (PEEX) Conference and the 6th PEEX Meeting, Report Series in Aerosol Science (Vol. 180, pp. 153–157).
- Future Earth . (2014). Future earth strategic research agenda 2014 . Paris: International Council for Science (ICSU).
- Fuzzi, S. , Baltensperger, U. , Carslaw, K. , Decesari, S. , Denier van der Gon, H. D. , Facchini, M. C. , … Gilardoni, S. (2015). Particulate matter, air quality and climate: Lessons learned and future needs. Atmospheric Chemistry and Physics , 15 , 8217–8299.10.5194/acp-15-8217-2015
- Gao, Z. H. , Sun, B. , & Li, Z. Y. (2016). Land degradation assessment by coupling npp and climate in Xilin gollegue, inner Monglia, China. In H. K. Lappalainen , A. Borisova , D. Liang , J. Enroth , and M. Kulmala (Eds.), Proceedings of the 2nd Pan-Eurasian Experiment (PEEX) Conference and the 6th PEEX Meeting, Report Series in Aerosol Science (pp. 159–162).
- Grell, G. A. , Peckham, S. E. , Schmitz, R. , McKeen, S. A. , Frost, G. , Skamarock, W. C. , & Eder, B. (2005). Fully coupled “online” chemistry within the WRF model. Atmospheric Environment , 39 , 6957–6975.10.1016/j.atmosenv.2005.04.027
- Guo, S. , Hu, M. , Zamora, M. L. , Peng, J. F. , Shang, D. J. , Zheng, J. , … Zhang, R. Y. (2014). Elucidating severe urban haze formation in China. Proceedings of the National Academy of Sciences , 111 , 17373–17378.10.1073/pnas.1419604111
- Guo, H. D. (2016). Space borne earth observing technologies for environmental monitoring in the belt and road region. In H. K. Lappalainen , A. Borisova , D. Liang , J. Enroth , and M. Kulmala (Eds.), Proceedings of the 2nd Pan-Eurasian Experiment (PEEX) Conference and the 6th PEEX Meeting, Report Series in Aerosol Science (Vol. 180, pp. 168–169).
- Guo, J. , Su, T. , Li, Z. , Miao, Y. , Li, J. , Liu, H. , … Zhai, P. (2017). Declining frequency of summertime local-scale precipitation over eastern China from 1970 to 2010 and its potential link to aerosols. Geophysical Research Letters , 44 , 5700–5708.
- Guo, W. , Liu, Y. , & Xue, Y. (2016). Impact of accurate description of land use type and vegetation on simulation of the East Asian monsoon precipitation. In H. K. Lappalainen , A. Borisova , D. Liang , J. Enroth , and M. Kulmala (Eds.), Proceedings of the 2nd Pan-Eurasian Experiment (PEEX) Conference and the 6th PEEX Meeting, Report Series in Aerosol Science (Vol. 180, pp. 172–173).
- Hari, P. , & Kulmala, M. (2005). Station for measuring ecosystem-atmosphere relations (SMEAR II). Physical and Physiological Forest Ecology , 10 , 315–322.
- Hari, P. , & Kulmala, L. , (Ed.). (2008). Boreal forest and climate change (582 pp., Vol. 34). New York, NY : Springer-Verlag.10.1007/978-1-4020-8718-9
- Hari, P. , Petäjä, T. , Bäck, J. , Kerminen, V. M. , Lappalainen, H. K. , Vihma, T. , … Kulmala, M. (2016). Conceptual design of a measurement network of the global change. Atmospheric Chemistry and Physics , 16 , 1017–1028.10.5194/acp-16-1017-2016
- Hazeleger, W. , Wang, X. , Severijns, Stefanescu S. , Bintanja, R. , Sterl, A. , Wyser, K. , … van der Wiel, K. (2012). EC-Earth V2.2: Description and validation of a new seamless earth system prediction model. Climate Dynamics , 39 , 2611–2629.10.1007/s00382-011-1228-5
- Holben, B. N. , Eck, T. F. , Slutsker, I. , Tanré, D. , Buis, J. P. , Setzer, A. , … Nakajima, T. (1998). AERONET – A federated instrument network and data archive for aerosol characterization. Remote Sensing of Environment , 66 , 1–16.10.1016/S0034-4257(98)00031-5
- Hollmann, R. , Merchant, C. , Saunders, R. , Downy, C. , Buchwitz, M. , Cazenave, A. , … Wagner, W. (2013, October). The ESA climate change initiative: Satellite data records for essential climate variables. Bulletin of the American Meteorological Society , 1541–1552. doi:10.1175/BAMS-D-11-00254.1 10.1175/BAMS-D-11-00254.1
- Hsu, N. C. , Lee, J. , Sayer, A. M. , Carletta, N. , Chen, S. H. , Tucker, C. J. , … Tsay, S. C. (2017). Retrieving near-global aerosol loading over land and ocean from AVHRR. Journal of Geophysical Research: Atmospheres , 122 . doi:10.1002/2017JD026932
- Huang, R. J. , Zhang, Y. , Bozzetti, C. , Ho, K. F. , Cao, J. J. , Han, Y. , … Zotter, P. (2014). High secondary aerosol contribution to particulate pollution during haze events in China. Nature , 514 , 218–222.10.1038/nature13774
- Hyvönen, S. , Junninen, H. , Laakso, L. , Dal Maso, M. , Grönholm, T. , Bonn, B. , … Kulmala, M. (2005). A look at aerosol formation using data mining techniques. Atmospheric Chemistry and Physics , 5 , 3345–3356.10.5194/acp-5-3345-2005
- IPCC . (2013). Climate Change: The Physical Science Basis. Working Group I Contribution to the Fifth Assessment Report of the Intergovernmental Panel on Climate Change (T. F. Stocker, D. Qin, G.-K. Plattner, M. Tignor, S. K. Allen, J. Boschung, A. Nauels, Y. Xia, V. Bex, & P. M. Midgley, Eds.). Cambridge and New York, NY: Cambridge University Press.
- Jokinen, T. , Sipilä, M. , Junninen, H. , Ehn, M. , Lönn, G. , Hakala, J. , … Worsnop, D. R. (2012). Atmospheric sulphuric acid and neutral cluster measurements using CI-APi-TOF. Atmospheric Chemistry and Physics , 12 , 4117–4125.10.5194/acp-12-4117-2012
- Junninen, H. , Lauri, A. , Keronen, P. , Aalto, P. , Hiltunen, V. , Hari, P. , & Kulmala, M. (2009). Smart-SMEAR: On-line data exploration and visualization tool for SMEAR stations. Boreal Environment Research , 14 , 447–457.
- Kahn, R. A. , Li, W. H. , Moroney, C. , Diner, D. J. , Martonchik, J. V. , & Fishbein, E. (2007). Aerosol source plume physical characteristics from space-based multiangle imaging. Journal of Geophysical Research: Atmospheres , 112 (D11).
- Kahn, R. A. , & Gaitley, B. J. (2015). An analysis of global aerosol type as retrieved by MISR. Journal of Geophysical Research: Atmospheres , 120 . doi:10.1002/2015JD023322
- Kirkby, J. , Duplissy, J. , Sengupta, K. , Frege, C. , Gordon, H. , Williamson, C. , … Curtius, J. (2016). Ion-induced nucleation of pure biogenic particles. Nature , 533 , 521–526.10.1038/nature17953
- Kraaijenbrink, P. D. A. , Bierkens, M. F. P. , Lutz, A. F. , & Immerzeel, W. W. (2017). Impact of a global temperature rise of 1.5 degrees Celsius on Asia’s glaciers. Nature , 549 (7671), 257–260.10.1038/nature23878
- Kulmala, M. (2015). Atmospheric chemistry: China’s choking cocktail. Nature , 526 , 497–499.10.1038/526497a
- Kulmala, M. (2018). Build a global Earth observatory. Nature , 553 , 21–23.10.1038/d41586-017-08967-y
- Kulmala, M. , Arola, A. , Nieminen, T. , Riuttanen, L. , Sogacheva, L. , de Leeuw, G. , … Lehtinen, K. E. J. (2011). The first estimates of global nucleation mode aerosol concentrations based on satellite measurements. Atmospheric Chemistry and Physics , 11 (10791–10801), 2011. doi:10.5194/acp-11-10791-2011
- Kulmala, M. , Lappalainen, H. K. , Petäjä, T. , Kurten, T. , Kerminen, V. M. , Viisanen, Y. , … Zilitinkevich, S. (2015). Introduction: The Pan-Eurasian Experiment (PEEX) – multidisciplinary, multiscale and multicomponent research and capacity-building initiative. Atmospheric Chemistry and Physics , 15 , 13085–13096.10.5194/acp-15-13085-2015
- Kulmala, M. , Kerminen, V.-M. , Petäjä, T. , Ding, A. J. , & Wang, L. (2017). Atmospheric gas-to-particle conversion: Why NPF events are observed in megacities? Faraday Discussions , 200 , 271–288. doi:10.1039/c6fd00257a
- Kulmala, M. , Kontkanen, J. , Junninen, H. , Lehtipalo, K. , Manninen, H. E. , Nieminen, T. , … Worsnop, D. R. (2013). Direct observations of atmospheric aerosol nucleation. Science , 339 , 943–946.10.1126/science.1227385
- Kulmala, M. , Petäjä, T. , Ehn, M. , Thornton, J. , Sipilä, M. , Worsnop, D. R. , & Kerminen, V. M. (2014). Chemistry of atmospheric nucleation: On recent advances on precursor characterization and atmospheric cluster composition in connection with atmospheric new particle formation. Annual Review of Physical Chemistry , 65 , 21–37.10.1146/annurev-physchem-040412-110014
- Kulmala, M. , Petaja, T. , Kerminen, V. M. , Kujansuu, J. , Ruuskanen, T. , Ding, A. J. , … Worsnop, D. R. (2016a). On secondary new particle formation in China. Frontiers of Environmental Science & Engineering , 10 . doi:10.1007/s11783-016-0850-1
- Lappalainen, H. K. , Kerminen, V.-M. , Petäjä, T. , Kurten, T. , Baklanov, A. , Shvidenko, A. , … Kulmala, M. (2016). Pan-Eurasian Experiment (PEEX): Towards a holistic understanding of the feedbacks and interactions in the land–atmosphere–ocean–society continuum in the northern Eurasian region. Atmospheric Chemistry and Physics , 16 , 14421–14461. doi:10.5194/acp-16-14421-2016
- Lappalainen, K. H. , Petaja, T. , Kujansuu, J. , Kerminen, V. M. , Shvidenko, A. , Back, J. , … Ruuskanen, T. (2014). Pan-Eurasian Experiment (PEEX) – a research initiative meeting the grand challenges of the changing environment of the northern Pan-Eurasian arctic-boreal areas. Geography, Environment, Sustainability , 2 , 13–48.
- Lelieveld, J. , Evans, J. S. , Fnais, M. , Giannadaki, D. , & Pozzer, A. (2015). The contribution of outdoor air pollution sources to premature mortality on a global scale. Nature , 525 , 367–371.10.1038/nature15371
- Levy, R. C. , Mattoo, S. , Munchak, L. A. , Remer, L. A. , Sayer, A. M. , Patadia, F. , & Hsu, N. C. (2013). The Collection 6 MODIS aerosol products over land and ocean. Atmospheric Measurement Techniques , 6 , 2989–3034. doi:10.5194/amt-6-2989-2013
- Liu, Y. , de Leeuw, G. , Kerminen, V.-M. , Zhang, J. , Zhou, P. , Nie, W. , … Petäjä, T. (2017). Analysis of aerosol effects on warm clouds over the Yangtze River Delta from multi-sensor satellite observations. Atmospheric Chemistry and Physics , 17 , 5623–5641. doi:10.5194/acp-17-5623-2017
- Maji, K. J. , Dikshit, A. K. , Arora, M. , & Deshpande, A. (2018). Estimating premature mortality attributable to PM2.5 exposure and benefit of air pollution control policies in China for 2020. Science of The Total Environment , 612 , 683–693.10.1016/j.scitotenv.2017.08.254
- McGrath, M. J. , Olenius, T. , Ortega, I. K. , Loukonen, V. , Paasonen, P. , Kurtén, T. , … Vehkamäki, H. (2012). Atmospheric cluster dynamics code: A flexible method for solution of the birth-death equations. Atmospheric Chemistry and Physics , 12 , 2345–2355.10.5194/acp-12-2345-2012
- Monks, S. A. , Arnold, S. R. , Emmons, L. K. , Law, K. S. , Turquety, S. , Duncan, B. N. , … Ancellet, G. (2015). Multi-model study of chemical and physical controls on transport of anthropogenic and biomass burning pollution to the Arctic. Atmospheric Chemistry and Physics , 15 , 3575–3603. doi:10.5194/acp-15-3575-2015
- Nie, W. , Ding, A. J. , Wang, T. , Kerminen, V. M. , George, C. , Xue, L. K. , … Kulmala, M. (2014). Polluted dust promotes new particle formation and growth. Scientific Reports , 4 , 6634.
- Overland, J. E. , & Wang, M. (2013). When will the summer Arctic be nearly sea ice free? Geophysical Research Letters , 40 , 2097–2101. doi:10.1002/grl.50316
- Overland, J. E. , & Wang, M. (2016). Recent extreme arctic temperatures are due to a split polar vortex. Journal of Climate , 29 (15), 5609–5616. doi:10.1175/JCLI-D-16-0320.1
- Petäjä, T. , Järvi, L. , Kerminen, V. M. , Ding, A. J. , Sun, J. N. , Nie, W. , … Kulmala, M. (2016). Enhanced air pollution via aerosol boundary layer feedback in China. Scientific Reports , 6 , 310.10.1038/srep18998
- Petzold, A. , Thouret, V. , Gerbig, C. , Zahn, A. , Brenninkmeijer, C. A. M. , Gallagher, M. , … IAGOS Team . (2015). Global-scale atmosphere monitoring by in-service aircraft – current achievements and future prospects of the European Research Infrastructure IAGOS. Tellus B: Chemical and Physical Meteorology , 67 , 28452. doi:10.3402/tellusb.v67.28452
- Popp, T. , de Leeuw, G. , Bingen, C. , Brühl, C. , Capelle, V. , Chedin, A. , … Xue, Y. (2016). Development, production and evaluation of aerosol climate data records from european satellite observations (Aerosol_cci). Remote Sensing , 8 , 421. doi:10.3390/rs8050421
- Rissanen, M. P. , Kurtén, T. , Sipilä, M. , Thornton, J. A. , Kangasluoma, J. , Sarnela, N. , … Ehn, M. (2014). The formation of highly oxidized multifunctional products in the ozonolysis of cyclohexene. Journal of the American Chemical Society , 136 , 15596–15606.10.1021/ja507146s
- Roldin, P. , Liao, L. , Mogensen, D. , Dal Maso, M. , Rusanen, A. , Kerminen, V. M. , … Boy, M. (2015). Modelling the contribution of biogenic volatile organic compounds to new particle formation in the Jülich plant atmosphere chamber. Atmospheric Chemistry and Physics , 15 , 10777–10798.10.5194/acp-15-10777-2015
- Sayer, A. M. , Munchak, L. A. , Hsu, N. C. , Levy, R. C. , Bettenhausen, C. , & Jeong, M.-J. (2014). MODIS collection 6 aerosol products: Comparison between Aqua’s e-Deep blue, dark target, and “merged” data sets, and usage recommendations. Journal of Geophysical Research: Atmospheres , 119 , 13965–13989. doi:10.1002/2014JD022453
- Schellnhuber, J. , Cruzen, P. , Clark, W. , Claussen, M. , & Held, H. (Eds.). (2004). Earth system analysis for sustainability, dahlem workshop on earth system analysis for sustainability (454 pp). Berlin: MIT Press. 25–30 May 2003.
- Schutgens, N. A. J. , & Stier, P. (2014). A pathway analysis of global aerosol processes. Atmospheric Chemistry and Physics , 14 , 11657–11686.10.5194/acp-14-11657-2014
- Sipila, M. , Berndt, T. , Petaja, T. , Brus, D. , Vanhanen, J. , Stratmann, F. , … Kulmala, M. (2010). The role of sulfuric acid in atmospheric nucleation. Science , 327 , 1243–1246.10.1126/science.1180315
- Smith, L. C. (2010). The World in 2050: Four forces shaping civilization’s Northern future . Clays, Bungay, Suffolk: Brockman.
- Stavrakou, T. , Müller, J. F. , Bauwens, M. , De Smedt, I. , Van Roozendael, M. , Guenther, A. , … Xia, X. (2014). Isoprene emissions over Asia 1979–2012: Impact of climate and land-use changes. Atmospheric Chemistry and Physics , 14 , 4587–4605. doi:10.5194/acp-14-4587-2014
- Sundström, A.-M. , Nikandrova, A. , Atlaskina, K. , Nieminen, T. , Vakkari, V. , Laakso, L. , … Kulmala, M. (2015). Characterization of satellite-based proxies for estimating nucleation mode particles over South Africa. Atmospheric Chemistry and Physics , 15 , 4983–4996. doi:10.5194/acp-15-4983-2015
- van der Mijling, B. A. R. J , Ding, J. , Koukouli, M. E. , Liu, F. , Li, Q. , … Theys, N. (2017). Cleaning up the air: Effectiveness of air quality policy for SO2 and NO x emissions in China. Atmospheric Chemistry and Physics , 17 , 1775–1789. doi:10.5194/acp-17-1775-2017
- Vanhanen, J. , Mikkilä, J. , Lehtipalo, K. , Sipilä, M. , Manninen, H. E. , Siivola, E. , … Kulmala, M. (2011). Particle size magnifier for nano-CN detection. Aerosol Science and Technology , 45 , 533–542.10.1080/02786826.2010.547889
- Wang, T. , Wei, X. L. , Ding, A. J. , Poon, C. N. , Lam, K. S. , Li, Y. S. , Chan, L. Y. , & Anson, M. (2009). Increasing surface ozone concentrations in the background atmosphere of Southern China, 1994–2007. Atmos. Chem. Phys. , 9 , 6217–6227. doi:10.5194/acp-9-6217-2009
- Xiao, S. , Wang, M. Y. , Yao, L. , Kulmala, M. , Zhou, B. , Yang, X. , … Wang, L. (2015). Strong atmospheric new particle formation in winter in urban Shanghai, China. Atmospheric Chemistry and Physics , 15 , 1769–1781.10.5194/acp-15-1769-2015
- Xue, Y. , Juang, H.-M. , Li, W.-P. , Prince, S. , DeFries, R. , Jiao, Y. , & Vasic, R. (2004). Role of land surface processes in monsoon development: East Asia and West Africa. Journal of Geophysical Research: Atmospheres , 109 (D3). doi:10.1029/2003JD003556
- Xue, Y. , & Dirmeyer, P. A. (2015). Land-atmosphere interactions in monsoon regimes and future prospects for enhancing prediction. CLIVAR Exchanges , 66 (1), 19.
- Xue, Y. , He, X. , de Leeuw, G. , Mei, L. , Che, Y. , Rippin, W. , … Hu, Y. (2017). Long-time series aerosol optical depth retrieval from AVHRR data over land in North China and Central Europe. Remote Sensing of Environment , 198 , 471–489.10.1016/j.rse.2017.06.036
- Ye, Q. , Zong, J. , Tian, L. , Gogley, J. G. , Song, C. , & Guo, W. (2016). Three epochs of data on glacier changes on the Tibetan plateau derived from LANDSAT imagery, from the 1970s to 2013. In H. K. Lappalainen , A. Borisova , D. Liang , J. Enroth , and M. Kulmala (Eds.), Proceedings of the 2nd Pan-Eurasian Experiment (PEEX) Conference and the 6th PEEX Meeting, Report Series in Aerosol Science (Vol. 180, p. 578).
- Yubao, Q. , Savela, H. , Key, J. R. , Menenti, M. , Vitale, V. , Cheng, X. , … the GEOCRI Group21 . (2016). Statement for the 2016 Arctic Observing Summit, Statement of GEO . Retrieved from www.arcticobservingsummit.org/sites/arcticobservingsummit.org/files/Qiu-REVISEDRC–GEOCRI-Statement-20160201-revised.pdf
- Zhang, S. , & Yuan, J. , & Zhang J. (2016). Analysis on correlation between NPP of different vegetation type and air temperature, precipitation LAI and NDVI in East Asian monsoon region – a case in Hebei province. In H. K. Lappalainen , A. Borisova , D. Liang , J. Enroth , and M. Kulmala (Eds.), Proceedings of the 2nd Pan-Eurasian Experiment (PEEX) Conference and the 6th PEEX Meeting Report Series in Aerosol Science (Vol. 180, pp. 559–602).
- Zhang, Y. , Bocquet, M. , Mallet, V. , Seigneur, C. , & Baklanov, A. (2012). Real-time air quality forecasting, part II: State of the science, current research needs, and future prospects. Atmospheric Environment , 60 , 656–676.10.1016/j.atmosenv.2012.02.041
- Wang, J. , Zhao, B. , Wang, S. , Yang, F. , Xing, J. , Morawska, L. , … Hao, J. (2017). Particulate matter pollution over China and the effects of control policies. Science of The Total Environment , 584–585 , 426–447.10.1016/j.scitotenv.2017.01.027
- Webster, P. J. , Magaña, V. O. , Palmer, T. N. , Shukla, J. , Tomas, R. A. , Yanai, M. , & Yasunari, T. (1998). Monsoons: Processes, predictability, and the prospects for prediction. Journal of Geophysical Research: Oceans , 103 , 14451–14510. doi:10.1029/97JC02719
- Zilitinkevich, S. S. , Hunt, J. C. R. , Grachev, A. A. , Esau, I. N. , Lalas, D. P. , Akylas, E. , … Joffre, S. M. (2006). The influence of large convective eddies on the surface layer turbulence. Quarterly Journal of the Royal Meteorological Society , 132 , 1423–1456.10.1256/qj.05.79
- Zilitinkevich, S. (2012). The height of the atmospheric planetary boundary layer: State of the art and ew development – Chapter 13. In H.J.S. Fernando , Z. Klaić , & J.L. McKulley (Eds.), National security and human health implications of climate change. NATO Science for Peace and Security Series – C: Environmental Security (pp. 147–161). Springer. ISBN 978-94-007-2429-7.
- Zilitinkevich, S. , Esau, I. , & Baklanov, A. (2007). Further comments on the equilibrium height of neutral and stable planetary boundary layers. Quarterly Journal of the Royal Meteorological Society , 133 , 265–271.10.1002/(ISSN)1477-870X
- Zilitinkevich, S. , Kulmala, M. , Esau, I. , & Baklanov, A. (2015). Megacities – refining models to personal environment. WMO Bulletin , 64 (1), 20–22.
- Zilitinkevich, S. S. , Elperin, T. , Kleeorin, N. , Rogachevskii, I. , & Esau, I. N. (2013). A Hierarchy of energy- and flux-budget (EFB) turbulence closure models for stably-stratified geophysical flows. Boundary-Layer Meteorology , 146 , 341–373.10.1007/s10546-012-9768-8