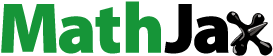
ABSTRACT
Among the environmental threats, the intensification of natural hazards, such as soil erosion may threaten the integrity and value of cultural heritage sites. In this framework, the present study’s main objective was to identify archaeological sites susceptible by soil erosion, taking the case study of Chania prefecture in Crete Island. Remotely sensed and other available geospatial datasets were analyzed in a GIS-based empirical model, namely Unit Stream Power Erosion and Deposition (USPED), to estimate the average annual soil loss and deposition rates due to water-induced erosion in the study area. The resultant erosion map was then intersected with the locations and surrounding zones of the known archaeological sites for identifying the sites and the portions of their vicinity being at risk. The results revealed that Chania prefecture and its cultural heritage are significantly affected by both soil loss and deposition processes. Between the two processes, soil loss was found to be more intensive, influencing a larger part of the prefecture (especially to the west) as well as a higher amount of archaeological sites. The extreme and high soil loss classes were also detected to cover the most considerable portion of the sites’ surrounding area. The identification of the archaeological sites being most exposed to soil erosion hazard can constitute a basis for cultural heritage managers in order to take preventive preservation measures and develop specific risk mitigation strategies.
1. Introduction
According to UNESCO World Heritage Centre (Citation2017), cultural heritage consists of all those monuments, groups of buildings, and sites with an outstanding value from a historical, artistic and scientific point of view. It plays a significant role in the socio-economic growth of a country, reflecting at the same time the sense of belonging and individuals’ cultural identity (Spennemann, Citation1999). Given the very large extent of some properties (especially in the case of the world heritage sites) or the level of detail needed for their assessment, the geospatial technologies of Geographic Information System (GIS) and Remote Sensing (RS) constitute particularly useful tools in the field of cultural heritage research by a spatial perspective. GIS can be defined as a computer-based system that is used to input, store, retrieve, process, analyze, and visualize spatial information in order to support decision-making (Awange & Kiema, Citation2019). RS is the practice of deriving information about an object from measurements made at a distance from it, based on the radiation in the electromagnetic spectrum reflected or emitted from the Earth’s surface (Campbell & Wynne, Citation2011). The integration of GIS and RS provides the most effective methodology for detecting, monitoring, and documenting cultural heritage sites and monuments (Negula, Sofronie, Virsta, & Badea, Citation2015). Studies conducted for detecting looted archeological areas or buried archeological remains (Agapiou, Lysandrou, & Hadjimitsis, Citation2017; Giardino, Citation2011; Lasaponara, Leucci, Masini, & Persico, Citation2014), monitoring cultural heritage sites (Oppio et al., Citation2015; Pastonchi et al., Citation2018) and identifying areas with potential archaeological evidence (Lysandrou & Agapiou, Citation2016) have demonstrated the great benefits of this integration.
Nowadays, cultural heritage is facing several anthropogenic and environmental threats, such as population growth and urban expansion from one side and the occurrence of severe natural hazards from the other side. The protection and preservation of cultural heritage sites from these threats, by incorporating sustainable development and resilience initiatives, constitutes a significant concern worldwide over the last decades. The link between threats and built cultural heritage at risk has been implemented in numerous projects on national and European levels, often resulting in comprehensive risk mapping and threat monitoring (Accardo, Giani, & Giovagnoli, Citation2003; Della Torre, Citation2020).
In the last decade, the climate change has increased the intensity and the frequency of natural hazards. Cultural heritage monuments and sites, as an integral part of the human-built environment, are completely exposed to natural hazards’ adverse actions (Ravankhah et al., Citation2019). Despite their similarity in terms of their negative impact, these actions differ by type (external or internal) and duration. The disruption or the damage of the monuments represent the external effects, whereas the internal effects are signified by the increased sensitivity of structural materials to the environment’s conditions (Hapciuc et al., Citation2016). Moreover, natural hazards like salinity and soil erosion may have long term and persistent effects indicated by the gradual decay of a heritage monument. In contrast, flooding or seismic events are more likely to have short term and instant effects in the form of direct catastrophic damages. Therefore, the assessment of a natural hazard by identifying its spatial extent and intensity and evaluating its expected effects is considered as an essential component of cultural heritage management and planning. The integration of GIS and RS technologies has been widely used to analyze, map, and retrieve reliable information related to the natural hazards (Agapiou et al., Citation2015). Special attention has been given to the assessment of several natural hazards at the locations and vicinity of cultural heritage sites (Cuca, Citation2020; Liu, Xu, Chen, Chen, & Zhang, Citation2019; Lombardo, Tanyas, & Nicu, Citation2020; Ortiz, Ortiz, Martín, & Vázquez, Citation2016; Ravankhah et al., Citation2019; Salamon, Netzer-Cohen, Cohen, & Zilberman, Citation2018; Valagussa et al., Citation2020).
Water-induced soil erosion constitutes one of the major natural hazards worldwide. It involves the detachment, transport and sedimentation of soil particles from a given initial area to a new depositional area due to rainfall and water surface runoff (Gelagay & Minale, Citation2016). The detachment and sedimentation of soil particles, expressed by soil loss and soil deposition, respectively, contribute significantly to the ecological deterioration of the environment and the degradation of soil fertility (thus, agricultural productivity reduction). These severe environmental and economic effects of water-induced soil erosion have displayed its assessment as a critical need.
A variety of soil erosion assessment models have become available over recent years with empirical, conceptual, and physical models composing the three main groups. However, in terms of computational complexity and data requirements, the implementation of GIS-based empirical models (Barmaki, Pazira, & Hedayat, Citation2011; Karydas & Panagos, Citation2018) has been widely preferred against the more complicated conceptual and physical models (Choi, Arnhold, Huwe, & Reineking, Citation2017; Pandey, Himanshu, Mishra, & Singh, Citation2016). In general, the empirical models have been applied at different regions and spatial scales by analyzing data that represent soil erosion-influencing conditions like climate, topography, soil composition, surface biophysical coverage, and human activities (Elaloui, Marrakchi, Fekri, Maimouni, & Aradi, Citation2017; Halecki, Kruk, & Ryczek, Citation2018; Kourgialas, Koubouris, Karatzas, & Metzidakis, Citation2016; Panagos et al., Citation2015a; Polykretis, Alexakis, Grillakis, & Manoudakis, Citation2020).
In the framework of cultural heritage management, the soil loss and soil deposition seem to affect not only the visible-standing monuments but also the unexcavated subsurface remains (Agapiou, Lysandrou, & Hadjimitsis, Citation2020). When developing management strategies to ensure the long-term survival of threatened monuments and archaeological remains, the understanding and determination of rates, timing and drivers of soil erosion on cultural heritage sites are fundamental (Kincey, Gerrard, & Warburton, Citation2017). Concerning the relative literature, a limited number of studies have focused on empirical model-based soil erosion assessment for cultural heritage sites. For instance, Alexakis and Sarris (Citation2010) assessed separately different environmental (including soil erosion) and human threats, and they finally combined them to produce an integrated risk assessment result for prehistoric and historic archaeological sites of western Crete Island (Greece). Similar work was carried out for a district of Cyprus by Agapiou, Lysandrou, Themistocleous, and Hadjimitsis (Citation2016), based on homogeneous clusters of cultural heritage monuments. Furthermore, Cuca and Agapiou (Citation2018) quantified soil loss and its association with land-use changes for two study areas in Cyprus: an UNESCO protected archaeological site and a section of an ancient Hellenistic-Roman road network.
By using empirical models, the above studies considered the regions as homogeneous planar landscapes not being affected by the complex topography, especially regarding the soil flow convergence and divergence. Moreover, they provided overall estimations exclusively for soil loss disregarding the respective soil deposition that may occur. In contrast to them, this study determined the spatial extent and intensity of water-induced erosion by estimating both soil loss and deposition rates, and applied them as a risk assessment index for the archaeological sites of Chania prefecture in the island of Crete. A GIS-based empirical model, the Unit Stream Power Erosion and Deposition (USPED), was implemented for this purpose. Remotely sensed and other available spatial datasets were incorporated in the model to produce the soil erosion map, which was then intersected with the locations and surrounding zones of the study area’s archaeological sites for identifying the sites and the portions of their vicinity being at risk.
2. Study area
Located in the southern part of Greece, Crete is the largest Greek and fifth largest Mediterranean island covering a total extent of over 8,200 km2 (). Nowadays, Crete is the most populated Greek island with over 600,000 inhabitants (ELSTAT – Hellenic Statistical Authority, Citation2011). The majority of them is concentrated in areas around the urban administrative prefectures of Chania, Rethymno, Heraklion, and Lasithi. Evidence for modern human presence dates to 10,000–12,000 years ago, and it was not until the Neolithic period (8,500 to 4,900 BP) when the first signs of advanced agriculture appeared in the island opening the way for the subsequent emergence of the Minoan civilization during the Bronze Age (5,600 to 3,000 BP) (Glowacki & Vogeikoff-Brogan, Citation2011). The continuous habitation on the island was enriched by influences from other cultures of the Eastern Mediterranean, highlighting the historical value of the island. This is reflected in the presence of a significant number of cultural heritage monuments and sites.
Figure 1. (a) The location of Chania prefecture; (b) Distribution of the most significant known archeological sites of Chania prefecture.
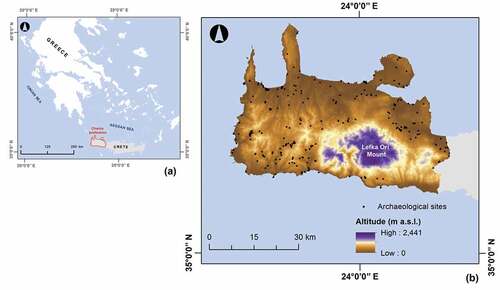
The prefecture of Chania at the western part of Crete was selected for further evaluation in this study (). It covers about 2,300 km2 containing the 25% (over 150,000 inhabitants) of the island’s total population. Its climate is sub-humid Mediterranean with humid cold winters and rather warm summers (Tsanis, Koutroulis, Daliakopoulos, & Jacod, Citation2011), while its topography follows the typical Cretan landscape mainly composed of mountainous ridges (reaching an altitude of 2,441 m above sea level) and various landforms (Argyriou, Teeuw, & Sarris, Citation2017). The study area concentrates a large number of archaeological sites, spanning among different historical periods from the Minoan, to Classical, Roman, Arabic, Venetian, and Ottoman periods (Panou, Ragia, Dimelli, & Mania, Citation2018). These sites are visible mainly in urban and rural areas of low and moderate altitudes, spreading both near and far from the coastline (). Their preservation status has been steadily compromised by adverse climate conditions and the occurrence of severe natural hazards, as well as the rapid expansion of the modern urban and touristic activities over the last few decades.
3. Materials and methods
3.1. Data
For the study’s needs, a geospatial database was designed and developed in a GIS environment including datasets related to the erosion-influencing factors and the known archaeological sites of the region.
Spatial datasets representing the factors that affect soil erosion constitute critical information for any empirical modelling. In this study, various relative datasets were analyzed in the applied model, including rainfall, satellite imagery, soil, topography, and land use/cover data. The spatial scale of the analysis and the data availability comprised the main factors for their selection. Particularly, a time series of daily rainfall measurements covering a period between 1981 and 2019 was obtained from two independent networks of meteorological stations in Crete. Openly available, cloud-free satellite images of 30 m spatial resolution, taken from Landsat-8 operational land imager (OLI) in May 2019, were also collected. Two different soil datasets were used to acquire the study area’s soil types and their relative properties, respectively. A Shuttle Radar Topographic Mission (SRTM) Digital Elevation Model (DEM) of 30 m spatial resolution and a set of land use/cover (LUCAS) observations were exploited to indicate the terrain elevation and the agricultural practices, respectively ().
Table 1. Summary of spatial datasets used in this study.
The spatial dataset of archaeological sites was derived from the “Digital Documentation and Management Inventory” of “Digital Crete: Mediterranean Cultural Itineraries” project. The project was implemented under the framework of the Greek Operational Program Information Society and funded by the 3rd European Community Support Framework, resulting to the creation of a cultural information system consisted of digitized documentation and information regarding the cultural heritage of the island of Crete, from prehistory to the modern periods (Sarris et al., Citation2009). Based on the spatial information of the Inventory, the locations of 263 archaeological sites were recognized ().
3.2. USPED modelling
The Unit Stream Power Erosion and Deposition (USPED) is a fixed model, which estimates soil loss and deposition rates considering a steady overland water flow with uniform rainfall-excess conditions (Garcia Rodriguez & Gimenez Suarez, Citation2012). With a theoretical background initially defined by Moore and Burch (Citation1986) and then improved by Mitasova, Hofierka, Zlocha, and Iverson (Citation1996), USPED assumes that soil erosion depends on the detachment capacity and the sediment transport capacity of overland water flow. Particularly, it is based on the following two assumptions (Mitasova et al., Citation1996):
The water flow can transport a limited amount of detached soil sediment determined by its full transport capacity. Hence, the sediment flow rate, qs, can be approximated by the sediment transport capacity, Tc, as below:
where r is a location or GIS grid cell with (x, y) coordinates, Kt is the transportability coefficient, q is the water flow rate (m3 m−1 s−1), β is the slope gradient (degrees), and m, n are constants depending on the type of erosion (set as 1.6 and 1.3 respectively for rill erosion, and as 1 for sheet erosion). Terms of this equation can be also expressed by the incorporation of five erosion-influencing factors, including four natural factors such as rainfall erosivity, soil erodibility, cover management and topographic component for sediment transport, and one anthropogenic factor such as support practice:
where K is the soil erodibility factor (t ha h ha−1 MJ−1 mm−1), C is the cover management factor (dimensionless), P is the support practice factor (dimensionless), R is the rainfall erosivity factor (MJ mm ha h−1), and A is the upslope contributing area per unit contour width (m2 m−1). According to the properties (e.g. texture, structure, permeability) of a specified soil type, the K-factor represents its inherent susceptibility to erosion (Pal & Chakrabortty, Citation2019). The higher its value, the more susceptible the soil type. The C and P-factors reflect the impact of vegetation coverage and agricultural practices (e.g., contour farming, tillage, terracing, stone walls, grass margins, etc.), respectively, on the erosion (Maury, Gholkar, Jadhav, & Rane, Citation2019; Phinzi & Ngetar, Citation2019). The values of both factors range from 0 to 1 with those close to 0 being attributed to areas with high vegetation coverage and presence of support practices respectively, whereas those close to 1 to bare lands and areas with none practice respectively. The R-factor quantifies the rate of runoff that is likely to be associated with the rainfall amount and intensity (Sujatha & Sridhar, Citation2018). The higher its value, the greater the propensity of soil to erode. Moreover, the factor of topographic component for sediment transport, LST, can be defined as:
Applying to complex topographies, LST-factor (dimensionless) reflects the flow convergence (Leh, Bajwa, & Chaubey, Citation2013). Therefore, by taking into account the EquationEquations (2)(2)
(2) , (Equation3
(3)
(3) ) and (Equation4
(4)
(4) ), the sediment transport capacity can also be derived from:
(b) The soil loss/deposition rate, ds, can be computed as the change in sediment flow rate expressed by a divergence in sediment flow:
where s0 is the unit vector in the steepest slope direction, and α is the slope aspect (degrees). The resultant value of ds is both negative and positive indicating soil loss and soil deposition, respectively. For a GIS-based implementation, the terms of EquationEquation (6)(6)
(6) can be rewritten using the following relationships between partial derivatives, and slope gradient and aspect:
where x, y, and z represent a 3-dimensional space.
4. Soil erosion assessment
In order to assess soil erosion in the present study, the USPED model was applied within the GIS environment. The first step for the implementation of the model was the preparation of the previously described erosion-influencing factors by exploiting the acquired spatial datasets.
The different soil types of Chania prefecture were linked to their relative texture properties. The K-factor values for the soil types were then estimated by using these properties in the following approach developed by Williams and Renard (Citation1983):
where SAN is the sand content (%), SIL is the silt content (%), CLA is the clay content (%), C is the organic carbon content (%), and SN = 1 – (SAN/100).
For the creation of C-factor, the pre-processing of Landsat-8 satellite images was initially conducted through the application of atmospheric and radiometric corrections based on dark object subtraction (Song, Woodcock, Seto, Lenney, & Macomber, Citation2001) and radiometric rescaling coefficients (Chander, Markham, & Helder, Citation2009), respectively. A random sample of 246 areas was then collected and labeled as specific land cover types by visual interpretation. Using this sample, a supervised classification algorithm like Mahalanobis distance (Srivastava & Rao, Citation2016) was performed to produce a detailed land cover map. C-factor values were assigned to the relative land cover types of the resultant map, according to Sujatha and Sridhar (Citation2018).
Three types of support practices such as contour farming, stone walls, and grass margins were considered for calculating the P-factor as proposed by Panagos et al. (Citation2015b):
where Pcf, Psw, and Pgm are the P-factor values for contour farming, stone walls, and grass margins, respectively. The Pcf values were derived from the SRTM DEM-based derivative of slope gradient (Morgan, Citation2005), whereas the Psw and Pgm from the densities of relative LUCAS observation point data (Panagos et al., Citation2015b).
The R-factor was produced by analyzing the daily measurement rainfall data in the regression-based approach proposed by Grillakis, Polykretis, and Alexakis (Citation2020):
where R10 is the total rainfall within a month (mm) assuming the days with rainfall ≥ 10 mm, D10 is the number of days with rainfall ≥ 10 mm, n is the number of days covered by the rainfall data, k is the individual erosive events of each month j, and mj is the total number of erosive events of this month. Since the R-factor values from EquationEquation (11)(11)
(11) referred to a meteorological station level, they were then interpolated to a prefecture level.
The LST-factor was generated by incorporating the appropriate SRTM DEM-based hydro-morphological derivatives (slope gradient and flow accumulation) in EquationEquation (4)(4)
(4) . The m and n constants were set as 1 for examining sheet erosion.
All the above factors were organized in GIS raster grids with 30 m spatial resolution (). After the preparation of factors, the sediment transport capacity was estimated using EquationEquation (5)(5)
(5) . Considering this output and the EquationEquations (6)
(6)
(6) , (Equation7
(7)
(7) ) and (Equation8
(8)
(8) ), an erosion map was produced presenting the average annual soil loss and deposition rates in Chania prefecture. A classification of the estimated rates was then executed in a manual way according to their range. According to , although areas affected by high to extreme soil loss and deposition are distributed across the entire Chania prefecture, some pronounced concentrations of them can be mainly detected in its western and north-western parts as well as on its dominant massif, the Lefka Ori Mount. As it is shown in , these areas cover about 29% and 16% of the prefecture, respectively. About 29% of prefecture is characterized by low loss or deposition classes, whereas about 18% is stable.
Figure 2. Erosion-influencing factors analyzed in USPED model (a) K-factor (b) C-factor (c) P-factor (d) R-factor (e) LST-factor.
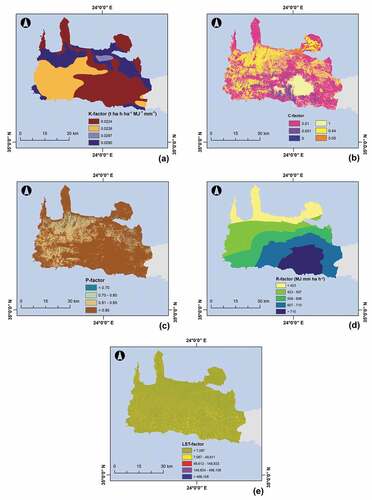
Figure 4. Correlation of the soil loss and deposition classes with coverage area percentages for Chania prefecture.
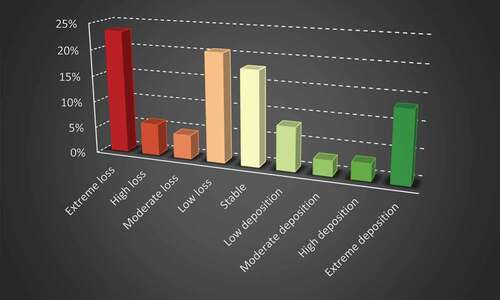
Given that the main objective of this study was to assess the exposure of cultural heritage sites to soil erosion, the intersection of the produced erosion map with the known locations of archaeological sites in the study area was carried out. However, the risk level for a particular site cannot be judged only for its particular location; instead, it should be based on the existence or not of risk levels within a specific spatial range around the location (Liu et al., Citation2019). Hence, a buffer zone of 500 m around each site was also examined. The results of these additional steps are shown in and . Based on this calculation, approximately 22% of the archaeological sites are located completely in areas of extreme soil loss. The particular sites are mainly located to the west and north-east of Chania prefecture. Approximately 15% of the sites are exposed to extreme soil deposition. These are mainly concentrated in the south-western part of the prefecture. With a percentage of 20% and 14%, significant amounts of sites are also detected in low loss and stable, respectively, locations. Statistics do not change significantly if we take into account the vicinity around the archaeological sites: 23% and 15% of the area enclosed in buffer zones belong to the classes of extreme soil loss and deposition, respectively. A portion accounting for approximately 17% of this area seems to be affected by low soil loss. A percentage of about 15% is detected within the stable soil erosion class.
5. Discussion and conclusions
The idea of preventive preservation and monitoring is promoted as an efficient way to protect archaeological sites and monuments of significant historical and cultural value from anthropogenic and environmental threats. Among the various environmental threats, water-induced soil erosion constitutes one of the major threats that has endangered a number of archaeological sites in the island of Crete and elsewhere in the Mediterranean region. Since soil erosion can directly influence a site by detaching soil sediment, transporting and deposing on it, the acquisition of knowledge about these two processes can be considered as an essential part for the cultural heritage management. Thus, the present study identified the sites of Chania prefecture, which are exposed to both soil loss and deposition processes. Through the identification of the threatened sites, the main purpose of the study can be defined as a contribution to managers and decision-makers on cultural heritage conservation in order to firstly be aware of those sites that need greater attention and protection, and then take focused preventive measures and develop specific risk mitigation strategies.
The applied methodology was mainly based on the assessment of the spatial extent and intensity of soil loss and deposition. The USPED empirical model was implemented under the integration of a set of erosion-influencing factors. Due to its spatial flexibility, USPED allows modelling the physical processes of erosion in a GIS environment. The model can provide an estimation of the average annual soil loss and deposition rates, which can be correlated to the topographic location of the archaeological sites. In this line, the present study comprises one of the first attempts to explore the impact of both erosion-related processes on archaeological sites.
Chania prefecture was found to be significantly affected by both soil loss and deposition. In fact, by increasing the runoff velocity, the steeper slopes of its mountainous terrain favour the detachment of sediments (soil loss). These sediments are then accumulated (soil deposition) close to or along the stream network because of low transport capacities. However, between the two processes, soil loss can be characterized as more intensive by influencing a larger part of the prefecture, especially towards the west. This can be also justified by the fact that the spatial variability of rainfall in Crete manifests a decrease from west to east (Grillakis et al., Citation2020) and its correlation with higher erosion levels to the west (Polykretis et al., Citation2020) confirm this finding.
From the perspective of cultural heritage, this study revealed that soil erosion could highly contribute to the degradation of the archeological sites of Chania prefecture, with both soil loss and deposition processes influencing them. In particular, a significant amount of archaeological sites (in total, 37%) are established in areas of extreme soil loss and deposition. The majority of these sites are mainly located in the western part of Chania prefecture. Furthermore, a similar tendency was shown in the vicinity of the sites, with a notable portion of their buffer zone area (in total, 38%) belonging to the relative erosion classes.
The present study presents a cost-efficient methodology to determine the soil erosion hazard of a given region and identify the cultural heritage sites being exposed to it. Despite the assumptions and limitations of this methodology, such as the simplification of localized reality and the inherent uncertainty of model, its results can constitute the basis for a better understanding of the sensitivity conditions of the archaeological sites. This understanding can be explained from the assumption that the soil erosion may cause similar resilience problems to sites with different structural characteristics (e.g. the type of basement, the construction method and materials). The particular methodology can be incorporated into a more integrated risk assessment approach, and especially considering vulnerability assessment, to adequately analyze the structural sensitivity of architectural monuments to soil erosion. It is evident that the general methodology can be extended to diverse geographical regions as well.
By using available geospatial, the proposed methodology can be easily applied in other regions of interest to the extent of assessing the hazard for different monuments and sites. This is also supported by the full and freely open access (FFO) policy of the Copernicus Programme, like the Sentinel satellite sensors. Multispectral imagery data characterized by a significant level of detail (spatial resolution) and frequent revisit (temporal resolution) are derived from these sensors, speeding up the scientific work such as survey, mapping, documentation, exploitation and monitoring of cultural heritage at different spatial scales. Thus, there is no doubt that the geospatial big data, nowadays available from heterogeneous data sources (sensor networks, digital libraries, web data service, social networks, etc.), provide new opportunities for the scientific community to improve the research and decision support applications with unprecedented value for digital cultural heritage, and engage citizens into the human past and its contemporary legacies. The current study also provides experimental evidence that the integration of GIS with RS technologies can be very useful in constructing risk maps for cultural heritage preservation.
Data availability statement
The data that support the findings of this study are available from the corresponding author, C.P., upon reasonable request.
Disclosure statement
No potential conflict of interest was reported by the author(s).
Additional information
Funding
Notes on contributors
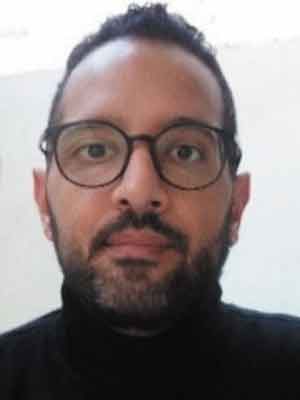
Christos Polykretis
Dr Christos Polykretis is Geographer (BSc) holding master (MSc) in “Management and Analysis of Geographic Information (Geoinformatics)” and PhD in Geoinformatics for environmental modeling. Since 2019, he is post-doctoral researcher at the Laboratory of Geophysical - Satellite Remote Sensing and Archaeo-environment (GeoSat ReSeArch Lab), Institute for Mediterranean Studies (IMS), Foundation for Research and Technology - Hellas (FORTH). His research interests focus on the application of Geoinformatics technologies (geographic information systems, remote sensing, etc.) in the study and analysis of several phenomena/problems (environmental, social, etc.). His specialization is mainly located in the areas of geospatial/satellite imagery data processing and spatial analysis. He has provided supportive teaching on relevant undergraduate and postgraduate university courses, and has also lectured on various training and lifelong learning programs. His research work includes publications in both national and international scientific journals and conference proceedings, as well as participations as external collaborator in several research projects. Furthermore, his previous professional experience includes jobs as geospatial data analyst in both public and private sectors.
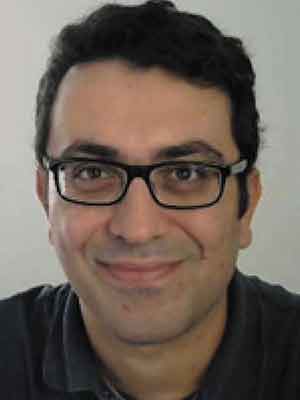
Dimitrios D. Alexakis
Dr Dimitrios D. Alexakis is Assistant Researcher at the Institute for Mediterranean Studies (IMS), Foundation for Research and Technology Hellas (FORTH). During his PhD studies, he received a Marie Curie Fellowship and a scholarship from Greek State Scholarship Foundation. After the completion of his PhD studies, he worked as postdoctoral researcher and adjunct Professor at Cyprus University of Technology (2011-2014) and Technical University of Crete (2014-2018). His scientific interests involve applications of Remote Sensing (Satellite, UAV, Field Spectroscopy, SAR, Aerial) and GIS in the fields of Geomorphology, Landscape Ecology, Natural Hazards, Landscape archaeology, Hydrology, Spatial planning and Environmental monitoring. He has published 44 peer-reviewed papers, 6 book chapters and more than 85 papers in conference proceedings. He has organized and participated as instructor in international workshops (RESTECH, Marie Curie, etc.) and has acted as member of the scientific/organizing committee of international conferences. Dr Alexakis is member of the editorial board of “Remote Sensing MDPI” and “Open Geosciences“ journals. He has been involved either as coordinator or partner in more than 40 International and National funded research projects (Horizon 2020, Life+, ERASMUS+ 2020, FP7 Research, INTERREG III, ARISTEIA, ELIDEK, etc.).
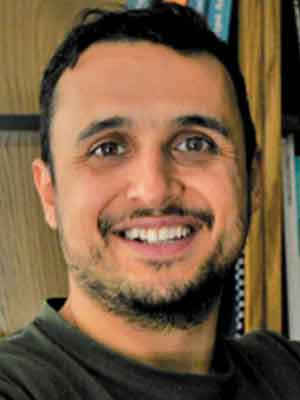
Manolis G. Grillakis
Dr Manolis G. Grillakis holds BSc in Environmental Engineering (2006), and a MSc (2007) in the field of Remote Sensing for Hydrological Applications, from the Technical University of Crete (TUC). Throughout his studies, he has received several awards and scholarships. After the acquisition of his PhD (2014) in Hydrology and Climate Change, he was a post-doctoral researcher at the School of Environmental Engineering, in TUC. In 2020, he joined the Institute for Mediterranean Studies (IMS) of Foundation for Research and Technology - Hellas (FORTH) where he is currently post-doctoral researcher in the Laboratory of Geophysical - Satellite Remote Sensing and Archaeo-environment (GeoSat ReSeArch Lab). His research interests are specialized on water resources management, climate change impact assessment (floods, soil moisture, drought, etc.), climate data bias correction, hydrological process and earth surface simulation modeling (GHMs - LSMs). He has extensive teaching experience as lecturer in the fields of Hydrology/Hydraulics and Environmental Engineering. Dr Grillakis has been involved in various research projects at a European (H2020, FP7, ETC.) and national level (HFRI, IKY, etc.). He has published a considerable number of papers in high impact peer-reviewed scientific journals, as well as in international and national (peer-reviewed) conference proceedings.
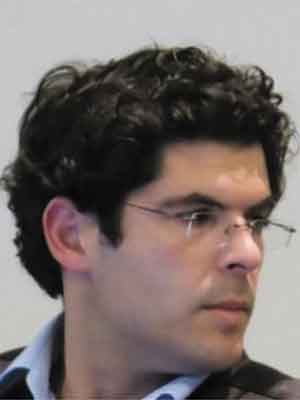
Athos Agapiou
Dr Athos Agapiou is an Assistance Professor (since 07/2021) at the Cyprus University of Technology. He obtained his Diploma from the School of Rural and Surveying Engineering at the National Technical University of Athens (2005), while two years later, he obtained a MSc in the field of Geoinformatics from the same university. Dr Agapiou holds a M.A. from the Department of History and Archaeology of the University of Cyprus (2010). In 2013, he received his PhD. Throughout his studies, he has received several awards and scholarships. During the last years, he has been involved in various research projects at a European (H2020), regional (JPI-CH; Interreg) and local level (Research Promotion Foundation Cyprus). For these research activities, he received the Cyprus Research Awards Competition, “Young Researcher 2018”. Currently, he is acting as the Chair of the CAA-Gr (National Chapter of the ‘Computer Applications and Quantitative Methods in Archaeology’), National Delegate of Cyprus for Cluster 4 – “Digital, Industry and Space” of the Horizon Europe EU Framework Programme for Research and Innovation, as well as reviewer at NASA Postdoctoral Program (NPP). He has authored numerous publications in scientific journals and conferences.
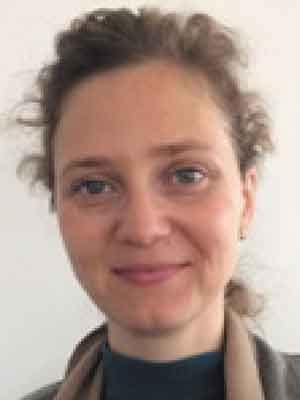
Branka Cuca
Dr Branka Cuca has received her bachelor degree in Architecture at the Politecnico di Milano (POLIMI) in 2003, where she has obtained a master’s degree in Architecture (2006). In March 2010, she has earned a title of PhD in Geomatics and Infrastructures. From 2013 to 2016, she has collaborated with Remote Sensing Lab at the Cyprus University of Technology (CUT). Since January 2017, she is an Assistant Professor in Topography and Cartography (Ricercatore legge 240/10 - t.det.) at the Department of Architecture, Built environment and Construction engineering (ABC) of POLIMI. Her scientific interests involve applications on Geoinformatics (Remote Sensing and GIS), Photogrammetry, Cultural Heritage, Surveying and Mapping. Since 2013, she acts as a co-chair of the Working Group Earth Observation/Copernicus of the European network NEREUS (Network of European Regions Using Space Technologies). Since 2009 until 2013, Dr Cuca was conduction coordination work at the Delegation of Lombardy Region in Brussels, supporting 12 research groups during project proposals preparation under European research and innovation programs (FP7, CIP, IEE, LIFE +, INTERREG, ESPON etc.) and promoting the interests of these research groups. He has authored numerous publications in peer-reviewed scientific journals and conference proceedings.
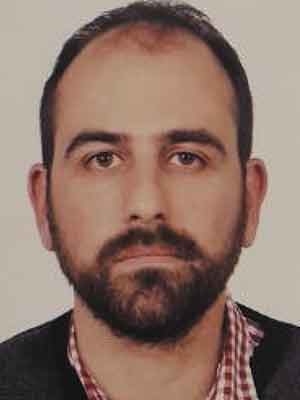
Nikos Papadopoulos
Dr Nikos Papadopoulos holds BSc in Geology (2001) and PhD (2007) in Applied Geophysics from the University of Thessaloniki (Greece). He was a post-doctoral researcher in the Korea Institute of Geoscience and Mineral Resources, KIGAM (2008) and Visiting Fellow Researcher in University of Arkansas, USA (2013). He joined the Foundation for Research and Technology, Hellas (FORTH) in 2009 where he is currently Associate Researcher of Applied Geophysics in Cultural Heritage in the Laboratory of Geophysical-Satellite Remote Sensing & Archaeo-environment (GeoSat ReSeArch Lab). His research interests include the numerical modeling and inversion of resistivity tomographic data, the implementation of diverse geophysical methods for near surface archaeological, environmental, urban, shallow marine applications and the employment of Geoinformation technologies in cultural and natural resources management. He is Associate Editor of Journal of Archaeological Prospection and Near Surface Geophysics and Topic/Guest Editor in Remote Sensing.
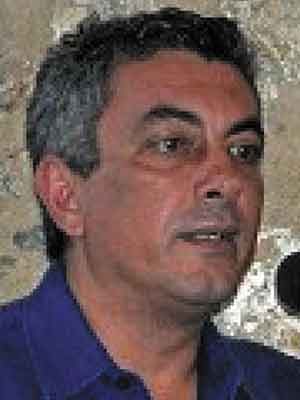
Apostolos Sarris
Dr Apostolos Sarris holds a B.A. n Astronomy & Physics (1985) and a M.A. in Physics (1988) from Boston University and a M.Sc. (1990) and a Ph.D. in Physics/Geophysics (1992) from the University of Nebraska-Lincoln. He is Professor of the “Sylvia Ioannou” Chair on Digital Humanities at the Archaeological Research Unit of Department of History and Archaeology at the University of Cyprus and Research Director at F.O.R.T.H. - Head of the GeoSat ReSeArch Lab. He is also an Adjunct/Affiliate Professor of Cyprus University of Technology and a Research Associate of the Dept. of Anthropology, The Field Museum of Natural History of Chicago, Illinois, USA. He has been a Senior MC Fellow of the EU at FRIAS/Freiburg University, a DLR-DAAD Research Fellow and a visiting senior researcher at the University of Leiden. He is currently Treasurer of UISPP Commission IV and associate editor of the Archaeological Prospection Journal. He has been involved in more than 110 large scale national, European and International large scale projects and in 240 programs of applied research in geophysics and remote sensing at Greece, Cyprus, Italy, Albania, Egypt, USA, Germany, Turkey, etc.
References
- Accardo, G., Giani, E., & Giovagnoli, A. (2003). The risk map of Italian cultural heritage. Journal of Architectural Conservation, 9(2), 41–57.
- Agapiou, A., Alexakis, D. D., Lysandrou, V., Sarris, A., Cuca, B., Themistocleous, K., & Hadjimitsis, D. G. (2015). Impact of urban sprawl to cultural heritage monuments: the case study of Paphos area in Cyprus. Journal of Cultural Heritage, 16(5), 671–680.
- Agapiou, A., Lysandrou, V., & Hadjimitsis, D. G. (2017). Optical remote sensing potentials for looting detection. Geosciences, 7(4), 98.
- Agapiou, A., Lysandrou, V., & Hadjimitsis, D. G. (2020). A European-scale investigation of soil erosion threat to subsurface archaeological remains. Remote Sensing, 12(4), 675.
- Agapiou, A., Lysandrou, V., Themistocleous, K., & Hadjimitsis, D. G. (2016). Risk assessment of cultural heritage sites clusters using satellite imagery and GIS: The case study of Paphos District, Cyprus. Natural Hazards, 83(S1), S5–S20.
- Alexakis, D., & Sarris, A. (2010). Environmental and Human Risk Assessment of the Prehistoric and Historic Archaeological Sites of Western Crete (Greece) with the Use of GIS, Remote Sensing, Fuzzy Logic and Neural Networks. In M. Ioannides, D. Fellner, A. Georgopoulos, & D. G. Hadjimitsis (Eds. DOI: 10.1007/978-3-642-16873-4_25), EuroMed 2010: Digital Heritage. Lecture Notes in Computer Science (Vol. 6436, pp. 332–342). Berlin, Heidelberg: Springer.
- Argyriou, A. V., Teeuw, R. M., & Sarris, A. (2017). GIS-based landform classification of bronze age archaeological sites on Crete Island. PLoS ONE, 12(2), e0170727.
- Awange, J., & Kiema, J. (2019). Fundamentals of GIS. In Environmental Geoinformatics – Extreme Hydro-Climatic and Food Security Challenges: Exploiting the Big Data. “Environmental Science and Engineering” book series (pp. 203–212). Cham, Switzerland: Springer.
- Barmaki, M., Pazira, E., & Hedayat, N. (2011). Investigation of relationships among the environmental factors and water erosion changes using EPM model and GIS. International Research Journal of Applied and Basic Sciences, 3(5), 945–949.
- Campbell, J. B., & Wynne, R. H. (2011). Introduction to Remote Sensing (5th edition ed., pp. 3–30). New York, USA: The Guilford Press.
- Chander, G., Markham, B. L., & Helder, D. L. (2009). Summary of current radiometric calibration coefficients for landsat MSS, TM, ETM+, and EO-1 ALI sensors. Remote Sensing of Environment, 113(5), 893–903.
- Choi, K., Arnhold, S., Huwe, B., & Reineking, B. (2017). Daily based morgan–morgan–finney (DMMF) model: A spatially distributed conceptual soil erosion model to simulate complex soil surface configurations. Water, 9(4), 278.
- Cuca, B. (2020). Copernicus sentinel imagery for more risk-resilient historic cities in coastal zones: Contribution to the monitoring of albenga archaeological site and delta of river Centa. Applied Geomatics. doi:10.1007/s12518-020-00340-5
- Cuca, B., & Agapiou, A. (2018). Impact of land-use change and soil erosion on cultural landscapes: The case of cultural paths and sites in Paphos district, Cyprus. Applied Geomatics, 10(4), 515–527.
- Della Torre, S. (2020). Italian perspective on the planned preventive conservation of architectural heritage. Frontiers of Architectural Research. doi:10.1016/j.foar.2020.07.008
- Elaloui, A., Marrakchi, C., Fekri, A., Maimouni, S., & Aradi, M. (2017). USLE-based assessment of soil erosion by water in the watershed upstream Tessaoute (Central High Atlas, Morocco). Modeling Earth Systems and Environment, 3(3), 873–885.
- ELSTAT – Hellenic Statistical Authority. (2011). Population and housing census: resident population. Retrieved from https://www.statistics.gr/el/statistics/pop
- Garcia Rodriguez, J. L., & Gimenez Suarez, M. C. (2012). Methodology for estimating the topographic factor LS of RUSLE3D and USPED using GIS. Geomorphology, 175–176, 98–106.
- Gelagay, H. S., & Minale, A. S. (2016). Soil loss estimation using GIS and Remote sensing techniques: A case of Koga watershed, Northwestern Ethiopia. International Soil and Water Conservation Research, 4(2), 126–136.
- Giardino, J. M. (2011). A history of NASA remote sensing contributions to archaeology. Journal of Archaeological Science, 38(9), 2003–2009.
- Glowacki, K. T., & Vogeikoff-Brogan, N. (2011). STEGA: The Archaeology of Houses and Households in Ancient Crete (Hesperia Supplement) (pp. 520). Princeton, New Jersey, USA: The American School of Classical Studies at Athens.
- Grillakis, M. G., Polykretis, C., & Alexakis, D. D. (2020). Past and projected climate change impacts on rainfall erosivity: advancing our knowledge for the eastern Mediterranean island of Crete. Catena, 193, 104625.
- Halecki, W., Kruk, E., & Ryczek, M. (2018). Evaluation of water erosion at a mountain catchment in Poland using the G2 model. Catena, 164, 116–124.
- Hapciuc, O. E., Romanescu, G., Minea, I., Iosub, M., Enea, A., & Sandu, I. (2016). Flood susceptibility analysis of the cultural heritage in the sucevita catchment (Romania). International Journal of Conservation Science, 7(2), 501–510.
- Karydas, C. G., & Panagos, P. (2018). The G2 erosion model: an algorithm for month-time step assessments. Environmental Research, 161, 256–267.
- Kincey, M., Gerrard, C., & Warburton, J. (2017). Quantifying erosion of ‘at risk’ archaeological sites using repeat terrestrial laser scanning. Journal of Archaeological Science: Reports, 12, 405–424.
- Kourgialas, N. N., Koubouris, G. C., Karatzas, G. P., & Metzidakis, I. (2016). Assessing water erosion in Mediterranean tree crops using GIS techniques and field measurements: the effect of climate change. Natural Hazards, 83(S1), S65–S81.
- Lasaponara, R., Leucci, G., Masini, N., & Persico, R. (2014). Investigating archaeological looting using satellite images and GEORADAR: The experience in lambayeque in North Peru. Journal of Archaeological Science, 42, 216–230.
- Leh, M., Bajwa, S., & Chaubey, I. (2013). Impact of land use change on erosion risk: An integrated remote sensing, geographic information system and modeling methodology. Land Degradation & Development, 24, 409–421.
- Liu, J., Xu, Z., Chen, F., Chen, F., & Zhang, L. (2019). Flood hazard mapping and assessment on the Angkor World Heritage Site, Cambodia. Remote Sensing, 11(1), 98.
- Lombardo, L., Tanyas, H., & Nicu, I. C. (2020). Spatial modeling of multi-hazard threat to cultural heritage sites. Engineering Geology, 277, 105776.
- Lysandrou, V., & Agapiou, A. (2016). Cities of the dead: Approaching the landscape of hellenistic and roman necropoleis of Cyprus. Archaeological and Anthropological Sciences, 8(4), 867–877.
- Maury, S., Gholkar, M., Jadhav, A., & Rane, N. (2019). Geophysical evaluation of soils and soil loss estimation in a semiarid region of Maharashtra using revised universal soil loss equation (RUSLE) and GIS methods. Environmental Earth Sciences, 78(5), 144.
- Mitasova, H., Hofierka, J., Zlocha, M., & Iverson, L. R. (1996). Modeling topographic potential for erosion and deposition using GIS. International Journal of GIS, 10, 629–641.
- Moore, I. D., & Burch, G. J. (1986). Modeling erosion and deposition: Topographic effects. Transactions of the ASAE, 29(6), 1624–1630.
- Morgan, R. P. C. (2005). Soil Erosion and Conservation (pp. 304). Oxford, UK: Blackwell Publishing.
- Negula, I. D., Sofronie, R., Virsta, A., & Badea, A. (2015). Earth observation for the world cultural and natural heritage. Agriculture and Agricultural Science Procedia, 6, 438–445.
- Oppio, A., Bottero, M., Ferretti, V., Fratesi, U., Ponzini, D., & Pracchi, V. (2015). Giving space to multicriteria analysis for complex cultural heritage systems: The case of the castles in Valle D’Aosta Region, Italy. Journal of Cultural Heritage, 16(6), 779–789.
- Ortiz, R., Ortiz, P., Martín, J. M., & Vázquez, M. A. (2016). A new approach to the assessment of flooding and dampness hazards in cultural heritage, applied to the historic centre of Seville (Spain). Science of the Total Environment, 551–552, 546–555.
- Pal, S. C., & Chakrabortty, R. (2019). Simulating the impact of climate change on soil erosion in sub-tropical monsoon dominated watershed based on RUSLE, SCS runoff and MIROC5 climatic model. Advances in Space Research, 64(2), 352–377.
- Panagos, P., Borrelli, P., Meusburger, K., Van Der Zanden, E. H., Poesen, J., & Alewell, C. (2015b). Modelling the effect of support practices (P-factor) on the reduction of soil erosion by water at European Scale. Environmental Science & Policy, 51, 23–34.
- Panagos, P., Borrelli, P., Poesen, J., Ballabio, C., Lugato, E., Meusburger, K., … Alewell, C. (2015a). The new assessment of soil loss by water erosion in Europe. Environmental Science & Policy, 54, 438–447.
- Pandey, A., Himanshu, S. K., Mishra, S. K., & Singh, V. P. (2016). Physically based soil erosion and sediment yield models revisited. Catena, 147, 595–620.
- Panou, C., Ragia, L., Dimelli, D., & Mania, K. (2018). An architecture for mobile outdoors augmented reality for cultural heritage. ISPRS International Journal of Geo-Information, 7(12), 463.
- Pastonchi, L., Barra, A., Monserrat, O., Luzi, G., Solari, L., & Tofani, V. (2018). Satellite data to improve the knowledge of geohazards in world heritage sites. Remote Sensing, 10(7), 992.
- Phinzi, K., & Ngetar, N. S. (2019). The assessment of water-borne erosion at catchment level using GIS-based RUSLE and remote sensing: A review. International Soil and Water Conservation Research, 7(1), 27–46.
- Polykretis, C., Alexakis, D. D., Grillakis, M. G., & Manoudakis, S. (2020). Assessment of intra-annual and inter-annual variabilities of soil erosion in Crete Island (Greece) by incorporating the dynamic “Nature” of R and C-factors in RUSLE modeling. Remote Sensing, 12(15), 2439.
- Ravankhah, M., De Wit, R., Argyriou, A. V., Chliaoutakis, A., Revez, M. J., Birkmann, J., … Giapitsoglou, K. (2019). Integrated assessment of natural hazards, including climate change’s influences, for cultural heritage sites: the case of the historic centre of rethymno in Greece. International Journal of Disaster Risk Science, 10(3), 343–361.
- Salamon, A., Netzer-Cohen, C., Cohen, M., & Zilberman, E. (2018). Preliminary methodology for qualitative assessment of earthquake hazards to historical monuments in Israel. International Journal of Disaster Risk Reduction, 31, 1062–1081.
- Sarris, A., Peraki, E., Chatzoyiannaki, N., Elvanidou, M., Kappa, E., Kakoulaki, G., … Athanasaki, K. (2009). Time drilling through the past of the Island of Crete. In A. Velho & H. Kamermans (eds), Technology and Methodology for Archaeological Practice: Practical applications for the past reconstruction/Technologie et Méthodologie pour la pratique en Archéologie: Applications pratiques pour la reconstruction du passé. Proceedings of the XV UISPP World Congress (Lisbon, 4-9 September 2006)/Actes du XV Congrès Mondial (Lisbonne, 4-9 Septembre 2006), Vol. 37, pp. 115–123.
- Song, C., Woodcock, C. E., Seto, K. C., Lenney, M. P., & Macomber, S. A. (2001). Classification and change detection using landsat TM data: when and how to correct atmospheric effects? Remote Sensing of Environment, 75(2), 230–244.
- Spennemann, D. H. R. (1999). Cultural heritage conservation during emergency management: Luxury or necessity? International Journal of Public Administration, 2(5), 745–804.
- Srivastava, N., & Rao, S. (2016). Learning-based text classifiers using the mahalanobis distance for correlated datasets. International Journal of Big Data Intelligence, 3(1), 1–9.
- Sujatha, E. R., & Sridhar, V. (2018). Spatial prediction of erosion risk of a small mountainous watershed using RUSLE: A case-study of the palar sub-watershed in Kodaikanal, South India. Water, 10(11), 1608.
- Tsanis, I. K., Koutroulis, A. G., Daliakopoulos, I. N., & Jacod, D. (2011). Severe climate-induced water shortage and extremes in Crete. Climatic Change, 106(4), 667–677.
- UNESCO World Heritage Centre. (2017). The Operational Guidelines for the Implementation of the World Heritage Convention. Retrieved from https://whc.unesco.org/en/guidelines/
- Valagussa, A., Frattini, P., Crosta, G., Spizzichino, D., Leoni, G., & Margottini, C. (2020). Multi-risk analysis on European cultural and natural UNESCO heritage sites. Natural Hazards. doi:10.1007/s11069-020-04417-7
- Williams, R. J., & Renard, K. G. (1983). EPIC—A new method for assessing erosion’s effect on soil productivity. Journal of Soil and Water Conservation, 38, 381–383.